Abstract
The self-assembly of globular proteins is often portrayed as a nucleation process in which the hydrogen bonding in segments of secondary structure is the precondition for further folding. We show here that this concept is unlikely because both the buried interior regions and the peptide chain turns of the folded protein (i.e., inside and outside) are predicted solely by the hydrophobicity of the residues, taken in sequential order along the chain. The helices and strands span the protein, and this observed secondary structure is seen to coincide with the regions predicted to be buried from hydrophobicity considerations alone. Our evidence suggests that linear chain regions rich in hydrophobic residues serve as small clusters that fold against each other, with concomitant or even later fixation of secondary structure. A helix or strand would arise in this folding process as one of a few energetically favorable alternatives for a given cluster, followed by a shift in the equilibrium between secondary structure conformers upon cluster association. the linera chain hydrophobicity alternates between locally maximal and minimal values, and these extrema partition the polypeptide chain into structural segments. This partitioning is seen in the x-ray structure as isodirectional segments bracketed between peptide chain-turns, with the segments expressed most often as helices and strands. the segment interactions define the geometry of the molecular interior and the chain-turns describe the predominant features of the molecular coastline. The segmentation of the molecule by linear chain hydrophobicity imposes a major geometric constraint upon possible folding events.
Full text
PDF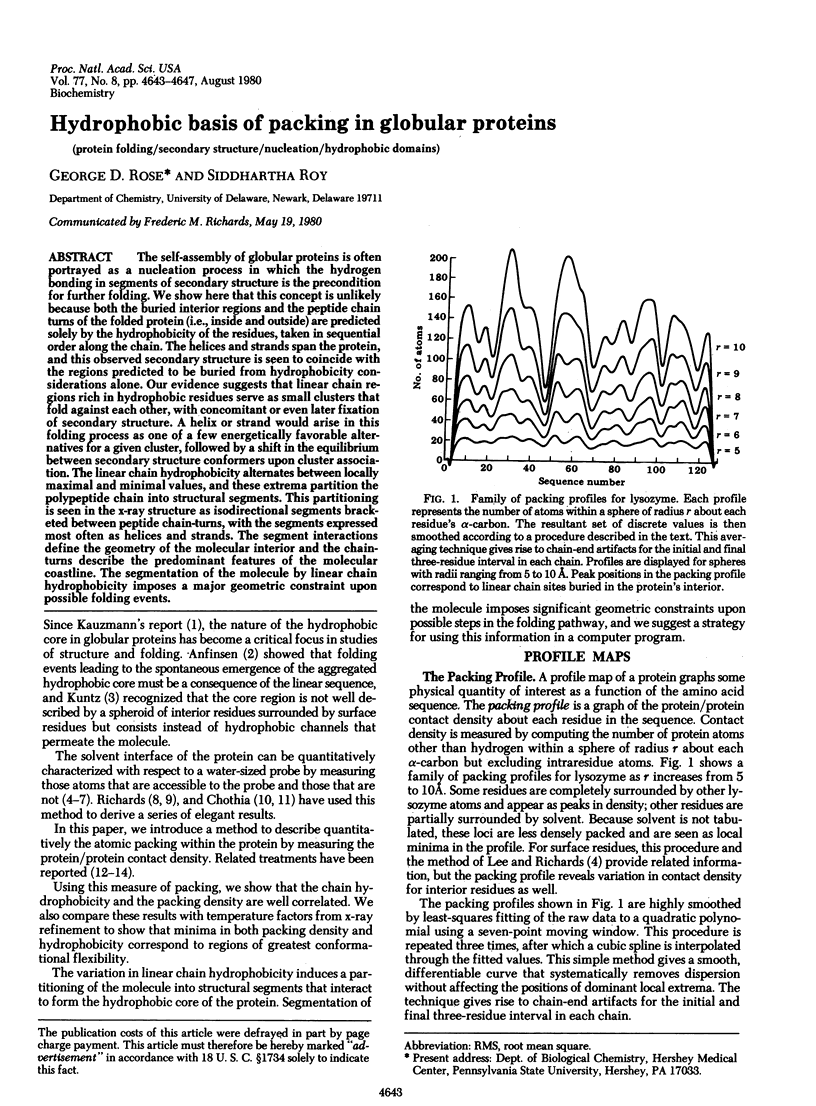
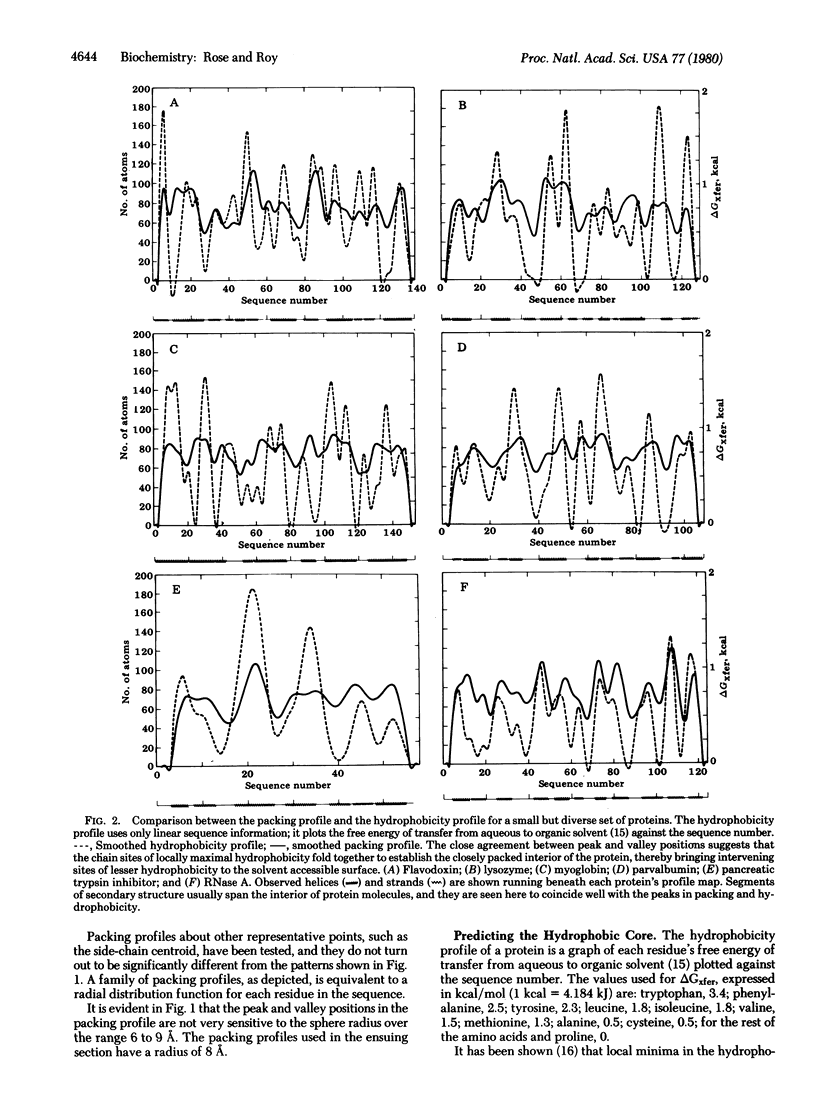
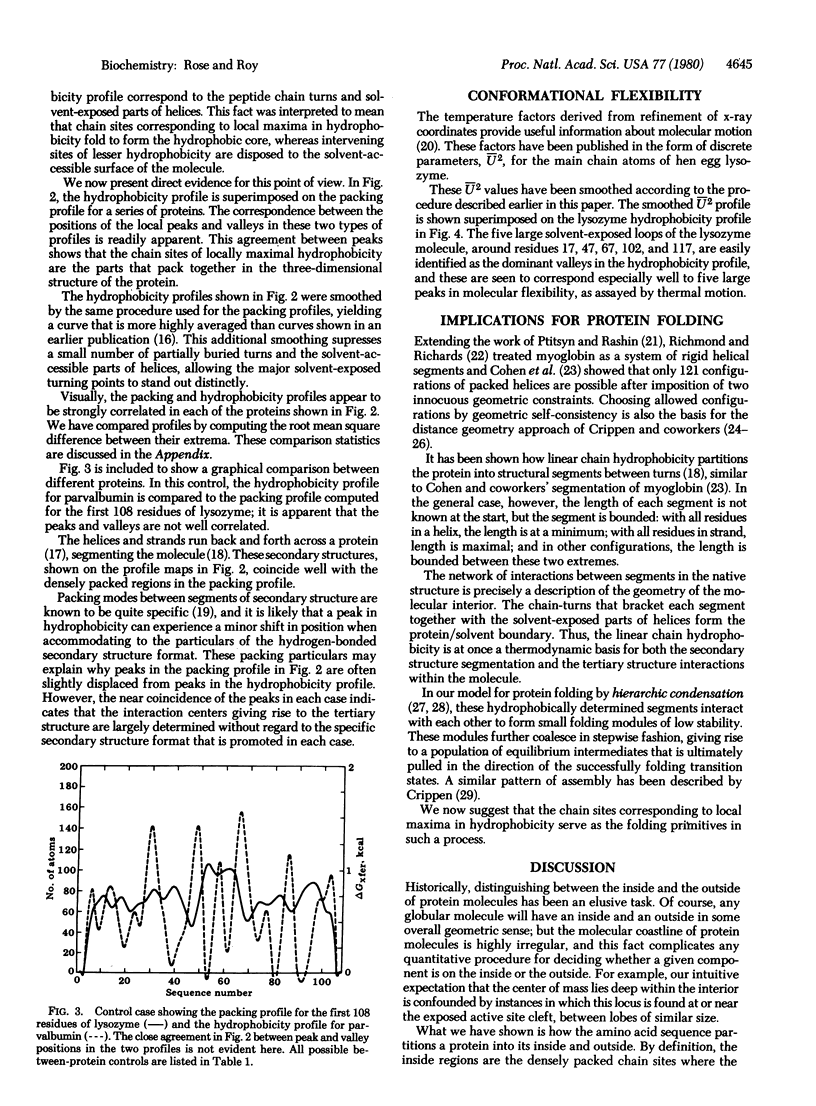
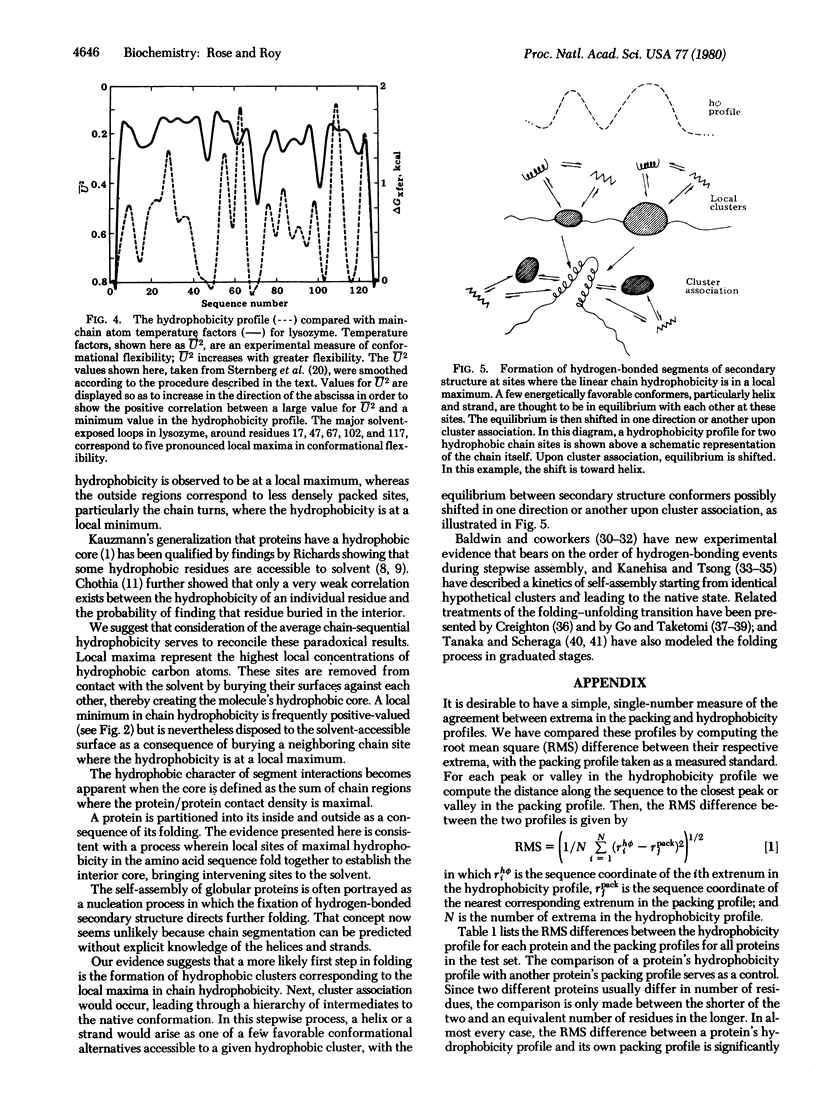
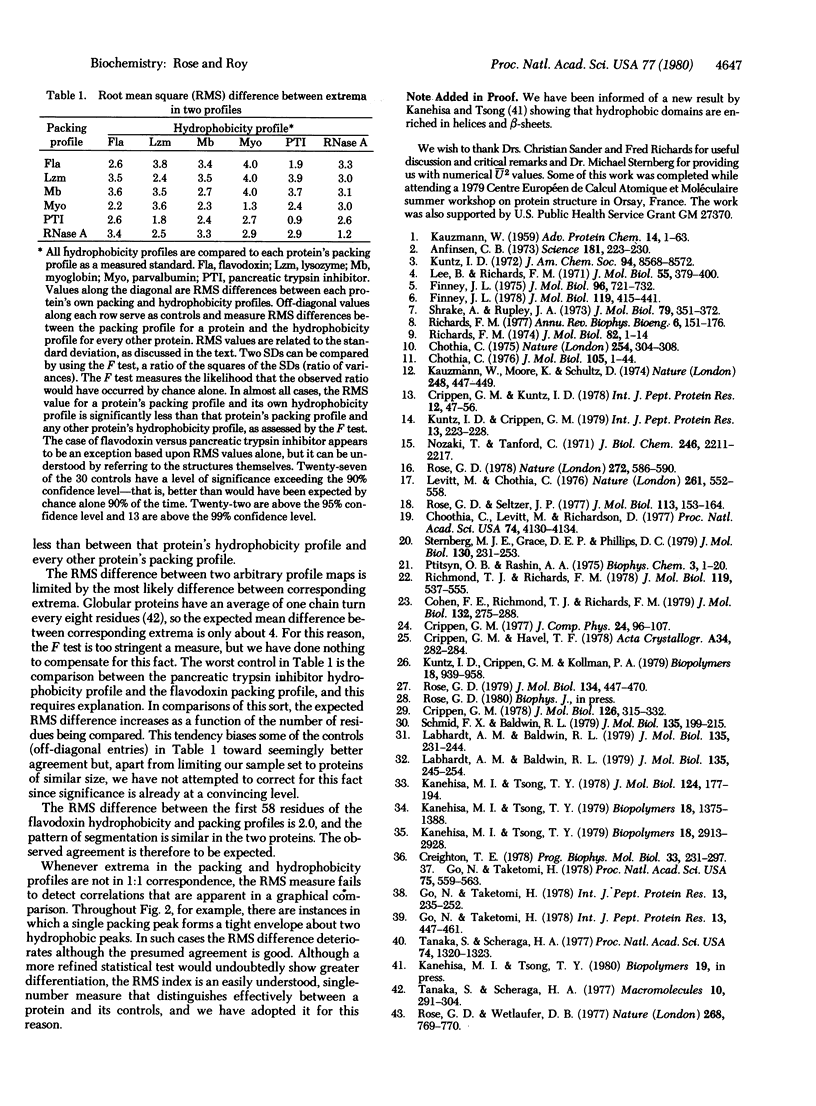
Images in this article
Selected References
These references are in PubMed. This may not be the complete list of references from this article.
- Anfinsen C. B. Principles that govern the folding of protein chains. Science. 1973 Jul 20;181(4096):223–230. doi: 10.1126/science.181.4096.223. [DOI] [PubMed] [Google Scholar]
- Chothia C., Levitt M., Richardson D. Structure of proteins: packing of alpha-helices and pleated sheets. Proc Natl Acad Sci U S A. 1977 Oct;74(10):4130–4134. doi: 10.1073/pnas.74.10.4130. [DOI] [PMC free article] [PubMed] [Google Scholar]
- Chothia C. Structural invariants in protein folding. Nature. 1975 Mar 27;254(5498):304–308. doi: 10.1038/254304a0. [DOI] [PubMed] [Google Scholar]
- Chothia C. The nature of the accessible and buried surfaces in proteins. J Mol Biol. 1976 Jul 25;105(1):1–12. doi: 10.1016/0022-2836(76)90191-1. [DOI] [PubMed] [Google Scholar]
- Cohen F. E., Richmond T. J., Richards F. M. Protein folding: evaluation of some simple rules for the assembly of helices into tertiary structures with myoglobin as an example. J Mol Biol. 1979 Aug 15;132(3):275–288. doi: 10.1016/0022-2836(79)90260-2. [DOI] [PubMed] [Google Scholar]
- Creighton T. E. Experimental studies of protein folding and unfolding. Prog Biophys Mol Biol. 1978;33(3):231–297. doi: 10.1016/0079-6107(79)90030-0. [DOI] [PubMed] [Google Scholar]
- Crippen G. M., Kuntz I. D. A survey of atom packing in globular proteins. Int J Pept Protein Res. 1978 Jul;12(1):47–56. doi: 10.1111/j.1399-3011.1978.tb02867.x. [DOI] [PubMed] [Google Scholar]
- Crippen G. M. The tree structural organization of proteins. J Mol Biol. 1978 Dec 15;126(3):315–332. doi: 10.1016/0022-2836(78)90043-8. [DOI] [PubMed] [Google Scholar]
- Finney J. L. Volume occupation, environment and accessibility in proteins. The problem of the protein surface. J Mol Biol. 1975 Aug 25;96(4):721–732. doi: 10.1016/0022-2836(75)90148-5. [DOI] [PubMed] [Google Scholar]
- Finney J. L. Volume occupation, environment, and accessibility in proteins. Environment and molecular area of RNase-S. J Mol Biol. 1978 Mar 5;119(3):415–441. doi: 10.1016/0022-2836(78)90223-1. [DOI] [PubMed] [Google Scholar]
- Go N., Taketomi H. Respective roles of short- and long-range interactions in protein folding. Proc Natl Acad Sci U S A. 1978 Feb;75(2):559–563. doi: 10.1073/pnas.75.2.559. [DOI] [PMC free article] [PubMed] [Google Scholar]
- Go N., Taketomi H. Studies on protein folding, unfolding and fluctuations by computer simulation. III. Effect of short-range interactions. Int J Pept Protein Res. 1979 Mar;13(3):235–252. doi: 10.1111/j.1399-3011.1979.tb01875.x. [DOI] [PubMed] [Google Scholar]
- Go N., Taketomi H. Studies on protein folding, unfolding and fluctuations by computer simulation. IV. Hydrophobic interactions. Int J Pept Protein Res. 1979 May;13(5):447–461. doi: 10.1111/j.1399-3011.1979.tb01907.x. [DOI] [PubMed] [Google Scholar]
- KAUZMANN W. Some factors in the interpretation of protein denaturation. Adv Protein Chem. 1959;14:1–63. doi: 10.1016/s0065-3233(08)60608-7. [DOI] [PubMed] [Google Scholar]
- Kanehisa M. I., Tsong T. Y. Mechanism of the multiphasic kinetics in the folding and unfolding of globular proteins. J Mol Biol. 1978 Sep 5;124(1):177–194. doi: 10.1016/0022-2836(78)90155-9. [DOI] [PubMed] [Google Scholar]
- Kauzmann W., Moore K., Schultz D. Protein densities from X-ray crystallographic coordinates. Nature. 1974 Mar 29;248(447):447–449. doi: 10.1038/248447a0. [DOI] [PubMed] [Google Scholar]
- Kuntz I. D., Crippen G. M. Protein densities. Int J Pept Protein Res. 1979 Feb;13(2):223–228. doi: 10.1111/j.1399-3011.1979.tb01872.x. [DOI] [PubMed] [Google Scholar]
- Kuntz I. D. Tertiary structure in carboxypeptidase. J Am Chem Soc. 1972 Nov 29;94(24):8568–8572. doi: 10.1021/ja00779a046. [DOI] [PubMed] [Google Scholar]
- Labhardt A. M., Baldwin R. L. Recombination of S-peptide with S-protein during folding of ribonuclease S. I. Folding pathways of the slow-folding and fast-folding classes of unfolded S-protein. J Mol Biol. 1979 Nov 25;135(1):231–244. doi: 10.1016/0022-2836(79)90349-8. [DOI] [PubMed] [Google Scholar]
- Labhardt A. M., Baldwin R. L. Recombination of S-peptide with S-protein during folding of ribonuclease S. II. Kinetic characterization of a stable folding intermediate shown by S-protein at pH 1.7. J Mol Biol. 1979 Nov 25;135(1):245–254. doi: 10.1016/0022-2836(79)90350-4. [DOI] [PubMed] [Google Scholar]
- Lee B., Richards F. M. The interpretation of protein structures: estimation of static accessibility. J Mol Biol. 1971 Feb 14;55(3):379–400. doi: 10.1016/0022-2836(71)90324-x. [DOI] [PubMed] [Google Scholar]
- Levitt M., Chothia C. Structural patterns in globular proteins. Nature. 1976 Jun 17;261(5561):552–558. doi: 10.1038/261552a0. [DOI] [PubMed] [Google Scholar]
- Nozaki Y., Tanford C. The solubility of amino acids and two glycine peptides in aqueous ethanol and dioxane solutions. Establishment of a hydrophobicity scale. J Biol Chem. 1971 Apr 10;246(7):2211–2217. [PubMed] [Google Scholar]
- Ptitsyn O. B., Rashin A. A. A model of myoglobin self-organization. Biophys Chem. 1975 Feb;3(1):1–20. doi: 10.1016/0301-4622(75)80033-0. [DOI] [PubMed] [Google Scholar]
- Richards F. M. Areas, volumes, packing and protein structure. Annu Rev Biophys Bioeng. 1977;6:151–176. doi: 10.1146/annurev.bb.06.060177.001055. [DOI] [PubMed] [Google Scholar]
- Richards F. M. The interpretation of protein structures: total volume, group volume distributions and packing density. J Mol Biol. 1974 Jan 5;82(1):1–14. doi: 10.1016/0022-2836(74)90570-1. [DOI] [PubMed] [Google Scholar]
- Richmond T. J., Richards F. M. Packing of alpha-helices: geometrical constraints and contact areas. J Mol Biol. 1978 Mar 15;119(4):537–555. doi: 10.1016/0022-2836(78)90201-2. [DOI] [PubMed] [Google Scholar]
- Rose G. D. Hierarchic organization of domains in globular proteins. J Mol Biol. 1979 Nov 5;134(3):447–470. doi: 10.1016/0022-2836(79)90363-2. [DOI] [PubMed] [Google Scholar]
- Rose G. D. Prediction of chain turns in globular proteins on a hydrophobic basis. Nature. 1978 Apr 13;272(5654):586–590. doi: 10.1038/272586a0. [DOI] [PubMed] [Google Scholar]
- Rose G. D., Seltzer J. P. A new algorithm for finding the peptide chain turns in a globular protein. J Mol Biol. 1977 Jun 15;113(1):153–164. doi: 10.1016/0022-2836(77)90046-8. [DOI] [PubMed] [Google Scholar]
- Rose G. D., Wetlaufer D. B. The number of turns in globular proteins. Nature. 1977 Aug 25;268(5622):769–770. doi: 10.1038/268769a0. [DOI] [PubMed] [Google Scholar]
- Schmid F. X., Baldwin R. L. Detection of an early intermediate in the folding of ribonuclease A by protection of amide protons against exchange. J Mol Biol. 1979 Nov 25;135(1):199–215. doi: 10.1016/0022-2836(79)90347-4. [DOI] [PubMed] [Google Scholar]
- Shrake A., Rupley J. A. Environment and exposure to solvent of protein atoms. Lysozyme and insulin. J Mol Biol. 1973 Sep 15;79(2):351–371. doi: 10.1016/0022-2836(73)90011-9. [DOI] [PubMed] [Google Scholar]
- Sternberg M. J., Grace D. E., Phillips D. C. Dynamic information from protein crystallography. An analysis of temperature factors from refinement of the hen egg-white lysozyme structure. J Mol Biol. 1979 May 25;130(3):231–252. doi: 10.1016/0022-2836(79)90539-4. [DOI] [PubMed] [Google Scholar]
- Tanaka S., Scheraga H. A. Hypothesis about the mechanism of protein folding. Macromolecules. 1977 Mar-Apr;10(2):291–304. doi: 10.1021/ma60056a015. [DOI] [PubMed] [Google Scholar]
- Tanaka S., Scheraga H. A. Model of protein folding: incorporation of a one-dimensional short-range (Ising) model into a three-dimensional model. Proc Natl Acad Sci U S A. 1977 Apr;74(4):1320–1323. doi: 10.1073/pnas.74.4.1320. [DOI] [PMC free article] [PubMed] [Google Scholar]