Abstract
Possible methods by which eukaryotic cells can regulate intracellular pH (pHi) in response to experimental acid loading were investigated by using as a model cell the fungus Neurospora. Attention was focused on the role of membrane transport in such regulation, starting from the fact that this organism possesses a powerful electrogenic proton extrusion pump. Intracellular acidification was forced by introducing butyric acid into the recording medium, and subsequent changes in pHi and membrane potential were determined with intracellular microelectrodes. In separate experiments, membrane current-voltage curves were obtained and resolved--by an explicit kinetic model--into distinct pump and leak components. Decreased pHi causes increased outward pumping of H+ ions, in a manner quantitatively consistent with their role as a substrate for the proton pump. This increased pumping is often manifest as a transient hyperpolarization at the onset of cytoplasmic acidification. With a considerably slower time course, decreased pHi also produces a large increase in membrane leak conductance, which brings about net membrane depolarization and further stimulates the pump (by virtue of the reduced back electromotive force). Although the identity of the ion responsible for increased leak conductance is not yet known, the evident modulation of conductance seemingly plays an important role in stabilizing the intracellular pH: Stimulation of the pump alone would have little net effect on pHi because it would result simply in enhanced backflux of H+ (to which the membrane is most permeable in normal circumstances). An increased leak to nonprotons, however, would allow the pump to accomplish net H+ ejection.
Full text
PDF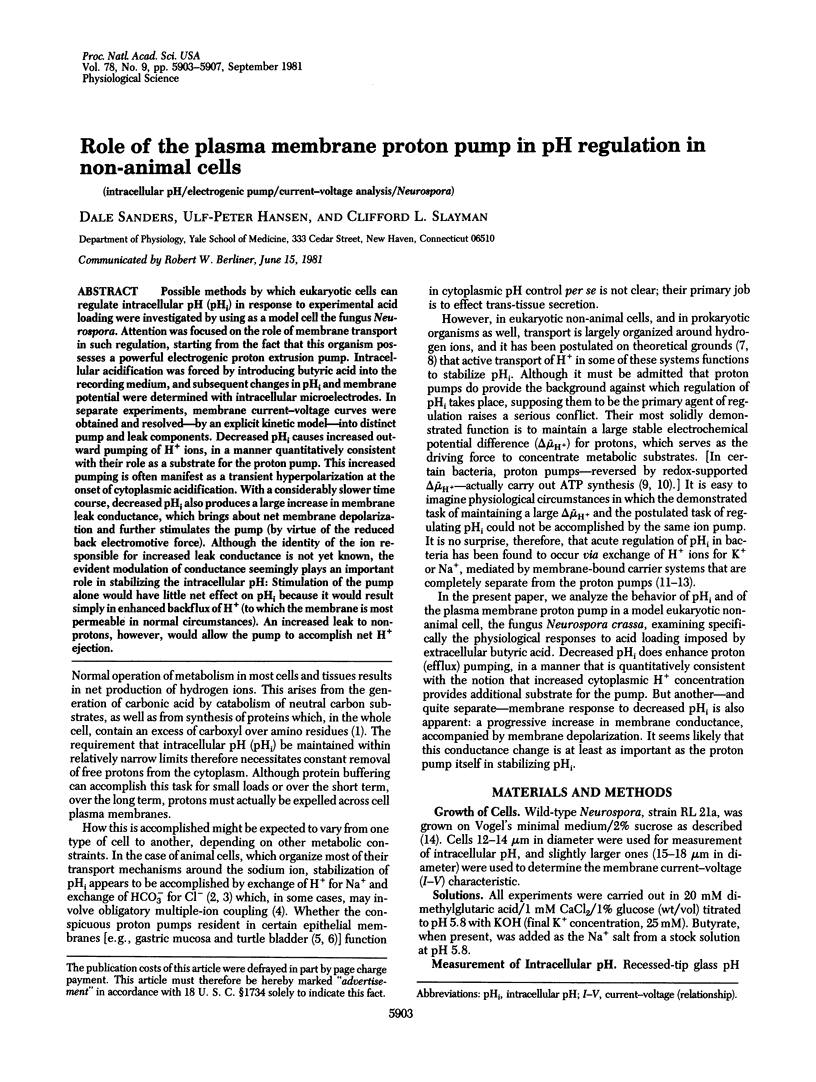
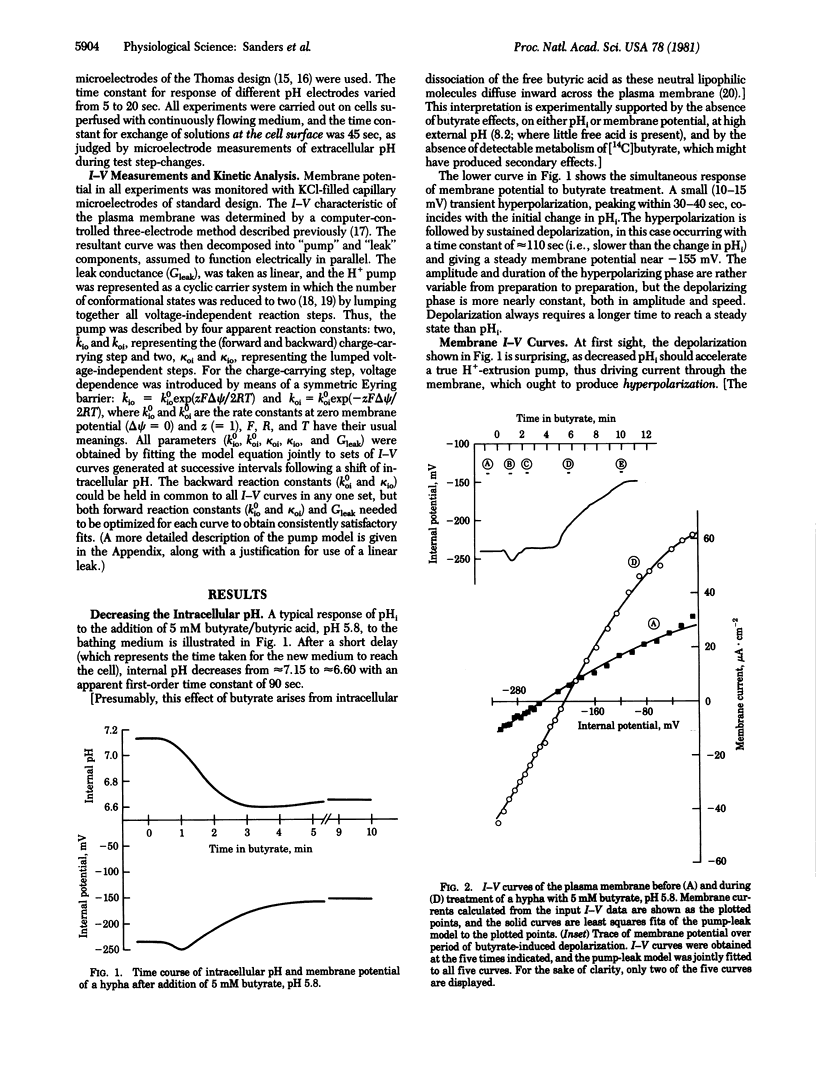
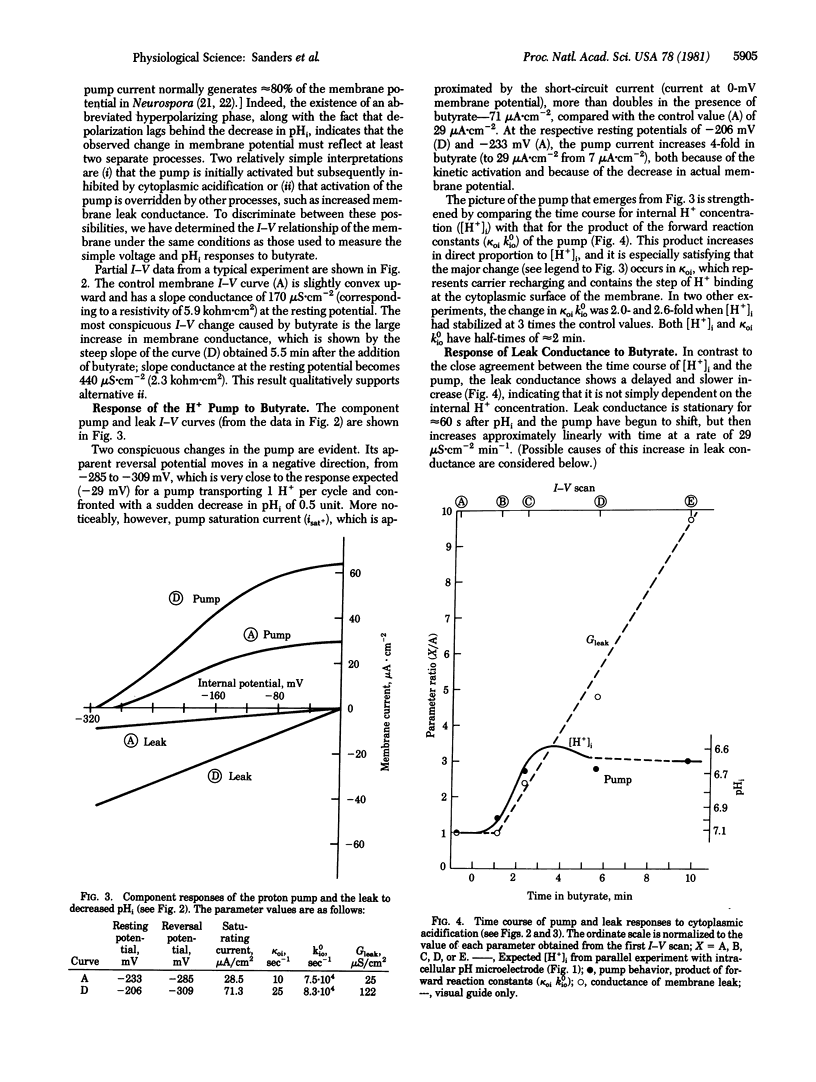
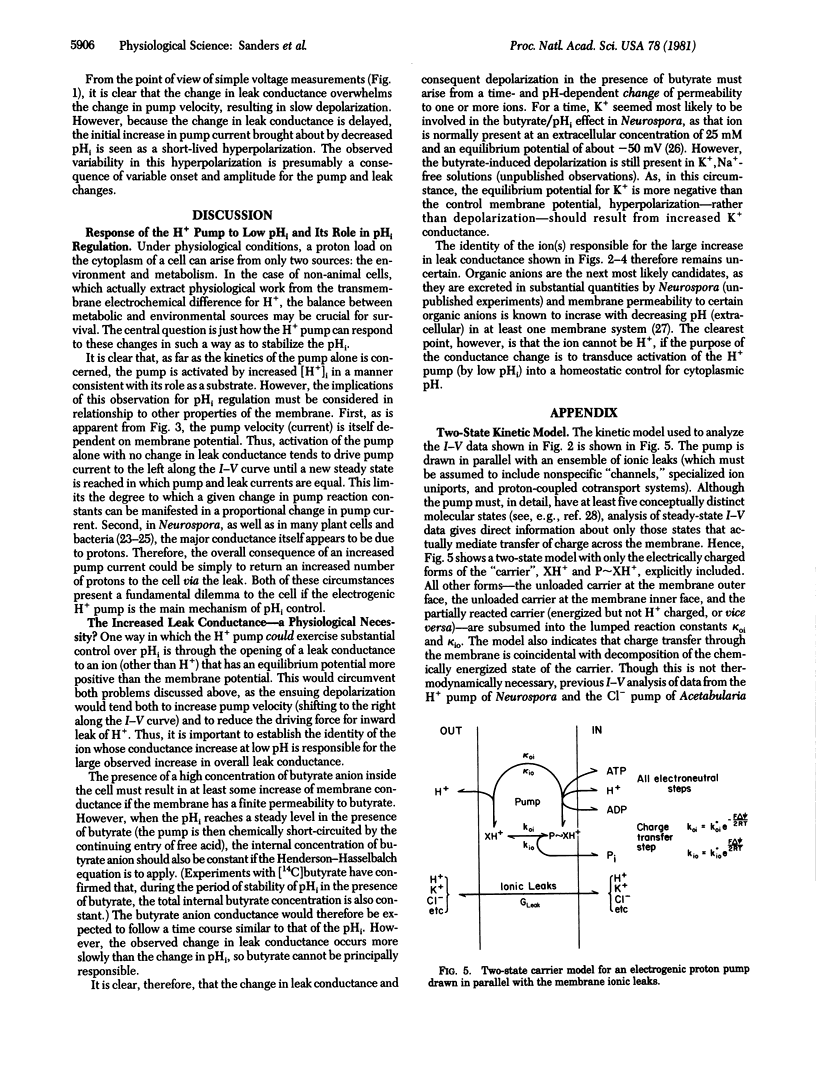
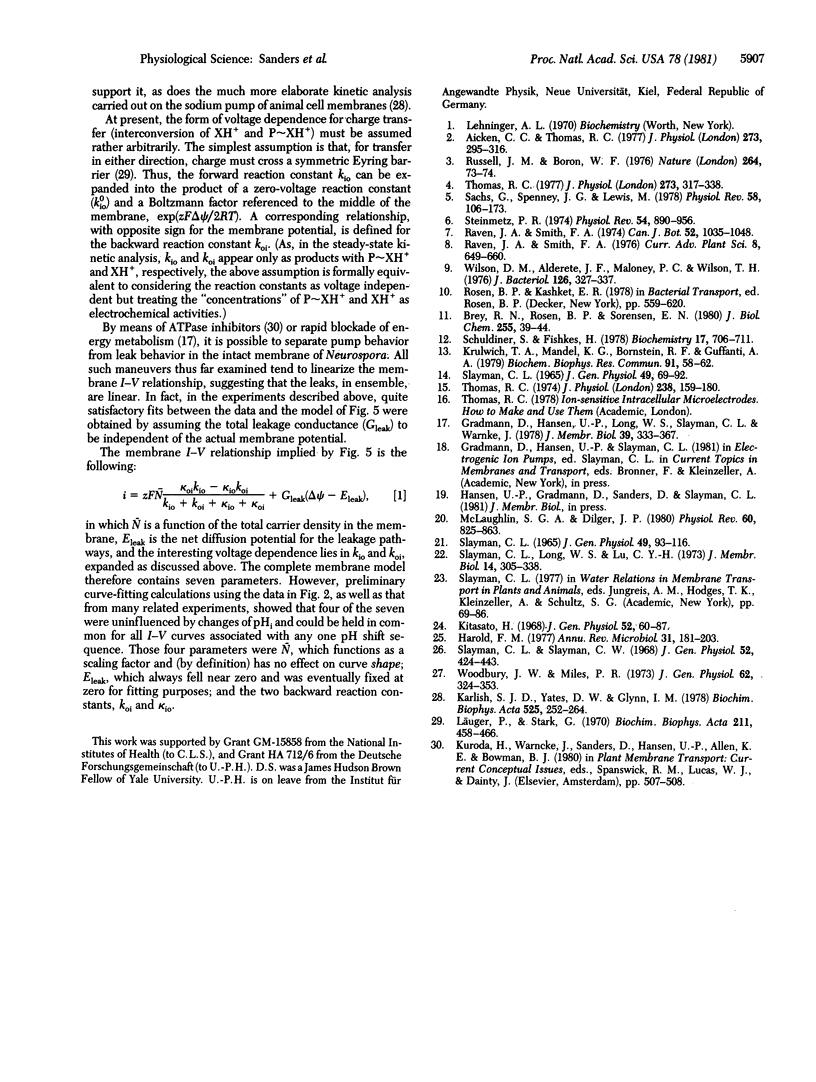
Selected References
These references are in PubMed. This may not be the complete list of references from this article.
- Aickin C. C., Thomas R. C. An investigation of the ionic mechanism of intracellular pH regulation in mouse soleus muscle fibres. J Physiol. 1977 Dec;273(1):295–316. doi: 10.1113/jphysiol.1977.sp012095. [DOI] [PMC free article] [PubMed] [Google Scholar]
- Brey R. N., Rosen B. P., Sorensen E. N. Cation/proton antiport systems in Escherichia coli. Properties of the potassium/proton antiporter. J Biol Chem. 1980 Jan 10;255(1):39–44. [PubMed] [Google Scholar]
- Gradmann D., Hansen U. P., Long W. S., Slayman C. L., Warncke J. Current-voltage relationships for the plasma membrane and its principal electrogenic pump in Neurospora crassa: I. Steady-state conditions. J Membr Biol. 1978 Mar 20;39(4):333–367. doi: 10.1007/BF01869898. [DOI] [PubMed] [Google Scholar]
- Harold F. M. Ion currents and physiological functions in microorganisms. Annu Rev Microbiol. 1977;31:181–203. doi: 10.1146/annurev.mi.31.100177.001145. [DOI] [PubMed] [Google Scholar]
- Karlish S. J., Yates D. W., Glynn I. M. Conformational transitions between Na+-bound and K+-bound forms of (Na+ + K+)-ATPase, studied with formycin nucleotides. Biochim Biophys Acta. 1978 Jul 7;525(1):252–264. doi: 10.1016/0005-2744(78)90219-x. [DOI] [PubMed] [Google Scholar]
- Kitasato H. The influence of H+ on the membrane potential and ion fluxes of Nitella. J Gen Physiol. 1968 Jul;52(1):60–87. doi: 10.1085/jgp.52.1.60. [DOI] [PMC free article] [PubMed] [Google Scholar]
- Krulwich T. A., Mandel K. G., Bornstein R. F., Guffanti A. A. A non-alkalophilic mutant of Bacillus alcalophilus lacks the Na+/H+ antiporter. Biochem Biophys Res Commun. 1979 Nov 14;91(1):58–62. doi: 10.1016/0006-291x(79)90582-5. [DOI] [PubMed] [Google Scholar]
- Läuger P., Stark G. Kinetics of carrier-mediated ion transport across lipid bilayer membranes. Biochim Biophys Acta. 1970 Sep 15;211(3):458–466. doi: 10.1016/0005-2736(70)90251-8. [DOI] [PubMed] [Google Scholar]
- McLaughlin S. G., Dilger J. P. Transport of protons across membranes by weak acids. Physiol Rev. 1980 Jul;60(3):825–863. doi: 10.1152/physrev.1980.60.3.825. [DOI] [PubMed] [Google Scholar]
- Russell J. M., Boron W. F. Role of choloride transport in regulation of intracellular pH. Nature. 1976 Nov 4;264(5581):73–74. doi: 10.1038/264073a0. [DOI] [PubMed] [Google Scholar]
- Sachs G., Spenney J. G., Lewin M. H+ transport: regulation and mechanism in gastric mucosa and membrane vesicles. Physiol Rev. 1978 Jan;58(1):106–173. doi: 10.1152/physrev.1978.58.1.106. [DOI] [PubMed] [Google Scholar]
- Schuldiner S., Fishkes H. Sodium-proton antiport in isolated membrane vesicles of Escherichia coli. Biochemistry. 1978 Feb 21;17(4):706–711. doi: 10.1021/bi00597a023. [DOI] [PubMed] [Google Scholar]
- Slayman C. L. Electrical properties of Neurospora crassa. Effects of external cations on the intracellular potential. J Gen Physiol. 1965 Sep;49(1):69–92. doi: 10.1085/jgp.49.1.69. [DOI] [PMC free article] [PubMed] [Google Scholar]
- Slayman C. L. Electrical properties of Neurospora crassa. Respiration and the intracellular potential. J Gen Physiol. 1965 Sep;49(1):93–116. doi: 10.1085/jgp.49.1.93. [DOI] [PMC free article] [PubMed] [Google Scholar]
- Slayman C. L., Long W. S., Lu C. Y. The relationship between ATP and an electrogenic pump in the plasma membrane of Neurospora crassa. J Membr Biol. 1973;14(4):305–338. doi: 10.1007/BF01868083. [DOI] [PubMed] [Google Scholar]
- Slayman C. L., Slayman C. W. Net uptake of potassium in Neurospora. Exchange for sodium and hydrogen ions. J Gen Physiol. 1968 Sep;52(3):424–443. doi: 10.1085/jgp.52.3.424. [DOI] [PMC free article] [PubMed] [Google Scholar]
- Steinmetz P. R. Cellular mechanisms of urinary acidification. Physiol Rev. 1974 Oct;54(4):890–956. doi: 10.1152/physrev.1974.54.4.890. [DOI] [PubMed] [Google Scholar]
- Thomas R. C. Intracellular pH of snail neurones measured with a new pH-sensitive glass mirco-electrode. J Physiol. 1974 Apr;238(1):159–180. doi: 10.1113/jphysiol.1974.sp010516. [DOI] [PMC free article] [PubMed] [Google Scholar]
- Thomas R. C. The role of bicarbonate, chloride and sodium ions in the regulation of intracellular pH in snail neurones. J Physiol. 1977 Dec;273(1):317–338. doi: 10.1113/jphysiol.1977.sp012096. [DOI] [PMC free article] [PubMed] [Google Scholar]
- Wilson D. M., Alderette J. F., Maloney P. C., Wilson T. H. Protonmotive force as the source of energy for adenosine 5'-triphosphate synthesis in Escherichia coli. J Bacteriol. 1976 Apr;126(1):327–337. doi: 10.1128/jb.126.1.327-337.1976. [DOI] [PMC free article] [PubMed] [Google Scholar]
- Woodbury J. W., Miles P. R. Anion conductance of frog muscle membranes: one channel, two kinds of pH dependence. J Gen Physiol. 1973 Sep;62(3):324–353. doi: 10.1085/jgp.62.3.324. [DOI] [PMC free article] [PubMed] [Google Scholar]