Abstract
Similar amino acid sequences were found in portions of bacterial enzymes that mediate different biochemical transformations. Reaction catalyzed by the enzymes include oxygenation, decarboxylation, isomerization, and hydrolysis. The proteins share a common evolutionary history because they participate in an overall catabolic process known as the beta-ketoadipate pathway. One interpretation of the sequence similarities might be that duplication of a single gene gave rise to ancestral genes for the enzymes with different catalytic activities. According to this view, homologous sequences from the ancestral gene were conserved as the proteins diverged to assume different functions. This hypothesis is vitiated by comparison of the NH2-terminal amino acid sequences of sets of enzymes that mediate identical or analogous metabolic reactions within an organism. Gene duplications giving rise to the enzymes within each set must have followed duplication of a putative ancestral gene for all the sets. Yet the amino acid sequences of the proteins within each set have diverged widely, and against this background of divergence the conservation of sequences from an ancestor common to all the enzymes is unlikely. Rather, it appears that most regions of sequence similarity shared by enzymes from different sets were acquired subsequent to their divergence from any common ancestor. In some cases it appears that relatively short regions of sequence homology were achieved by mutations causing the transfer of sequence information from one set of structural genes to structural genes in another set. Alignment of homologous amino acid sequences within any single set requires the introduction of few gaps. Because gaps are required to align sequences that have been altered by the insertion of genetic material, the evidence indicates that copies of oligonucleotides were exchanged by genetic substitution among different structural genes as they coevolved.
Full text
PDF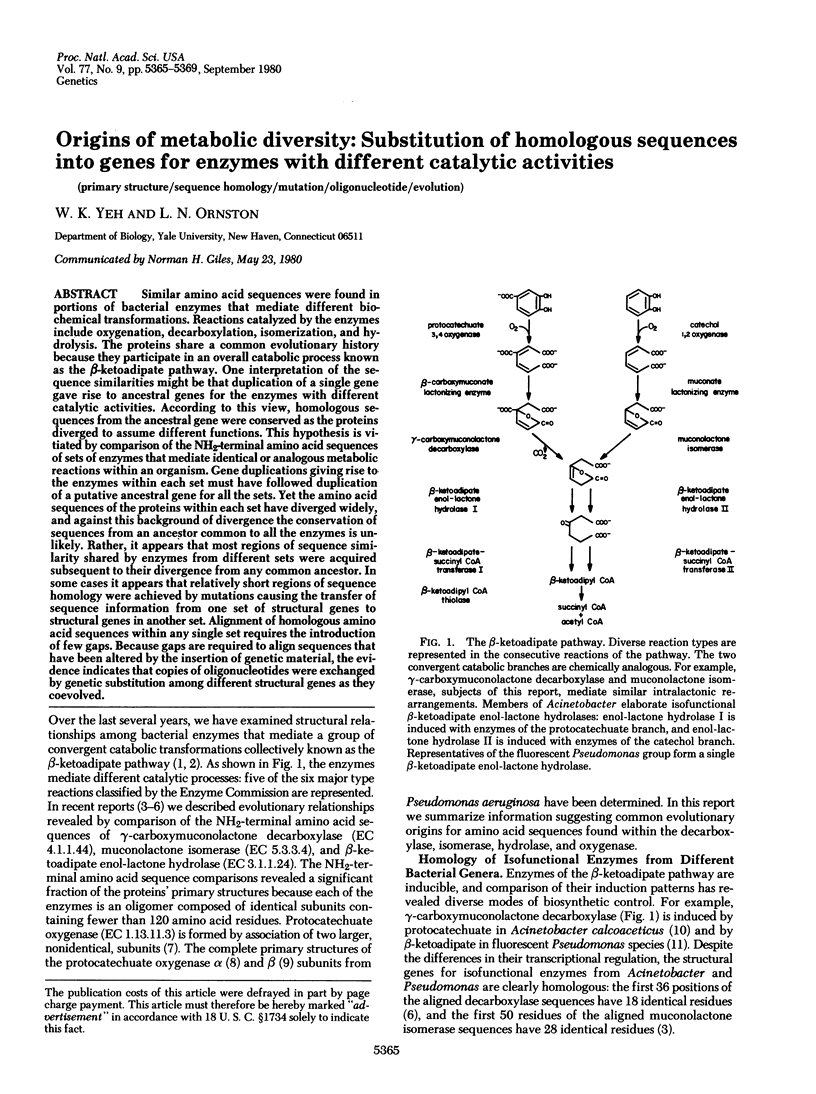
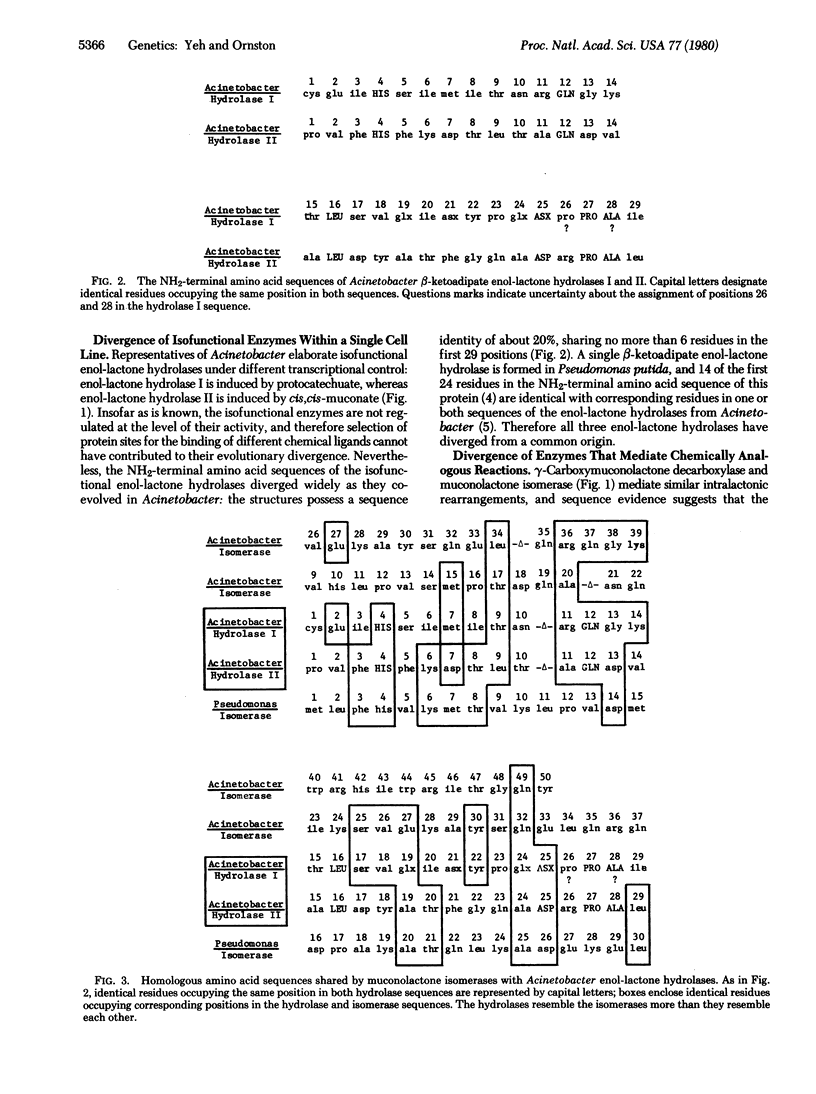
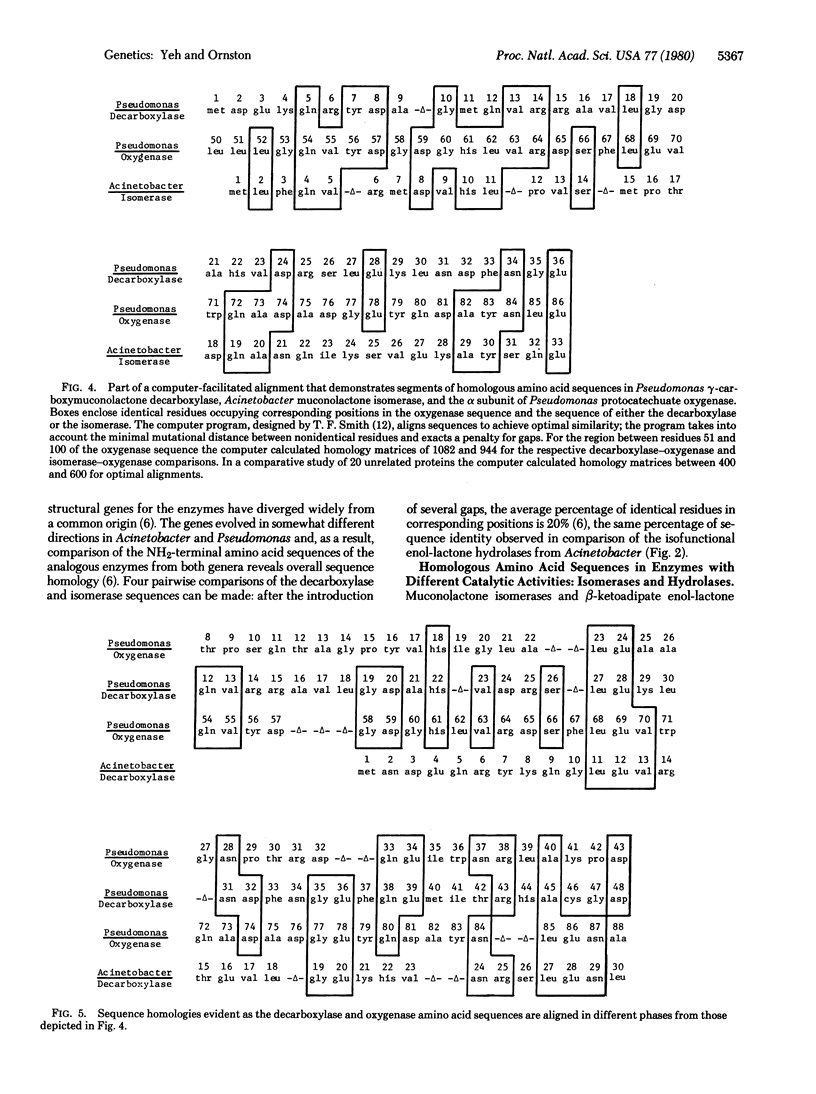
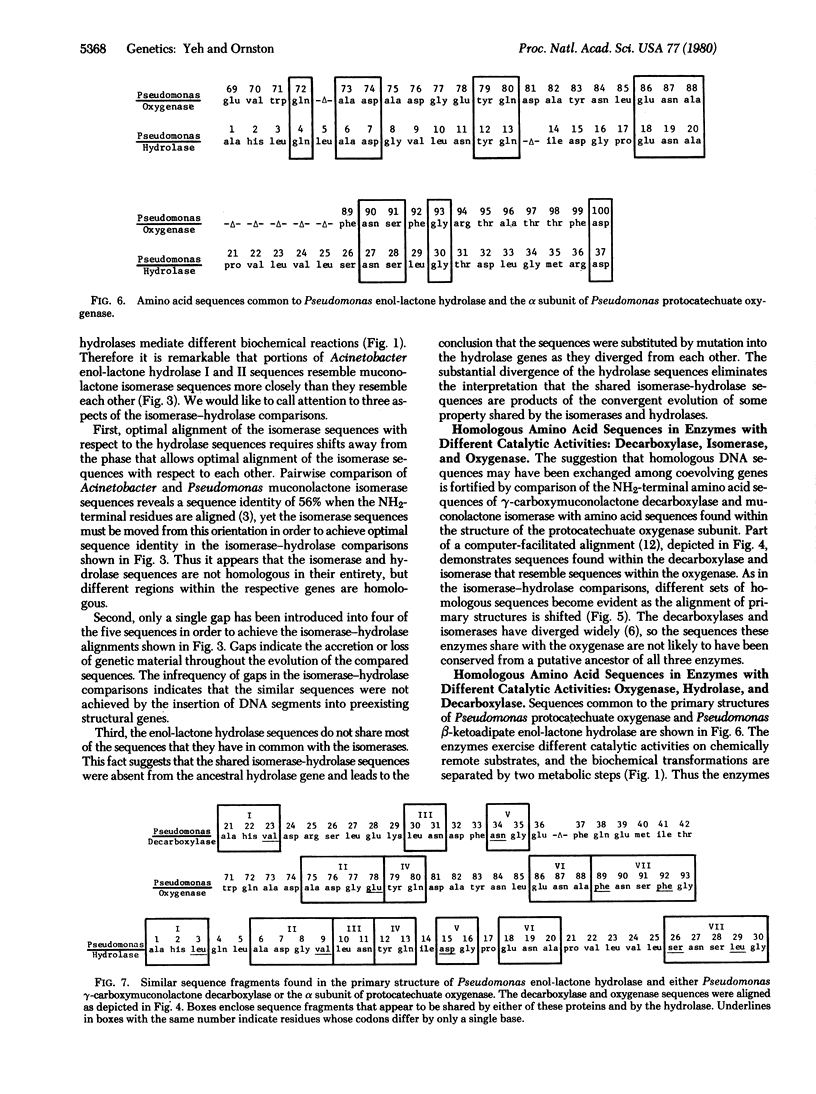
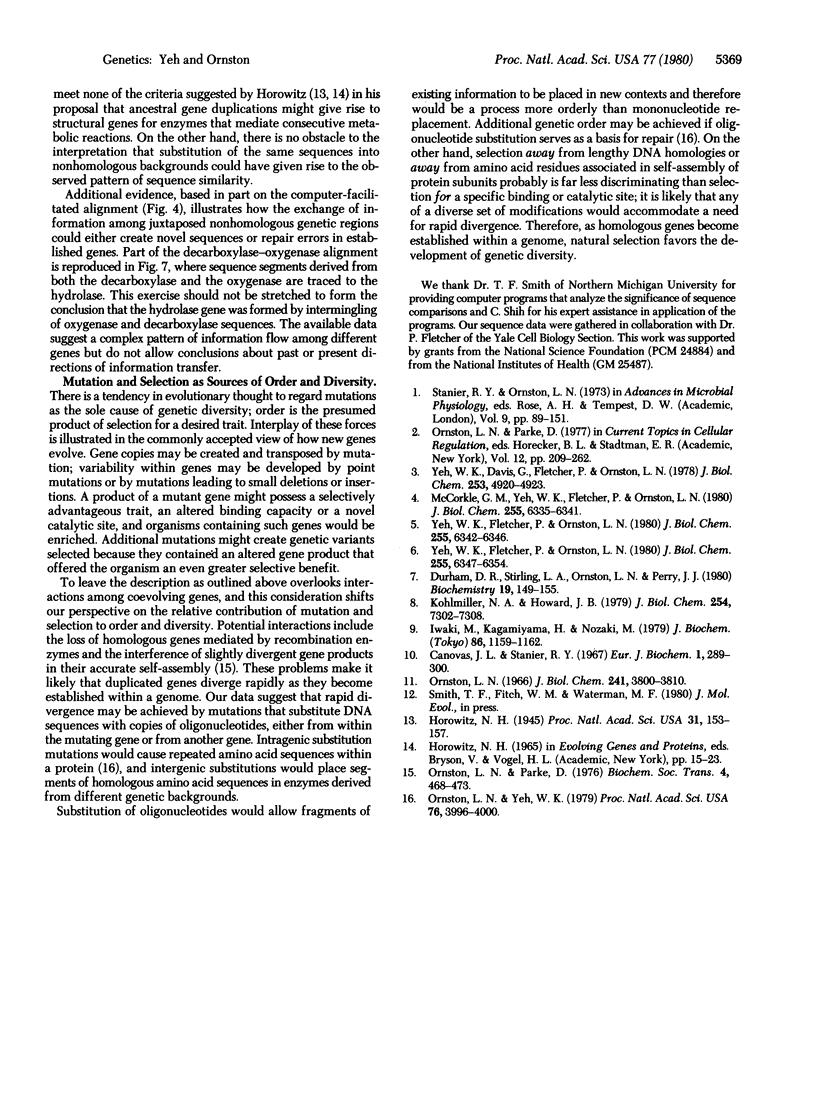
Selected References
These references are in PubMed. This may not be the complete list of references from this article.
- Cánovas J. L., Stanier R. Y. Regulation of the enzymes of the beta-ketoadipate pathway in Moraxella calcoacetica. 1. General aspects. Eur J Biochem. 1967 May;1(3):289–300. doi: 10.1007/978-3-662-25813-2_40. [DOI] [PubMed] [Google Scholar]
- Durham D. R., Stirling L. A., Ornston L. N., Perry J. J. Intergeneric evolutionary homology revealed by the study of protocatechuate 3,4-dioxygenase from Azotobacter vinelandii. Biochemistry. 1980 Jan 8;19(1):149–155. doi: 10.1021/bi00542a023. [DOI] [PubMed] [Google Scholar]
- Horowitz N. H. On the Evolution of Biochemical Syntheses. Proc Natl Acad Sci U S A. 1945 Jun;31(6):153–157. doi: 10.1073/pnas.31.6.153. [DOI] [PMC free article] [PubMed] [Google Scholar]
- Iwaki M., Kagamiyama H., Nozaki M. The complete amino acid sequence of the beta-subunit of protocatechuate 3,4-dioxygenase from Pseudomonas aeruginosa. J Biochem. 1979 Oct;86(4):1159–1162. doi: 10.1093/oxfordjournals.jbchem.a132612. [DOI] [PubMed] [Google Scholar]
- Kohlmiller N. A., Howard J. B. The primary structure of the alpha subunit of protocatechuate 3,4-dioxygenase. I. Isolation and sequence of the tryptic peptides. J Biol Chem. 1979 Aug 10;254(15):7302–7308. [PubMed] [Google Scholar]
- McCorkle G. M., Yeh W. K., Fletcher P., Ornston L. N. Repetitions in the NH2-terminal amino acid sequence of beta-ketoadipate enol-lactone hydrolase from Pseudomonas putida. J Biol Chem. 1980 Jul 10;255(13):6335–6341. [PubMed] [Google Scholar]
- Ornston L. N., Parke D. Evolution of catabolic pathways. Biochem Soc Trans. 1976;4(3):468–472. doi: 10.1042/bst0040468. [DOI] [PubMed] [Google Scholar]
- Ornston L. N. The conversion of catechol and protocatechuate to beta-ketoadipate by Pseudomonas putida. IV. Regulation. J Biol Chem. 1966 Aug 25;241(16):3800–3810. [PubMed] [Google Scholar]
- Ornston L. N., Yeh W. K. Origins of metabolic diversity: evolutionary divergence by sequence repetition. Proc Natl Acad Sci U S A. 1979 Aug;76(8):3996–4000. doi: 10.1073/pnas.76.8.3996. [DOI] [PMC free article] [PubMed] [Google Scholar]
- Yeh W. K., Davis G., Fletcher P., Ornston L. N. Homologous amino acid sequences in enzymes mediating sequential metabolic reactions. J Biol Chem. 1978 Jul 25;253(14):4920–4923. [PubMed] [Google Scholar]
- Yeh W. K., Fletcher P., Ornston L. N. Evolutionary divergence of co-selected beta-ketoadipate enol-lactone hydrolases in Acinetobacter calcoaceticus. J Biol Chem. 1980 Jul 10;255(13):6342–6346. [PubMed] [Google Scholar]
- Yeh W. K., Fletcher P., Ornston N. Homologies in the NH2-terminal amino acid sequences of gamma-carboxymuconolactone decarboxylases and muconolactone isomerases. J Biol Chem. 1980 Jul 10;255(13):6347–6354. [PubMed] [Google Scholar]