Abstract
The relationship between tension and Ca2+ concentration in single skinned muscle fibers has been determined with a high density of experimental points and the data have been fitted by a least squares method to the Hill equation. We find that the mean Hill coefficient for the slope of the tension/Ca2+ relationship is between 5 and 6, and the pKd is about 5.9. Because there are four Ca2+ binding sites on troponin C, and only two of these regulate hydrolysis of MgATP, we conclude that the regulation of tension by Ca2+ binding is greatly modified by other factors. One important factor is the time required for a cross-bridge to complete a cycle once initiated, relative to the time Ca2+ remains bound to troponin C. The pCa/tension relationship will shift to higher pCa values as the ratio of cross-bridge cycle time to the Ca2+ bound time increases. For example, the pCa/tension curve may progressively shift to the left with increase in tension because strain in the myofilament lattice progressively increases the cycle time. This left shift will produce a pCa/tension relationship that is steeper than the actual Ca2+ binding curve. The anticipated shift of the pCa/tension curve with cycle time also bears on interpretations of earlier experiments on the "active state" and on the effects of Ca2+ on the maximal velocity of shortening.
Full text
PDF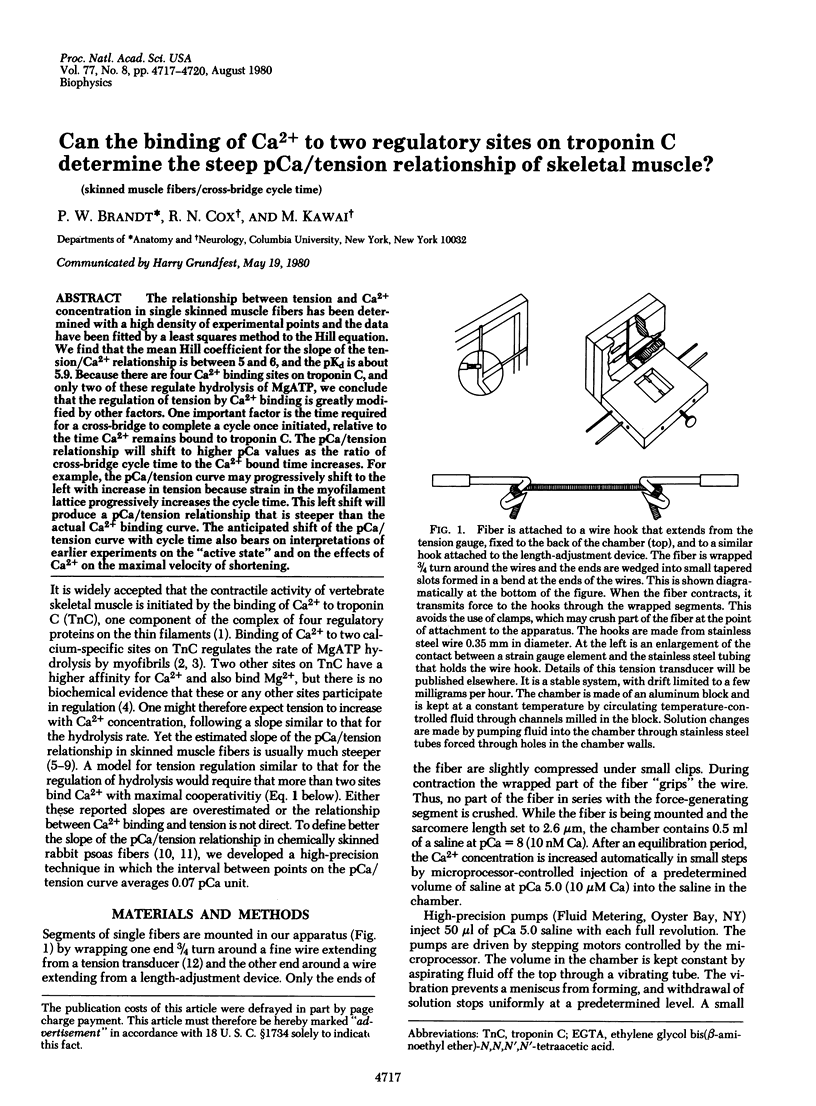
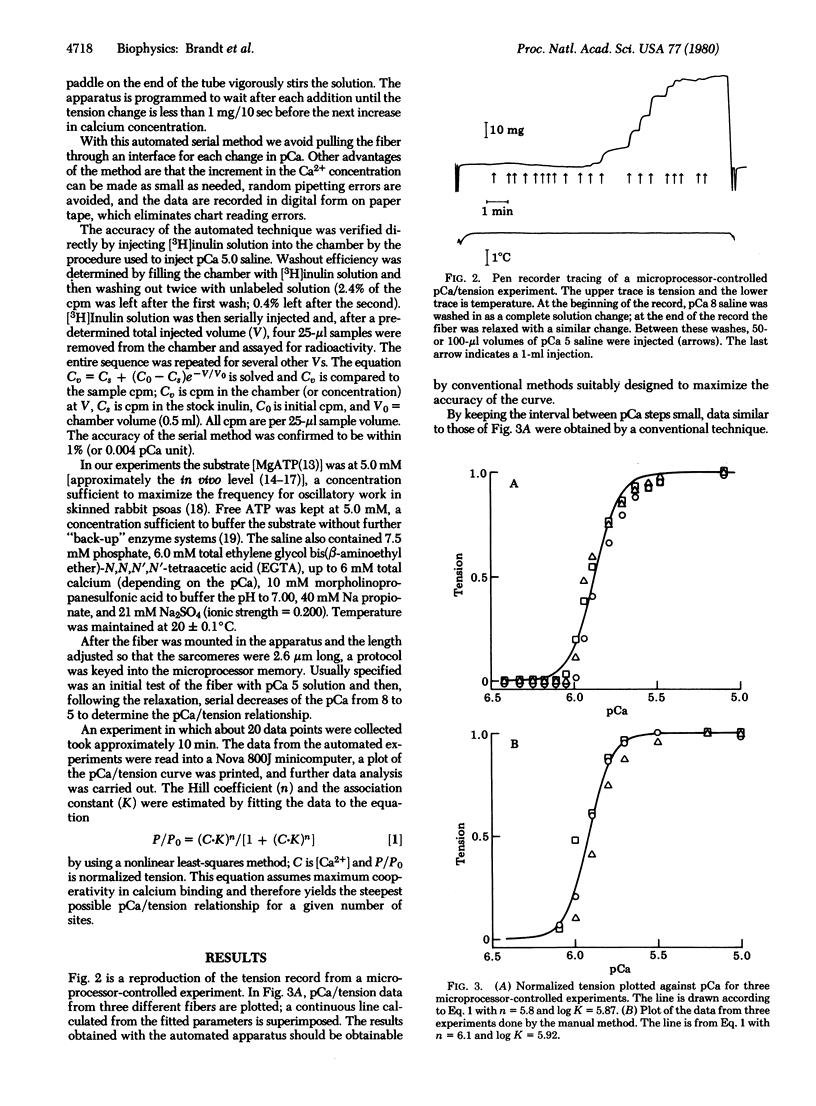
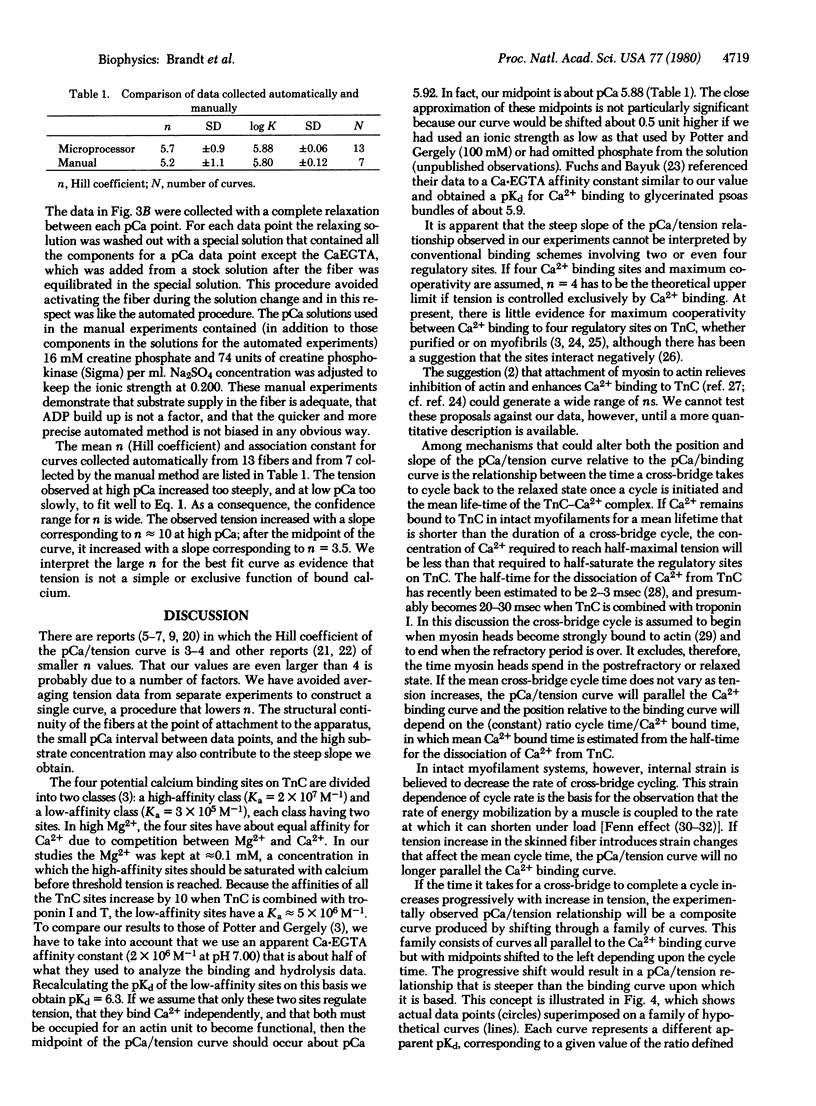
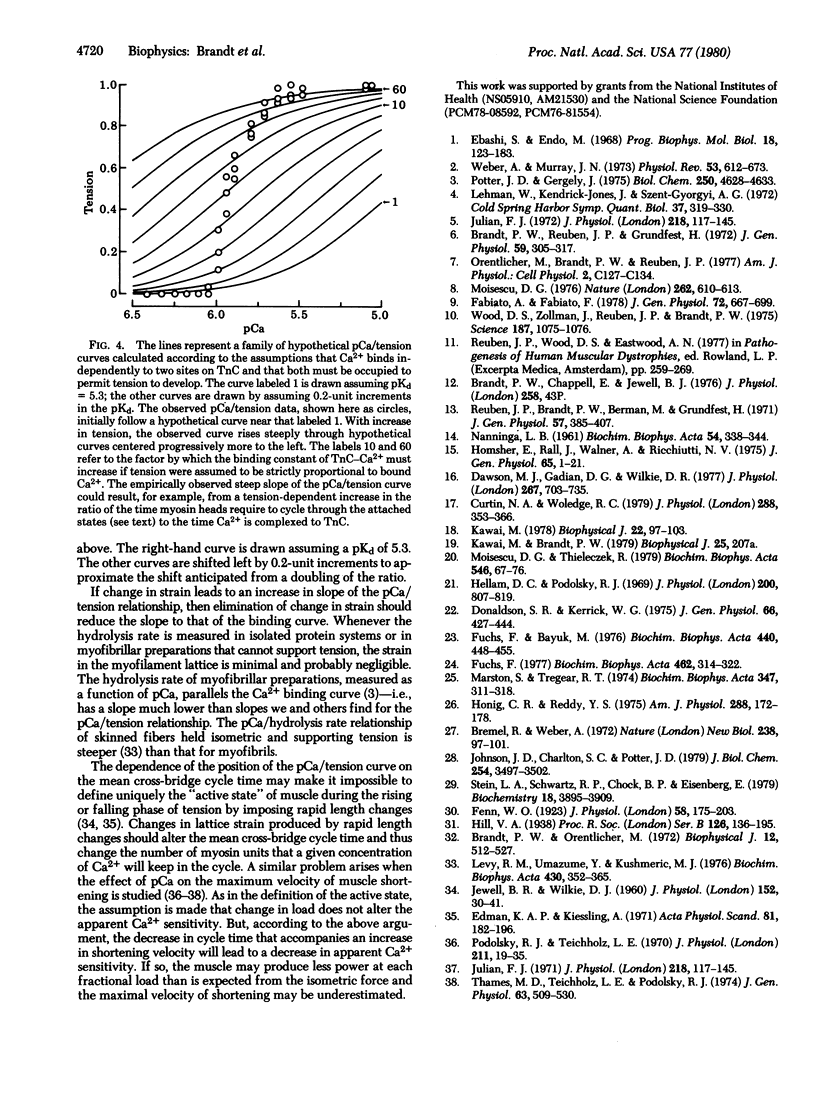
Selected References
These references are in PubMed. This may not be the complete list of references from this article.
- Brandt P. W., Chappell E., Jewell B. R. Proceedings: A robust transducer suitable for measuring forces of 1 muN. J Physiol. 1976 Jun;258(2):43P–44P. [PubMed] [Google Scholar]
- Brandt P. W., Orentlicher M. Muscle energetics and the Fenn effect. Biophys J. 1972 May;12(5):512–527. doi: 10.1016/S0006-3495(72)86100-9. [DOI] [PMC free article] [PubMed] [Google Scholar]
- Brandt P. W., Reuben J. P., Grundfest H. Regulation of tension in the skinned crayfish muscle fiber. II. Role of calcium. J Gen Physiol. 1972 Mar;59(3):305–317. doi: 10.1085/jgp.59.3.305. [DOI] [PMC free article] [PubMed] [Google Scholar]
- Bremel R. D., Weber A. Cooperation within actin filament in vertebrate skeletal muscle. Nat New Biol. 1972 Jul 26;238(82):97–101. doi: 10.1038/newbio238097a0. [DOI] [PubMed] [Google Scholar]
- Curtin N. A., Woledge R. C. Chemical change and energy production during contraction of frog muscle: how are their time courses related? J Physiol. 1979 Mar;288:353–366. [PMC free article] [PubMed] [Google Scholar]
- Dawson M. J., Gadian D. G., Wilkie D. R. Contraction and recovery of living muscles studies by 31P nuclear magnetic resonance. J Physiol. 1977 Jun;267(3):703–735. doi: 10.1113/jphysiol.1977.sp011835. [DOI] [PMC free article] [PubMed] [Google Scholar]
- Donaldson S. K., Kerrick W. G. Characterization of the effects of Mg2+ on Ca2+- and Sr2+-activated tension generation of skinned skeletal muscle fibers. J Gen Physiol. 1975 Oct;66(4):427–444. doi: 10.1085/jgp.66.4.427. [DOI] [PMC free article] [PubMed] [Google Scholar]
- Ebashi S., Endo M. Calcium ion and muscle contraction. Prog Biophys Mol Biol. 1968;18:123–183. doi: 10.1016/0079-6107(68)90023-0. [DOI] [PubMed] [Google Scholar]
- Edman K. A., Kiessling A. The time course of the active state in relation to sarcomere length and movement studied in single skeletal muscle fibres of the frog. Acta Physiol Scand. 1971 Feb;81(2):182–196. doi: 10.1111/j.1748-1716.1971.tb04891.x. [DOI] [PubMed] [Google Scholar]
- Fabiato A., Fabiato F. Myofilament-generated tension oscillations during partial calcium activation and activation dependence of the sarcomere length-tension relation of skinned cardiac cells. J Gen Physiol. 1978 Nov;72(5):667–699. doi: 10.1085/jgp.72.5.667. [DOI] [PMC free article] [PubMed] [Google Scholar]
- Fenn W. O. A quantitative comparison between the energy liberated and the work performed by the isolated sartorius muscle of the frog. J Physiol. 1923 Dec 28;58(2-3):175–203. doi: 10.1113/jphysiol.1923.sp002115. [DOI] [PMC free article] [PubMed] [Google Scholar]
- Fuchs F., Bayuk M. Cooperative binding of calcium to glycerinated skeletal muscle fibers. Biochim Biophys Acta. 1976 Aug 13;440(2):448–455. doi: 10.1016/0005-2728(76)90077-3. [DOI] [PubMed] [Google Scholar]
- Fuchs F. Cooperative interactions between calcium-binding sites on glycerinated muscle fibers. The influence of cross-bridge attachment. Biochim Biophys Acta. 1977 Nov 17;462(2):314–322. doi: 10.1016/0005-2728(77)90130-x. [DOI] [PubMed] [Google Scholar]
- Hellam D. C., Podolsky R. J. Force measurements in skinned muscle fibres. J Physiol. 1969 Feb;200(3):807–819. doi: 10.1113/jphysiol.1969.sp008723. [DOI] [PMC free article] [PubMed] [Google Scholar]
- Homsher E., Rall J. A., Wallner A., Ricchiuti N. V. Energy liberation and chemical change in frog skeletal muscle during single isometric tetanic contractions. J Gen Physiol. 1975 Jan;65(1):1–21. doi: 10.1085/jgp.65.1.1. [DOI] [PMC free article] [PubMed] [Google Scholar]
- Honig C. R., Reddy Y. S. Interaction of Ca++ binding sites of troponin: significance for Ca++ movements. Am J Physiol. 1975 Jan;228(1):172–178. doi: 10.1152/ajplegacy.1975.228.1.172. [DOI] [PubMed] [Google Scholar]
- JEWELL B. R., WILKIE D. R. The mechanical properties of relaxing muscle. J Physiol. 1960 Jun;152:30–47. doi: 10.1113/jphysiol.1960.sp006467. [DOI] [PMC free article] [PubMed] [Google Scholar]
- Johnson J. D., Charlton S. C., Potter J. D. A fluorescence stopped flow analysis of Ca2+ exchange with troponin C. J Biol Chem. 1979 May 10;254(9):3497–3502. [PubMed] [Google Scholar]
- Julian F. J. The effect of calcium on the force-velocity relation of briefly glycerinated frog muscle fibres. J Physiol. 1971 Oct;218(1):117–145. doi: 10.1113/jphysiol.1971.sp009607. [DOI] [PMC free article] [PubMed] [Google Scholar]
- Julian F. J. The effect of calcium on the force-velocity relation of briefly glycerinated frog muscle fibres. J Physiol. 1971 Oct;218(1):117–145. doi: 10.1113/jphysiol.1971.sp009607. [DOI] [PMC free article] [PubMed] [Google Scholar]
- Marston S., Tregear R. T. Calcium binding and the activation of fibrillar insect flight muscle. Biochim Biophys Acta. 1974 May 22;347(2):311–318. doi: 10.1016/0005-2728(74)90054-1. [DOI] [PubMed] [Google Scholar]
- Moisescu D. G. Kinetics of reaction in calcium-activated skinned muscle fibres. Nature. 1976 Aug 12;262(5569):610–613. doi: 10.1038/262610a0. [DOI] [PubMed] [Google Scholar]
- Moisescu D. G., Thieleczek R. Sarcomere length effects on the Sr2+- and Ca2+-activation curves in skinned frog muscle fibres. Biochim Biophys Acta. 1979 Apr 11;546(1):64–76. doi: 10.1016/0005-2728(79)90170-1. [DOI] [PubMed] [Google Scholar]
- NANNINGA L. B. Calculation of free magnesium, calcium and potassium in muscle. Biochim Biophys Acta. 1961 Dec 9;54:338–344. doi: 10.1016/0006-3002(61)90374-2. [DOI] [PubMed] [Google Scholar]
- Orentlicher M., Brandt P. W., Reuben J. P. Regulation of tension in skinned muscle fibers: effect of high concentrations of Mg-ATP. Am J Physiol. 1977 Nov;233(5):C127–C134. doi: 10.1152/ajpcell.1977.233.5.C127. [DOI] [PubMed] [Google Scholar]
- Podolsky R. J., Teichholz L. E. The relation between calcium and contraction kinetics in skinned muscle fibres. J Physiol. 1970 Nov;211(1):19–35. doi: 10.1113/jphysiol.1970.sp009263. [DOI] [PMC free article] [PubMed] [Google Scholar]
- Potter J. D., Gergely J. The calcium and magnesium binding sites on troponin and their role in the regulation of myofibrillar adenosine triphosphatase. J Biol Chem. 1975 Jun 25;250(12):4628–4633. [PubMed] [Google Scholar]
- Reuben J. P., Brandt P. W., Berman M., Grundfest H. Regulation of tension in the skinned crayfish muscle fiber. I. Contraction and relaxation in the absence of Ca (pCa is greater than 9). J Gen Physiol. 1971 Apr;57(4):385–407. doi: 10.1085/jgp.57.4.385. [DOI] [PMC free article] [PubMed] [Google Scholar]
- Stein L. A., Schwarz R. P., Jr, Chock P. B., Eisenberg E. Mechanism of actomyosin adenosine triphosphatase. Evidence that adenosine 5'-triphosphate hydrolysis can occur without dissociation of the actomyosin complex. Biochemistry. 1979 Sep 4;18(18):3895–3909. doi: 10.1021/bi00585a009. [DOI] [PubMed] [Google Scholar]
- Thames M. D., Teichholz L. E., Podolsky R. J. Ionic strength and the contraction kinetics of skinned muscle fibers. J Gen Physiol. 1974 Apr;63(4):509–530. doi: 10.1085/jgp.63.4.509. [DOI] [PMC free article] [PubMed] [Google Scholar]
- Weber A., Murray J. M. Molecular control mechanisms in muscle contraction. Physiol Rev. 1973 Jul;53(3):612–673. doi: 10.1152/physrev.1973.53.3.612. [DOI] [PubMed] [Google Scholar]
- Wood D. S., Zollman J., Reuben J. P., Brandt P. W. Human skeletal muscle: properties of the "chemically skinned%" fiber. Science. 1975 Mar 21;187(4181):1075–1076. doi: 10.1126/science.187.4181.1075. [DOI] [PubMed] [Google Scholar]