Abstract
Various methods to incorporate bacteriorhodopsin in black lipid membranes are reported. Both purple membrane patches and monomeric bacteriorhodopsin were used as starting material. The incorporation of bacteriorhodopsin into planar lipid bilayers was achieved by the following methods. (i) Purple membrane patches were transferred from water to solutions of lipids in n-alkanes. Black membranes were formed from such organic suspensions. (ii) Lipid layers containing solvent and purple membranes were spread on an air/water interface. These layers were used to form planar bilayers. (iii) Vesicles containing purple membranes or monomeric bacteriorhodopsin were spread on an air/water interface and, from the resulting layer, bilayers were formed. On illumination, steady-state photocurrents were observed in all three cases, indicating that these methods lead to functional transmembranous integration of the protein in the planar black lipid membrane. The influence of an applied electric field on the pumping process was studied on membranes formed by using method i. At approximately 200 mV, the photocurrent tends to zero. Furthermore, it was possible to make planar lipid bilayers photoelectrically active by adding vesicles containing monomeric bacteriorhodopsin to the bathing solution. Because, in this case, only transient photocurrents were observed, it can be concluded that the vesicles are attached to but not fused with the black lipid membrane.
Full text
PDF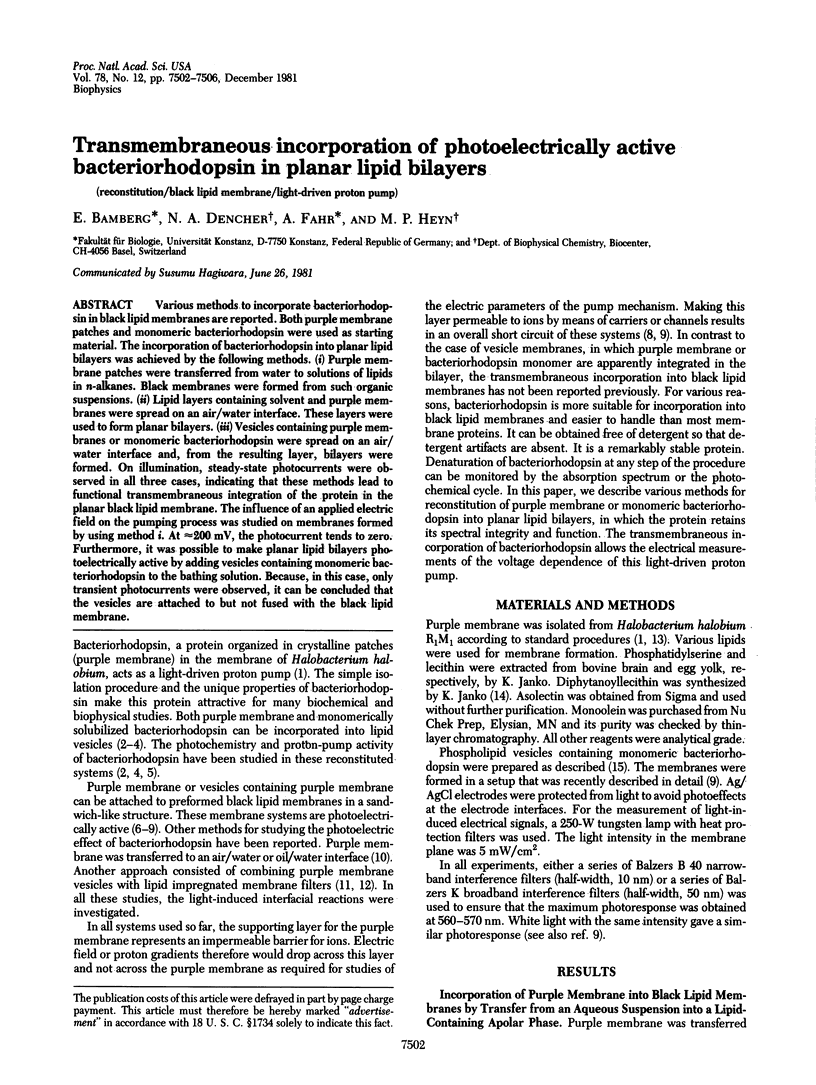
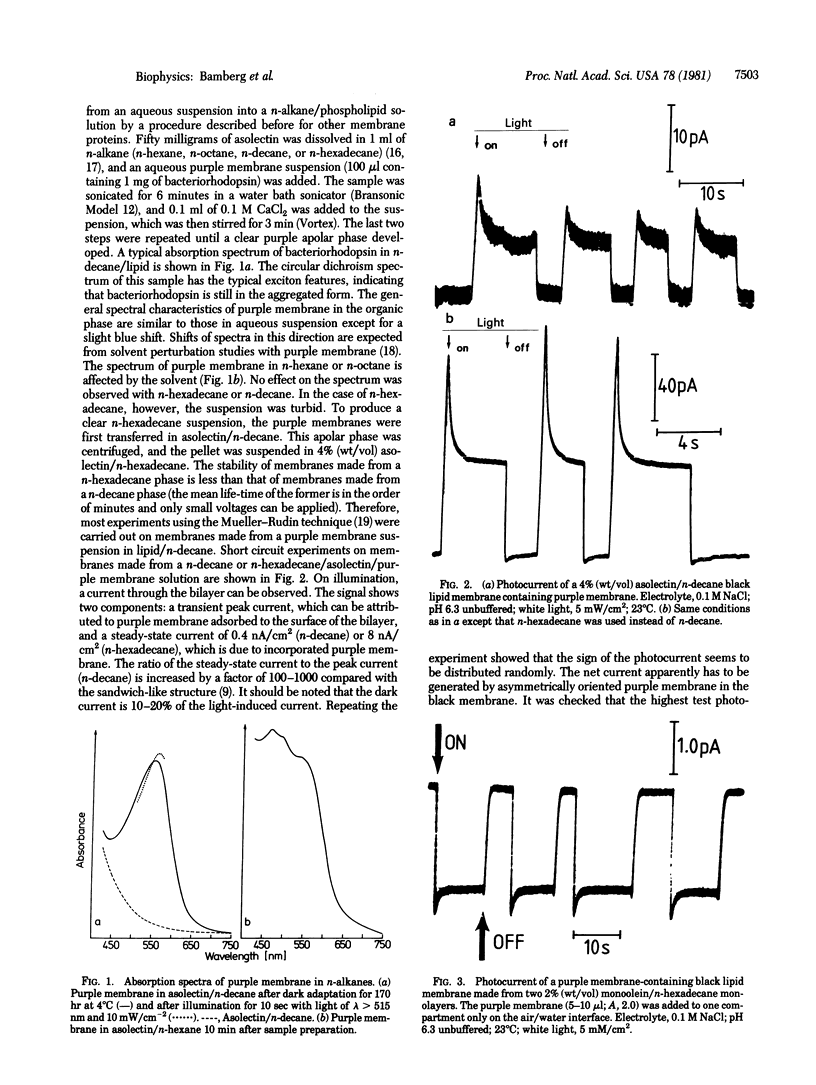
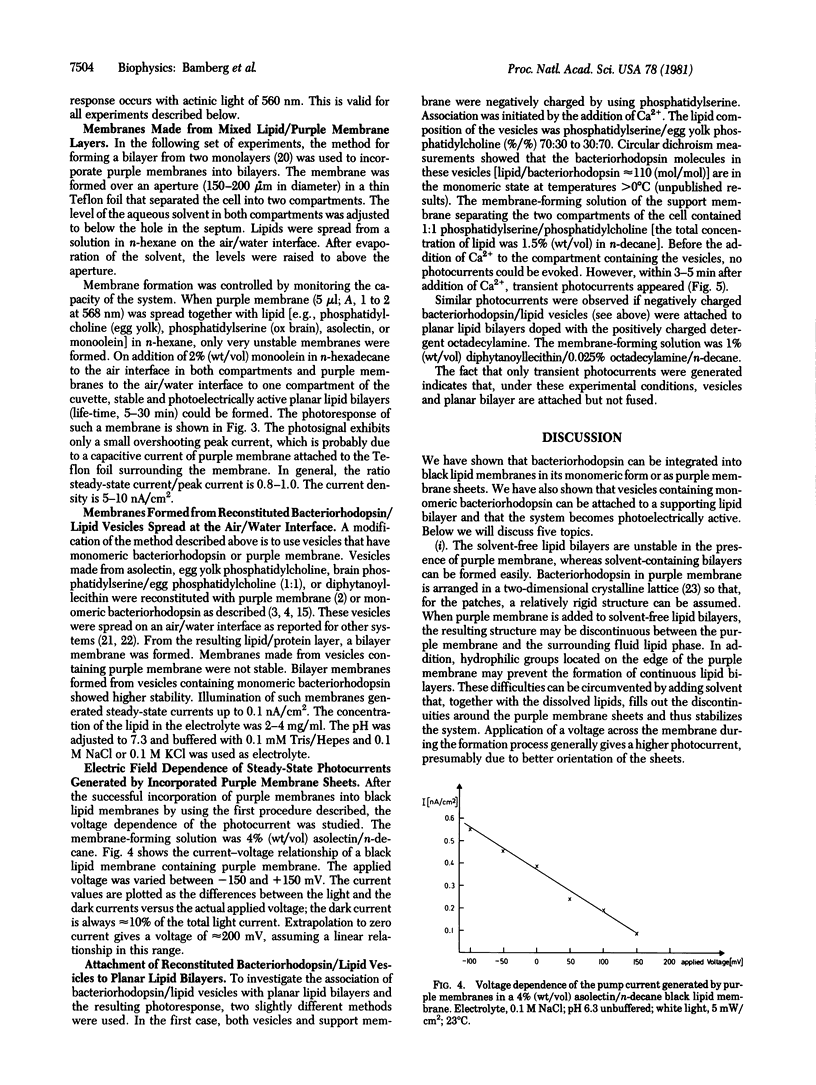
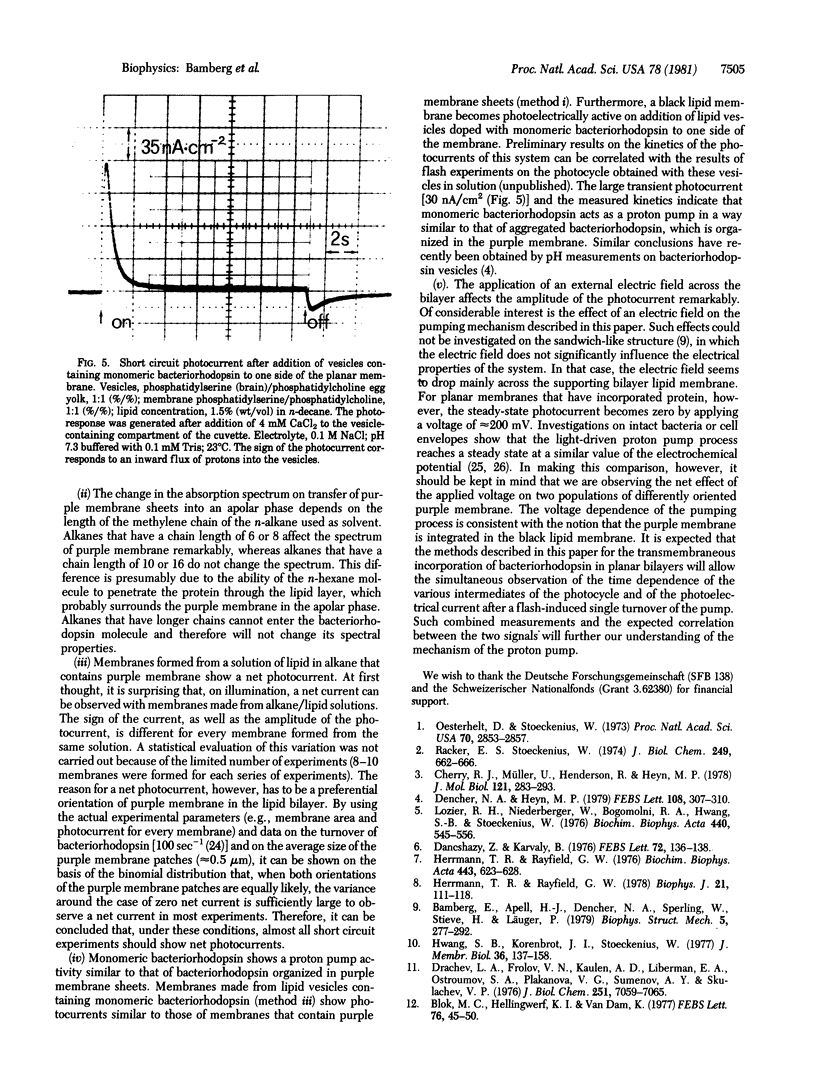
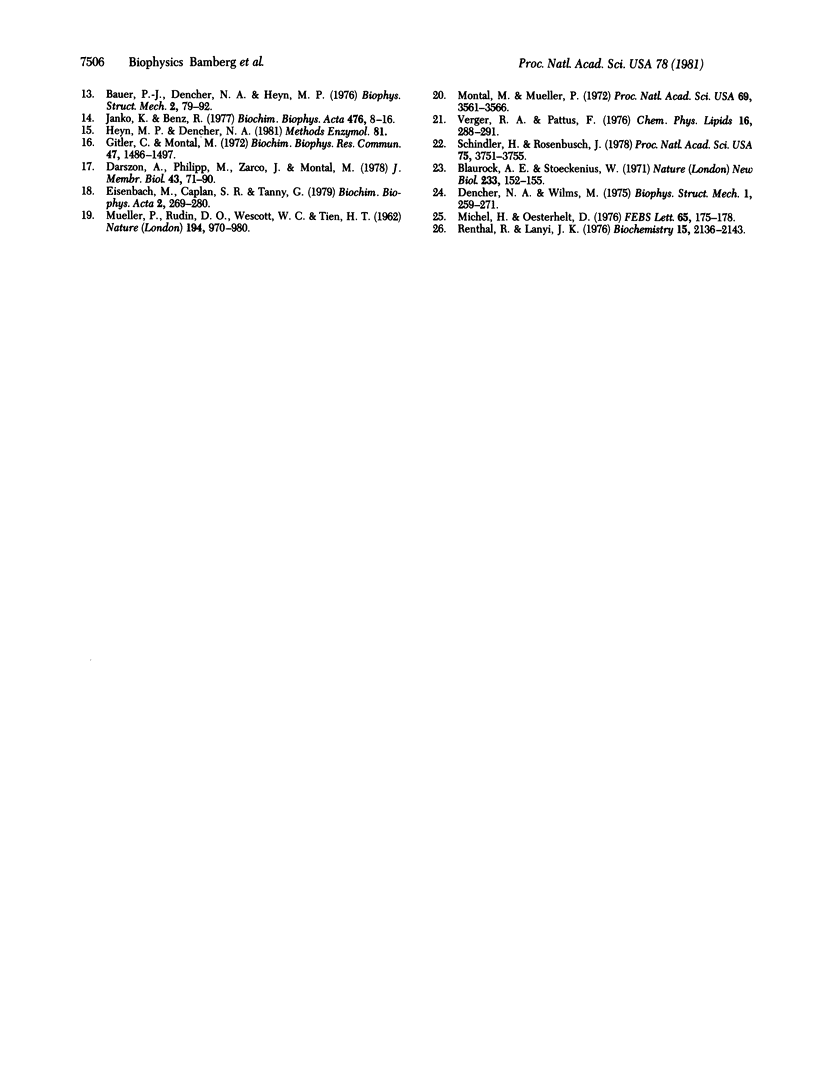
Images in this article
Selected References
These references are in PubMed. This may not be the complete list of references from this article.
- Bauer P. J., Dencher N. A., Heyn M. P. Evidence for chromophore-chromophore interactions in the purple membrane from reconstitution experiments of the chromophore-free membrane. Biophys Struct Mech. 1976 Apr 15;2(1):79–92. doi: 10.1007/BF00535654. [DOI] [PubMed] [Google Scholar]
- Blaurock A. E., Stoeckenius W. Structure of the purple membrane. Nat New Biol. 1971 Sep 29;233(39):152–155. doi: 10.1038/newbio233152a0. [DOI] [PubMed] [Google Scholar]
- Blok M. C., Hellingwerf K. J., Van Dam K. Reconstitution of bacteriorhodopsin in a millipore filter system. FEBS Lett. 1977 Apr 1;76(1):45–50. doi: 10.1016/0014-5793(77)80117-8. [DOI] [PubMed] [Google Scholar]
- Dancsházy Z., Karvaly B. Incorporation of bacteriorhodopsin into a bilayer lipid membrane; a photoelectric-spectroscopic study. FEBS Lett. 1976 Dec 15;72(1):136–138. doi: 10.1016/0014-5793(76)80829-0. [DOI] [PubMed] [Google Scholar]
- Dencher N. A., Heyn M. P. Bacteriorhodopsin monomers pump protons. FEBS Lett. 1979 Dec 15;108(2):307–310. doi: 10.1016/0014-5793(79)80552-9. [DOI] [PubMed] [Google Scholar]
- Dencher N., Wilms M. Flash photometric experiments on the photochemical cycle of bacteriorhodopsin. Biophys Struct Mech. 1975 May 30;1(3):259–271. doi: 10.1007/BF00535760. [DOI] [PubMed] [Google Scholar]
- Drachev L. A., Frolov V. N., Kaulen A. D., Liberman E. A., Ostroumov S. A., Plakunova V. G., Semenov A. Y., Skulachev V. P. Reconstitution of Biological Molecular generators of electric current. Bacteriorhodopsin. J Biol Chem. 1976 Nov 25;251(22):7059–7065. [PubMed] [Google Scholar]
- Eisenbach M., Caplan S. R., Tanny G. Interaction of purple membrane with solvents. I. Applicability of solubility parameter mapping. Biochim Biophys Acta. 1979 Jul 5;554(2):269–280. doi: 10.1016/0005-2736(79)90369-9. [DOI] [PubMed] [Google Scholar]
- Gitler C., Montal M. Thin-proteolipid films: a new approach to the reconstitution of biological membranes. Biochem Biophys Res Commun. 1972 Jun 28;47(6):1486–1491. doi: 10.1016/0006-291x(72)90240-9. [DOI] [PubMed] [Google Scholar]
- Herrmann T. R., Rayfield G. W. A measurement of the proton pump current generated by bacteriorhodopsin in black lipid membranes. Biochim Biophys Acta. 1976 Sep 7;443(3):623–628. doi: 10.1016/0005-2736(76)90482-x. [DOI] [PubMed] [Google Scholar]
- Herrmann T. R., Rayfield G. W. The electrical response to light of bacteriorhodopsin in planar membranes. Biophys J. 1978 Feb;21(2):111–125. doi: 10.1016/S0006-3495(78)85512-X. [DOI] [PMC free article] [PubMed] [Google Scholar]
- Hwang S. B., Korenbrot J. I., Stoeckenius W. Proton transport by bacteriorhodopsin through an interface film. J Membr Biol. 1977 Sep 14;36(2-3):137–158. doi: 10.1007/BF01868148. [DOI] [PubMed] [Google Scholar]
- Janko K., Benz R. Properties of lipid bilayer membranes made from lipids containing phytanic acid. Biochim Biophys Acta. 1977 Oct 3;470(1):8–16. doi: 10.1016/0005-2736(77)90057-8. [DOI] [PubMed] [Google Scholar]
- Lozier R. H., Niederberger W., Bogomolni R. A., Hwang S., Stoeckenius W. Kinetics and stoichiometry of light-induced proton release and uptake from purple membrane fragments, Halobacterium halobium cell envelopes, and phospholipid vesicles containing oriented purple membrane. Biochim Biophys Acta. 1976 Sep 13;440(3):545–556. doi: 10.1016/0005-2728(76)90041-4. [DOI] [PubMed] [Google Scholar]
- MUELLER P., RUDIN D. O., TIEN H. T., WESCOTT W. C. Reconstitution of cell membrane structure in vitro and its transformation into an excitable system. Nature. 1962 Jun 9;194:979–980. doi: 10.1038/194979a0. [DOI] [PubMed] [Google Scholar]
- Michel H., Oesterhelt D. Light-induced changes of the pH gradient and the membrane potential in H. halobium. FEBS Lett. 1976 Jun 1;65(2):175–178. doi: 10.1016/0014-5793(76)80473-5. [DOI] [PubMed] [Google Scholar]
- Montal M., Mueller P. Formation of bimolecular membranes from lipid monolayers and a study of their electrical properties. Proc Natl Acad Sci U S A. 1972 Dec;69(12):3561–3566. doi: 10.1073/pnas.69.12.3561. [DOI] [PMC free article] [PubMed] [Google Scholar]
- Oesterhelt D., Stoeckenius W. Functions of a new photoreceptor membrane. Proc Natl Acad Sci U S A. 1973 Oct;70(10):2853–2857. doi: 10.1073/pnas.70.10.2853. [DOI] [PMC free article] [PubMed] [Google Scholar]
- Renthal R., Lanyi J. K. Light-induced membrane potential and pH gradient in Halobacterium halobium envelope vesicles. Biochemistry. 1976 May 18;15(10):2136–2143. doi: 10.1021/bi00655a017. [DOI] [PubMed] [Google Scholar]
- Schindler H., Rosenbusch J. P. Matrix protein from Escherichia coli outer membranes forms voltage-controlled channels in lipid bilayers. Proc Natl Acad Sci U S A. 1978 Aug;75(8):3751–3755. doi: 10.1073/pnas.75.8.3751. [DOI] [PMC free article] [PubMed] [Google Scholar]
- Verger R., Pattus F. Spreading of membranes at the air/water interface. Chem Phys Lipids. 1976 Jul;16(4):285–291. doi: 10.1016/0009-3084(76)90023-2. [DOI] [PubMed] [Google Scholar]