Abstract
Recently an energy-recycling model was proposed that postulates the generation of an electrochemical gradient in fermentative bacteria by carrier-mediated excretion of metabolic end products in symport with protons. In this paper experimental support for this model is given. In batch cultures of Streptococcus cremoris with glucose as the sole energy source the maximal specific growth rate decreased by 30% when the external lactate concentration was decreased from 50 to 90 mM. In the same range of external lactate concentrations the molar growth yield Y for glucose as measured in energy-limited chemostat cultures also showed a 30% drop. From Y max lactose values of S. cremoris grown in the presence and absence of added lactate it was calculated that the net energy gain from the lactate efflux system was at least 12%. Lactate efflux from de-energized cells loaded with lactate could drive the uptake of leucine. This uptake was sensitive to carbonylcyanide p-trifluoromethoxyphenylhydrazone and was only partly inhibited by dicyclohexylcarbodiimide (DCCD). The limited inhibition by DCCD of lactate-induced leucine uptake indicates that ATP hydrolysis was not the driving force for transport of leucine. Uptake studies with the lipophilic cation tetraphenylphosphonium demonstrated that lactate efflux increased the electrical potential across the membrane by 51 mV. The generation of an electrical potential by lactate efflux and the demonstration of a potassium efflux-induced uptake of lactate indicates that lactate is translocated across the membrane by a symport system with more than one proton.
Full text
PDF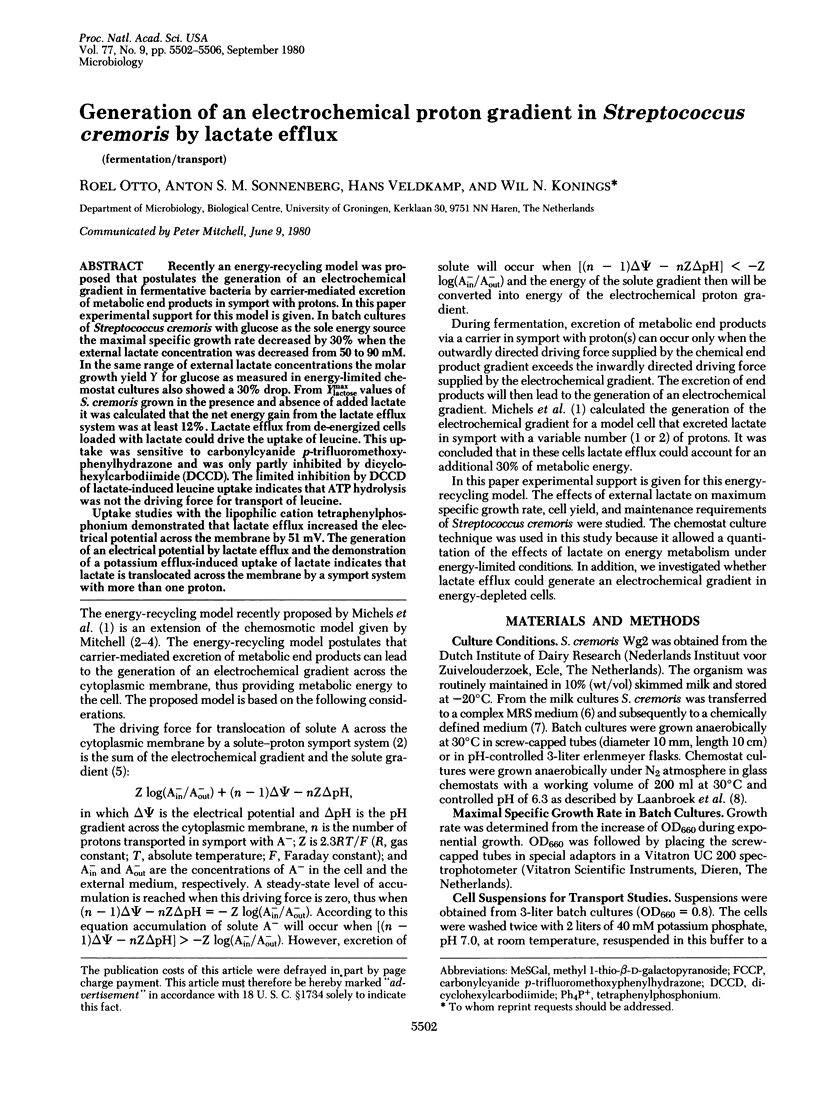
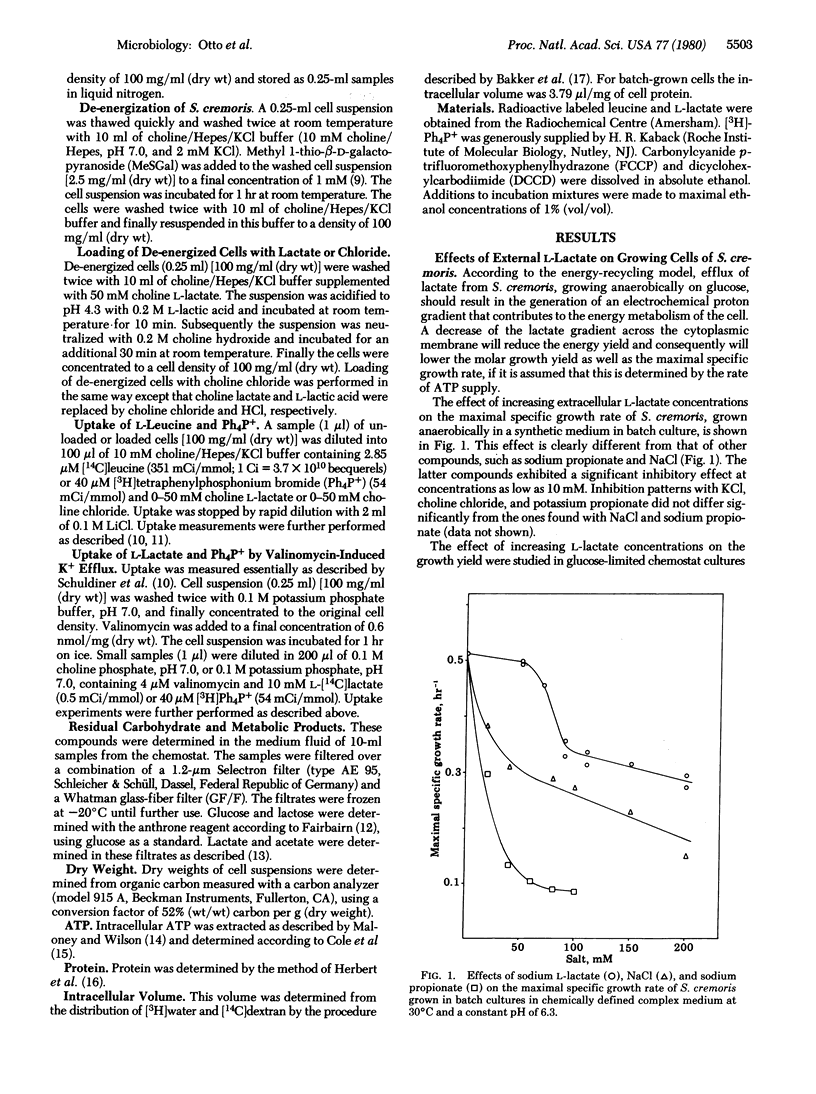
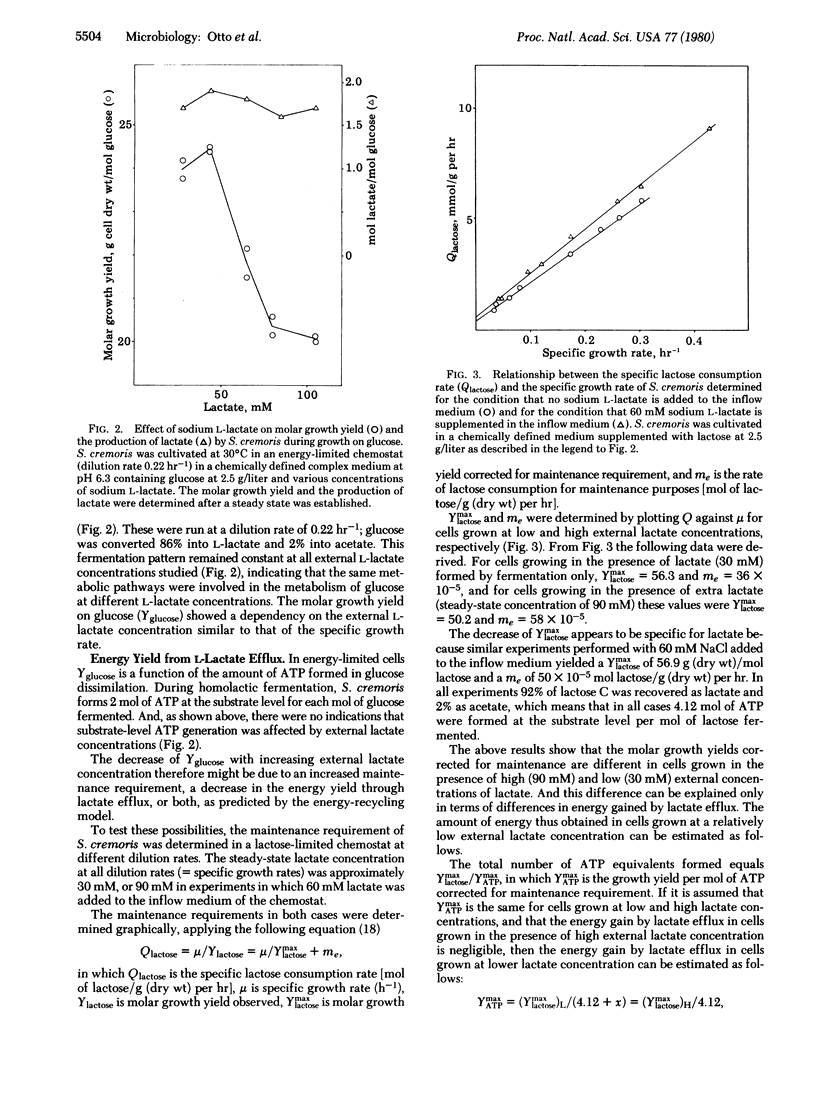
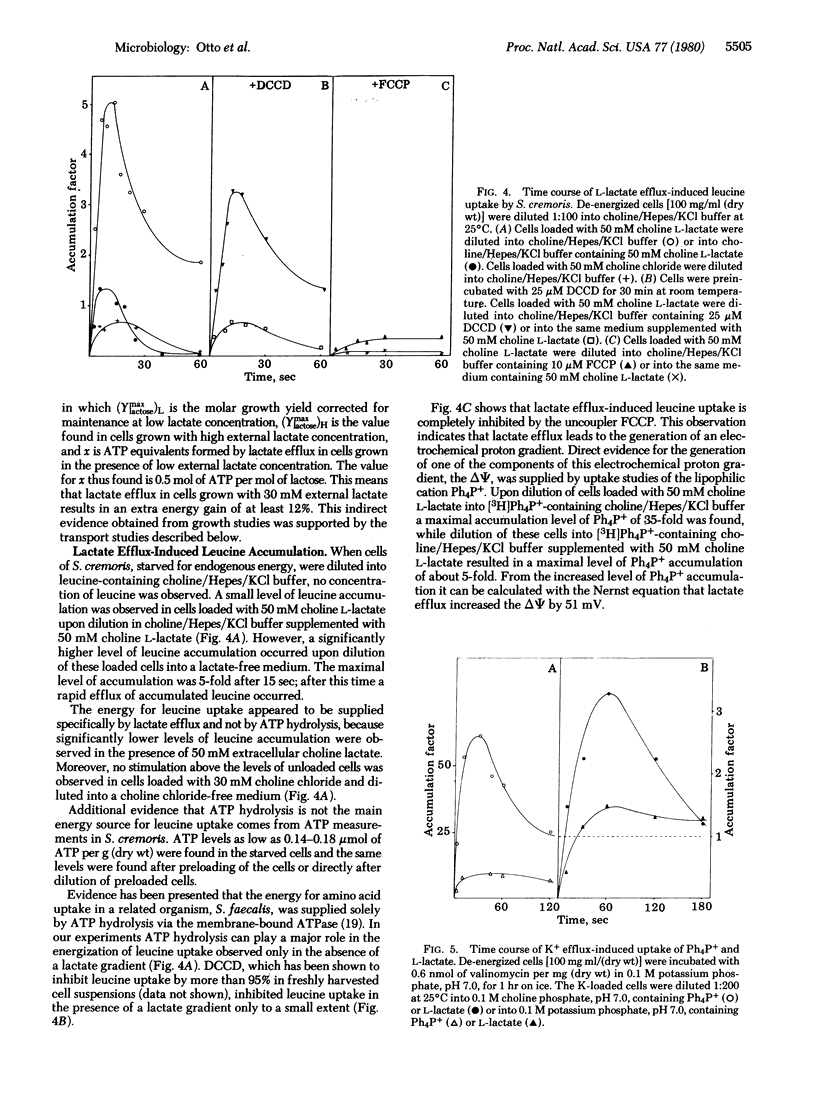
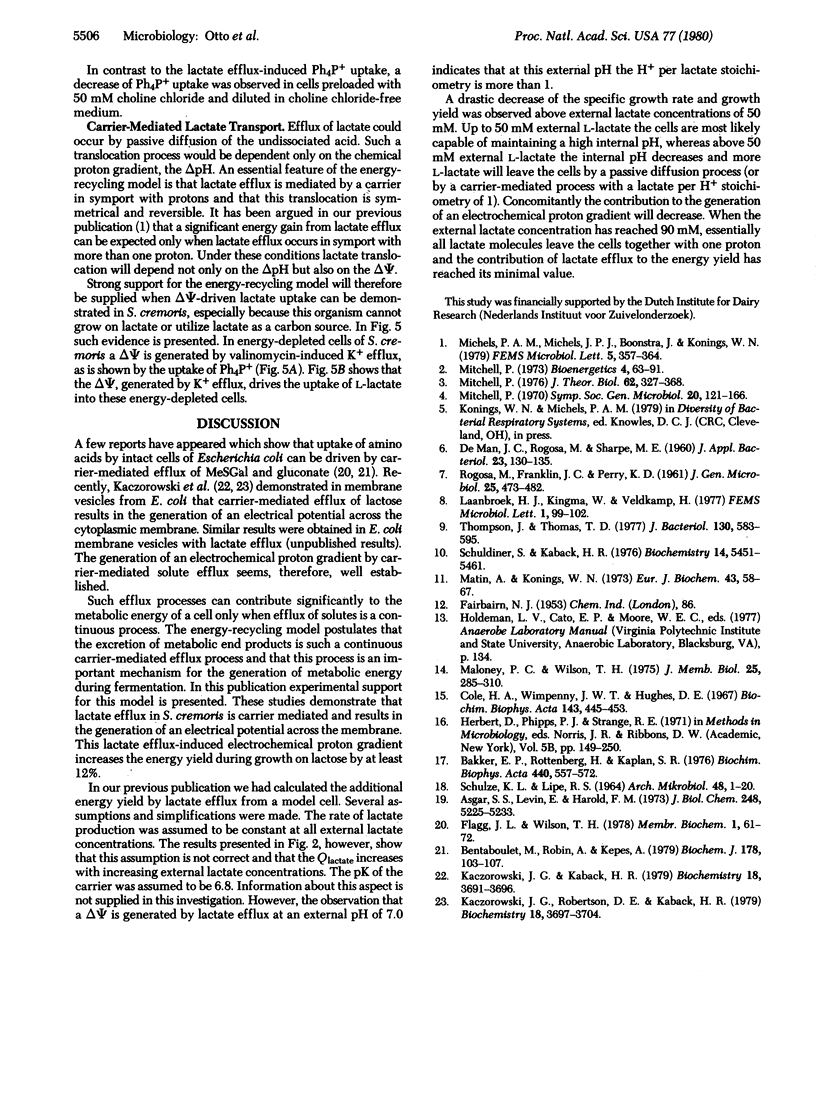
Selected References
These references are in PubMed. This may not be the complete list of references from this article.
- Asghar S. S., Levin E., Harold F. M. Accumulation of neutral amino acids by Streptococcus faecalis. Energy coupling by a proton-motive force. J Biol Chem. 1973 Aug 10;248(15):5225–5233. [PubMed] [Google Scholar]
- Bakker E. P., Rottenberg H., Caplan S. R. An estimation of the light-induced electrochemical potential difference of protons across the membrane of Halobacterium halobium. Biochim Biophys Acta. 1976 Sep 13;440(3):557–572. doi: 10.1016/0005-2728(76)90042-6. [DOI] [PubMed] [Google Scholar]
- Bentaboulet M., Robin A., Kepes A. Artificially induced active transport of amino acid driven by the efflux of a sugar via a heterologous transport system in de-energized Escherichia coli. Biochem J. 1979 Jan 15;178(1):103–107. doi: 10.1042/bj1780103. [DOI] [PMC free article] [PubMed] [Google Scholar]
- Cole H. A., Wimpenny J. W., Hughes D. E. The ATP pool in Escherichia coli. I. Measurement of the pool using modified luciferase assay. Biochim Biophys Acta. 1967;143(3):445–453. doi: 10.1016/0005-2728(67)90050-3. [DOI] [PubMed] [Google Scholar]
- Flagg J. L., Wilson T. H. A novel type of coupling between proline and galactoside transport in Escherichia coli. Membr Biochem. 1978;1(1-2):61–72. doi: 10.3109/09687687809064159. [DOI] [PubMed] [Google Scholar]
- Kaczorowski G. J., Kaback H. R. Mechanism of lactose translocation in membrane vesicles from Escherichia coli. 1. Effect of pH on efflux, exchange, and counterflow. Biochemistry. 1979 Aug 21;18(17):3691–3697. doi: 10.1021/bi00584a009. [DOI] [PubMed] [Google Scholar]
- Kaczorowski G. J., Robertson D. E., Kaback H. R. Mechanism of lactose translocation in membrane vesicles from Escherichia coli. 2. Effect of imposed delata psi, delta pH, and Delta mu H+. Biochemistry. 1979 Aug 21;18(17):3697–3704. doi: 10.1021/bi00584a010. [DOI] [PubMed] [Google Scholar]
- Maloney P. C., Wilson T. H. ATP synthesis driven by a protonmotive force in Streptococcus lactis. J Membr Biol. 1975;25(3-4):285–310. doi: 10.1007/BF01868580. [DOI] [PubMed] [Google Scholar]
- Matin A., Konings W. N. Transport of lactate and succinate by membrane vesicles of Escherichia coli, Bacillus subtilis and a pseudomonas species. Eur J Biochem. 1973 Apr 2;34(1):58–67. doi: 10.1111/j.1432-1033.1973.tb02728.x. [DOI] [PubMed] [Google Scholar]
- Mitchell P. Performance and conservation of osmotic work by proton-coupled solute porter systems. J Bioenerg. 1973 Jan;4(1):63–91. doi: 10.1007/BF01516051. [DOI] [PubMed] [Google Scholar]
- Mitchell P. Possible molecular mechanisms of the protonmotive function of cytochrome systems. J Theor Biol. 1976 Oct 21;62(2):327–367. doi: 10.1016/0022-5193(76)90124-7. [DOI] [PubMed] [Google Scholar]
- ROGOSA M., FRANKLIN J. G., PERRY K. D. Correlation of the vitamin requirements with cultural and biochemical characters of Lactobacillus spp. J Gen Microbiol. 1961 Jul;25:473–482. doi: 10.1099/00221287-25-3-473. [DOI] [PubMed] [Google Scholar]
- Schuldiner S., Kaback H. R. Membrane potential and active transport in membrane vesicles from Escherichia coli. Biochemistry. 1975 Dec 16;14(25):5451–5461. doi: 10.1021/bi00696a011. [DOI] [PubMed] [Google Scholar]
- Thompson J., Thomas T. D. Phosphoenolpyruvate and 2-phosphoglycerate: endogenous energy source(s) for sugar accumulation by starved cells of Streptococcus lactis. J Bacteriol. 1977 May;130(2):583–595. doi: 10.1128/jb.130.2.583-595.1977. [DOI] [PMC free article] [PubMed] [Google Scholar]