Abstract
31P NMR spectra of Ehrlich ascites tumor cells, suspended in a physiological medium, show well-defined αP, βP, and γP resonances of intracellular ATP. A comparison of the separation of 360 ± 1 Hz between the resonances of αP and βP in intact cells with the corresponding separations of 349 ± 0.5 and 438 ± 1 Hz in noncellular MgATP and ATP standards, measured at 10°C and 40.5 MHz NMR frequency, reveals that 88% of the total intracellular ATP (3.3 mM) is complexed to Mg2+. The corresponding value for intracellular free Mg2+ is 0.44 μmol/ml out of a total Mg2+ content of 8.5 μmol/ml cell water. A similar value of free Mg2+ is obtained from a comparison of the separation of 560 ± 1 Hz between the βP and γP resonances of intracellular ATP with the corresponding values of 552 ± 0.5 and 621 ± 1 Hz in noncellular MgATP and ATP standards. The fraction of total cellular ATP complexed to Mg2+ decreased to 80% in tumor cells incubated in a medium containing adenosine, glucose, and Pi without Mg2+, the corresponding level of intracellular free Mg2+ being significantly lower (0.24 mM) than that in the unincubated cells. With Mg2+ present in the incubation medium, MgATP and total Mg2+ levels increased by ≈2.5 mM, whereas with no added Mg2+, the MgATP increased 1.6 mM apparently at the expense of other Mg2+-complexed constituents since total Mg2+ remained essentially unchanged. At the measured levels of free Mg2+, intracellular ADP will be only 35-50% complexed to Mg2+. A knowledge of the state of Mg2+ complexation of ATP and ADP in intact Ehrlich tumor cells allows an accurate estimation of the phosphorylation potential and the extent of deviation of the mass action ratio ([MgATP][AMP]f/[MgADP][ADP]f) in cells with high phosphorylation potential from the Mg2+-dependent equilibrium value of the adenylate kinase reaction. The significance of the free Mg2+ value measured by the noninvasive NMR method in relation to the existence of Mg2+ complexation and compartmentation in tumor cells is discussed.
Keywords: nucleotide metabolism, adenine nucleotides, bioenergetics, phosphoryl transfer, phosphorylation potential
Full text
PDF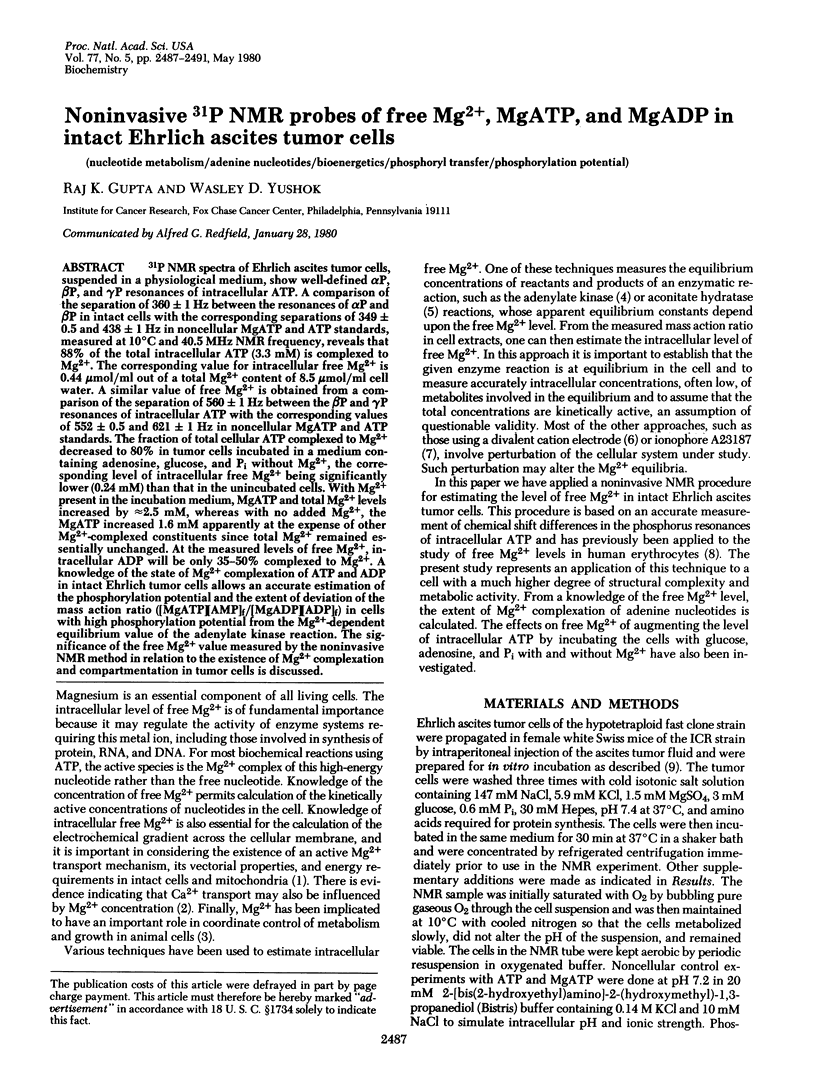
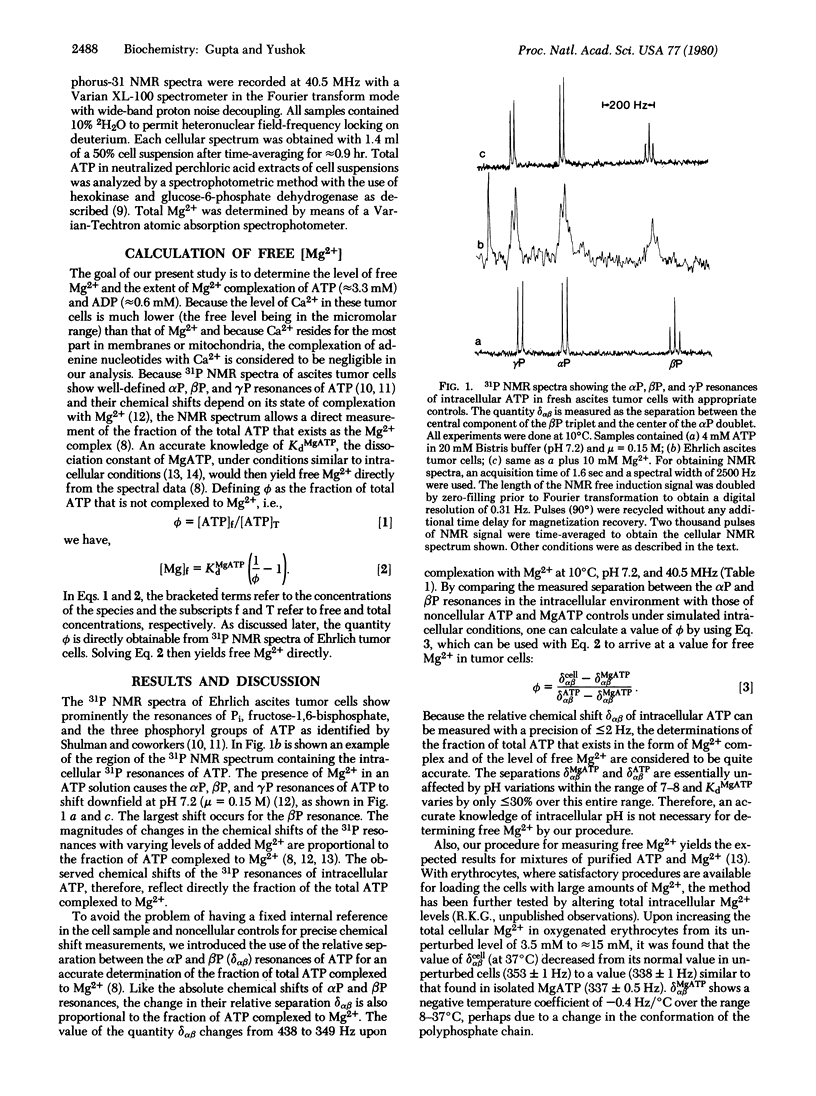
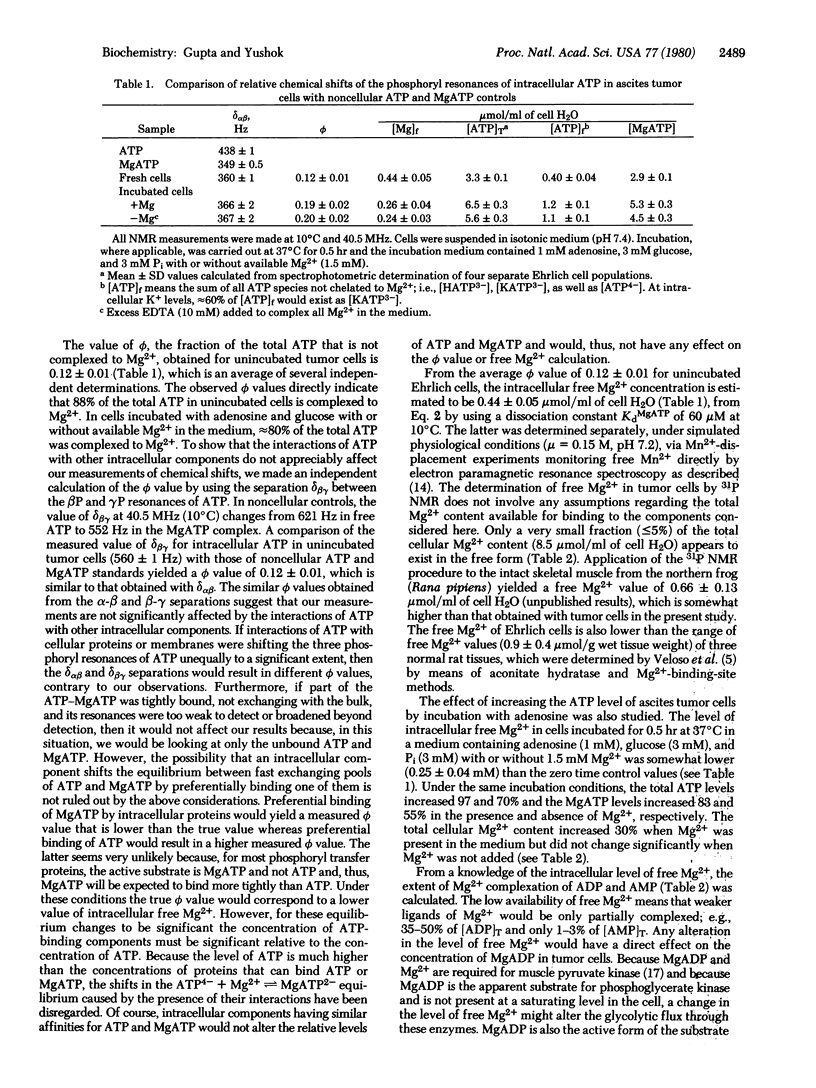
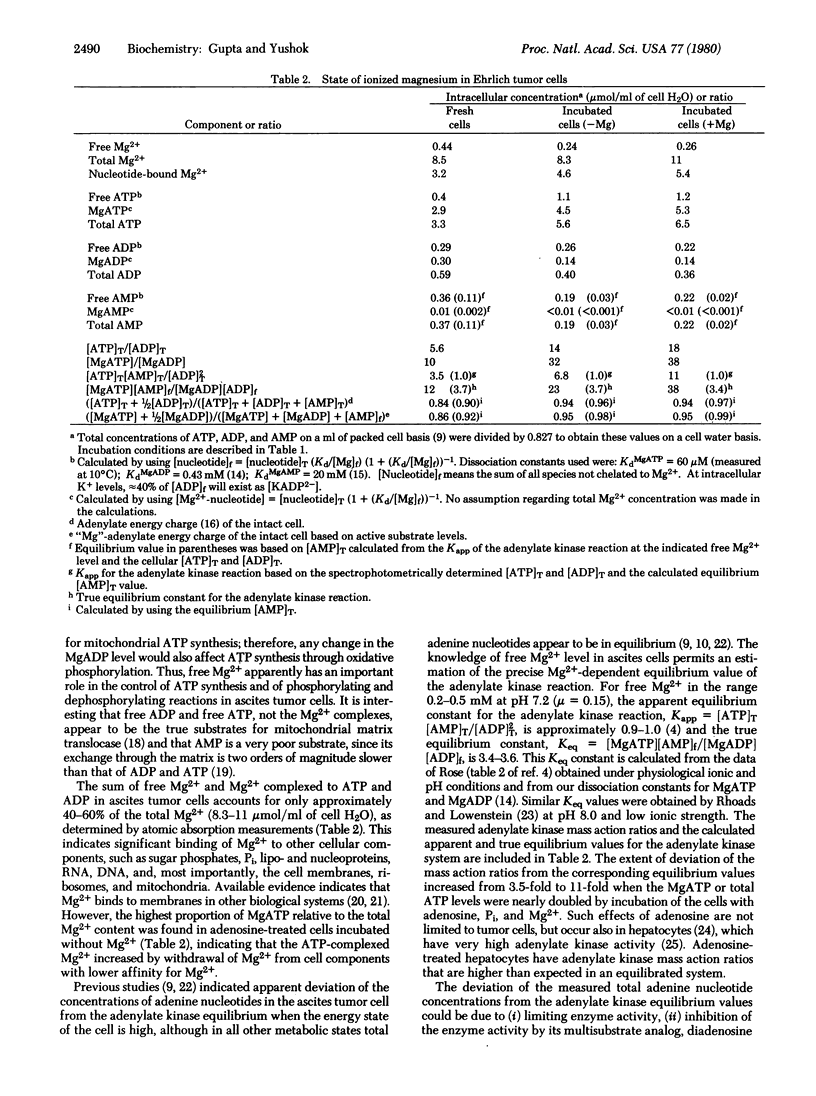
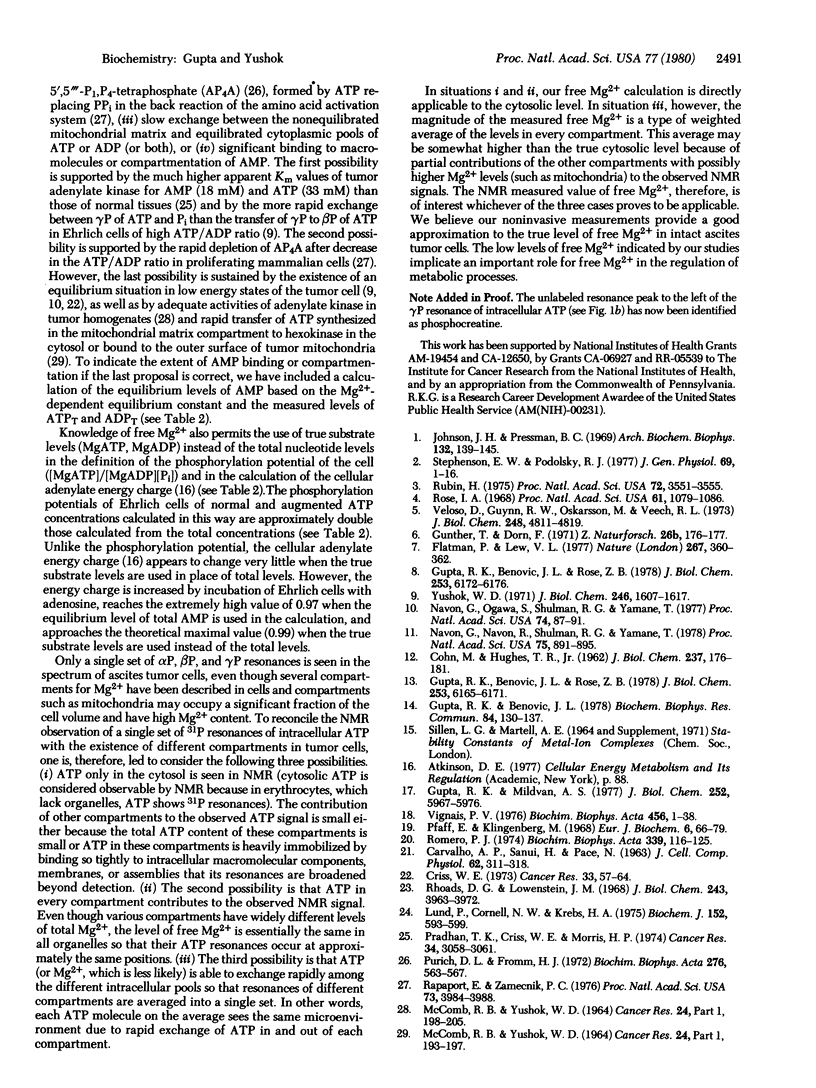
Selected References
These references are in PubMed. This may not be the complete list of references from this article.
- CARVALHO A. P., SANUI H., PACE N. CALCIUM AND MAGNESIUM BINDING PROPERTIES OF CELL MEMBRANE MATERIALS. J Cell Physiol. 1963 Dec;62:311–317. doi: 10.1002/jcp.1030620311. [DOI] [PubMed] [Google Scholar]
- COHN M., HUGHES T. R., Jr Nuclear magnetic resonance spectra of adenosine di- and triphosphate. II. Effect of complexing with divalent metal ions. J Biol Chem. 1962 Jan;237:176–181. [PubMed] [Google Scholar]
- Criss W. E. Control of the adenylate charge in Novikoff ascites cells. Cancer Res. 1973 Jan;33(1):57–64. [PubMed] [Google Scholar]
- Flatman P., Lew V. L. Use of ionophore A23187 to measure and to control free and bound cytoplasmic Mg in intact red cells. Nature. 1977 May 26;267(5609):360–362. doi: 10.1038/267360a0. [DOI] [PubMed] [Google Scholar]
- Gupta R. K., Benovic J. L. Magnetic resonance studies of the interaction of divalent metal cations with 2,3-bisphosphoglycerate. Biochem Biophys Res Commun. 1978 Sep 14;84(1):130–137. doi: 10.1016/0006-291x(78)90273-5. [DOI] [PubMed] [Google Scholar]
- Gupta R. K., Benovic J. L., Rose Z. B. Magnetic resonance studies of the binding of ATP and cations to human hemoglobin. J Biol Chem. 1978 Sep 10;253(17):6165–6171. [PubMed] [Google Scholar]
- Gupta R. K., Benovic J. L., Rose Z. B. The determination of the free magnesium level in the human red blood cell by 31P NMR. J Biol Chem. 1978 Sep 10;253(17):6172–6176. [PubMed] [Google Scholar]
- Gupta R. K., Mildvan A. S. Structures of enzyme-bound metal-nucleotide complexes in the phosphoryl transfer reaction of muscle pyruvate kinase. 31P NMR studies with magnesium and kinetic studies with chromium nucleotides. J Biol Chem. 1977 Sep 10;252(17):5967–5976. [PubMed] [Google Scholar]
- Günther T., Dorn F. Die intracelluläre Mg-Ionenaktivität in verschiedenen Säugetierzellen. Z Naturforsch B. 1971 Feb;26(2):175–177. [PubMed] [Google Scholar]
- Johnson J. H., Pressman B. C. Rates of exchange of mitochondrial Mg2+ determined from 28Mg flux measurements. Arch Biochem Biophys. 1969 Jun;132(1):139–145. doi: 10.1016/0003-9861(69)90345-2. [DOI] [PubMed] [Google Scholar]
- Lund P., Cornell N. W., Krebs H. A. Effect of adenosine on the adenine nucleotide content and metabolism of hepatocytes. Biochem J. 1975 Dec;152(3):593–599. doi: 10.1042/bj1520593. [DOI] [PMC free article] [PubMed] [Google Scholar]
- MCCOMB R. B., YUSHOK W. D. METABOLISM OF ASCITES TUMOR CELLS. III. EFFECT OF 2-DEOXYGLUCOSE PHOSPHORYLATION ON PHOSPHORUS METABOLISM. Cancer Res. 1964 Feb;24:193–197. [PubMed] [Google Scholar]
- MCCOMB R. B., YUSHOK W. D. METABOLISM OF ASCITES TUMOR CELLS. IV. ENZYMATIC REACTIONS INVOLVED IN ADENOSINETRIPHOSPHATE DEGRADATION INDUCED BY 2-DEOXYGLUCOSE. Cancer Res. 1964 Feb;24:198–205. [PubMed] [Google Scholar]
- Navon G., Navon R., Shulman R. G., Yamane T. Phosphate metabolites in lymphoid, Friend erythroleukemia, and HeLa cells observed by high-resolution 31P nuclear magnetic resonance. Proc Natl Acad Sci U S A. 1978 Feb;75(2):891–895. doi: 10.1073/pnas.75.2.891. [DOI] [PMC free article] [PubMed] [Google Scholar]
- Navon G., Ogawa S., Shulman R. G., Yamane T. 31P nuclear magnetic resonance studies of Ehrlich ascites tumor cells. Proc Natl Acad Sci U S A. 1977 Jan;74(1):87–91. doi: 10.1073/pnas.74.1.87. [DOI] [PMC free article] [PubMed] [Google Scholar]
- Pfaff E., Klingenberg M. Adenine nucleotide translocation of mitochondria. 1. Specificity and control. Eur J Biochem. 1968 Oct 17;6(1):66–79. doi: 10.1111/j.1432-1033.1968.tb00420.x. [DOI] [PubMed] [Google Scholar]
- Pradhan T. K., Criss W. E., Morris H. P. Kinetic regulation of adenylate kinases from muscle, liver, and hepatoma. Cancer Res. 1974 Nov;34(11):3058–3061. [PubMed] [Google Scholar]
- Purich D. L., Fromm H. J. Inhibition of rabbit skeletal muscle adenylate kinase by the transition state analogue, P 1 ,P 4 -di(adenosine-5')tetraphosphate. Biochim Biophys Acta. 1972 Aug 28;276(2):563–567. doi: 10.1016/0005-2744(72)91021-2. [DOI] [PubMed] [Google Scholar]
- Rhoads D. G., Lowenstein J. M. Initial velocity and equilibrium kinetics of myokinase. J Biol Chem. 1968 Jul 25;243(14):3963–3972. [PubMed] [Google Scholar]
- Romero P. J. The role of membrane-bound magnesium in the permeability of ghosts to K+. Biochim Biophys Acta. 1974 Feb 26;339(1):116–125. doi: 10.1016/0005-2736(74)90337-x. [DOI] [PubMed] [Google Scholar]
- Rose I. A. The state of magnesium in cells as estimated from the adenylate kinase equilibrium. Proc Natl Acad Sci U S A. 1968 Nov;61(3):1079–1086. doi: 10.1073/pnas.61.3.1079. [DOI] [PMC free article] [PubMed] [Google Scholar]
- Rubin H. Central role for magnesium in coordinate control of metabolism and growth in animal cells. Proc Natl Acad Sci U S A. 1975 Sep;72(9):3551–3555. doi: 10.1073/pnas.72.9.3551. [DOI] [PMC free article] [PubMed] [Google Scholar]
- Stephenson E. W., Podolsky R. J. Regulation by magnesium of intracellular calcium movement in skinned muscle fibers. J Gen Physiol. 1977 Jan;69(1):1–16. doi: 10.1085/jgp.69.1.1. [DOI] [PMC free article] [PubMed] [Google Scholar]
- Veloso D., Guynn R. W., Oskarsson M., Veech R. L. The concentrations of free and bound magnesium in rat tissues. Relative constancy of free Mg 2+ concentrations. J Biol Chem. 1973 Jul 10;248(13):4811–4819. [PubMed] [Google Scholar]
- Vignais P. V. Molecular and physiological aspects of adenine nucleotide transport in mitochondria. Biochim Biophys Acta. 1976 Apr 30;456(1):1–38. doi: 10.1016/0304-4173(76)90007-0. [DOI] [PubMed] [Google Scholar]
- Yushok W. D. Control mechanisms of adenine nucleotide metabolism of ascites tumor cells. J Biol Chem. 1971 Mar 25;246(6):1607–1617. [PubMed] [Google Scholar]