Abstract
In the present study, we have used single chicken blastoderms of defined early developmental stages, beginning with the prestreak stage, stage 1 (V. Hamburger and H. L. Hamilton, J. Morphol. 88:49-92, 1951), to analyze the onset of cardiac myogenesis by monitoring the appearance of selected cardiac muscle tissue-specific gene transcripts and the functional expression of the myocyte enhancer factor 2 (MEF-2) proteins. Using gene-specific oligonucleotide primers in reverse transcriptase PCR assay, we have demonstrated that the cardiac myosin light-chain 2 (MLC2) and alpha-actin gene transcripts appear as early as stage 5, i.e., immediately after the cardiogenic fate assignment at stage 4. Consistent with this observation is the developmental expression pattern of DNA-binding activity of BBF-1, a cardiac muscle-specific member of the MEF-2 protein family, which also begins at stage 5 prior to MEF-2. Differential expression of DNA-binding complexes is also observed with another AT-rich DNA sequence (CArG box) as probe, but the binding pattern with the ubiquitous TATA-binding proteins remains unchanged during the same developmental period. Thus, the cardiogenic commitment and differentiation of the precardiac mesoderm, as exemplified by the appearance of cardiac MEF-2, MLC2, and alpha-actin gene products, occur earlier than previously thought and appear to be closely linked. The onset of skeletal myogenic program follows that of the cardiogenic program with the appearance of skeletal MLC2 at stage 8. We also observed that mRNA for the MEF-2 family of proteins appears as early as stage 2 and that for CMD-1, the chicken counterpart of MyoD, appears at stage 5. The temporal separation of activation of cardiac and skeletal MLC2 genes, which appears immediately after the respective fate assignments, and those of cardiac MEF-2 and CMD-1, which occur before, are consistent with the established appearance of the myogenic programs and with the acquisition pattern of the two tissue-specific morphological characteristics in the early embryo. The preferential appearance of BBF-1 activity in precardiac moesderm, relative to that of MEF-2, indicates that these two protein factors are distinct members of the MEF-2 family and provides a compelling argument in support of the potential role of BBF-1 as a regulator of the cardiogenic cell lineage determination, while cardiac MEF-2 might be involved in maintenance of the cardiac differentiative state.
Full text
PDF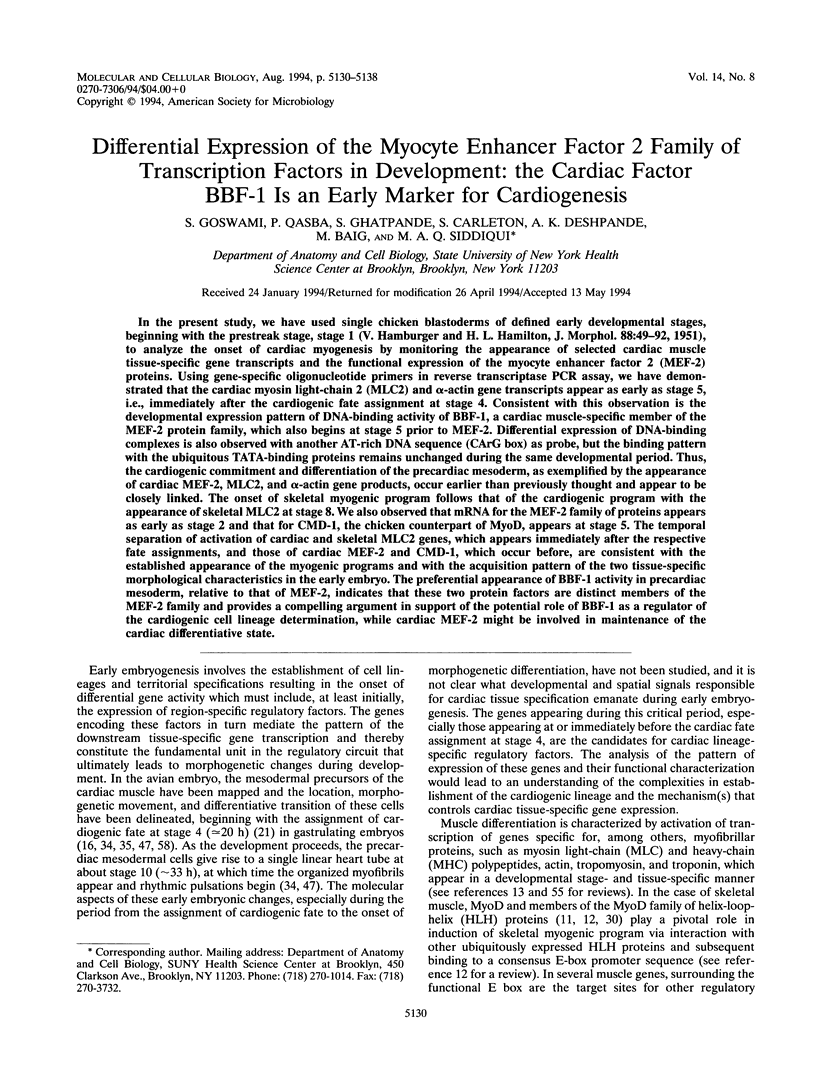
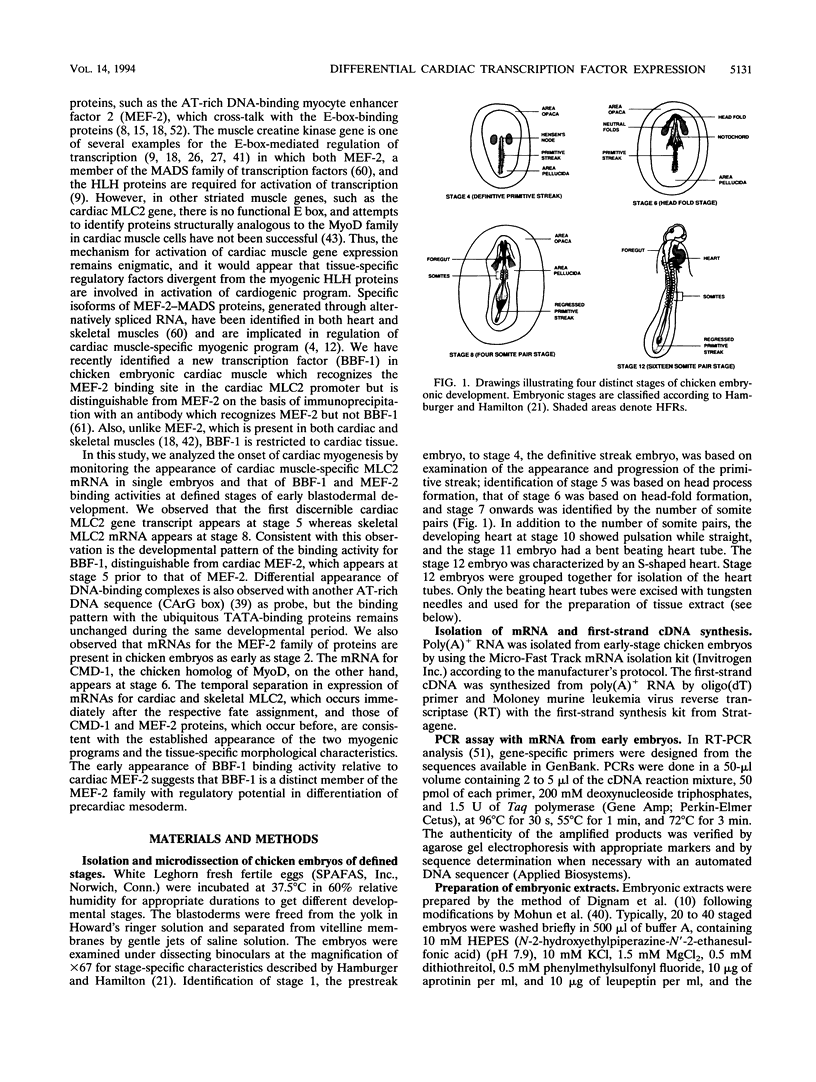
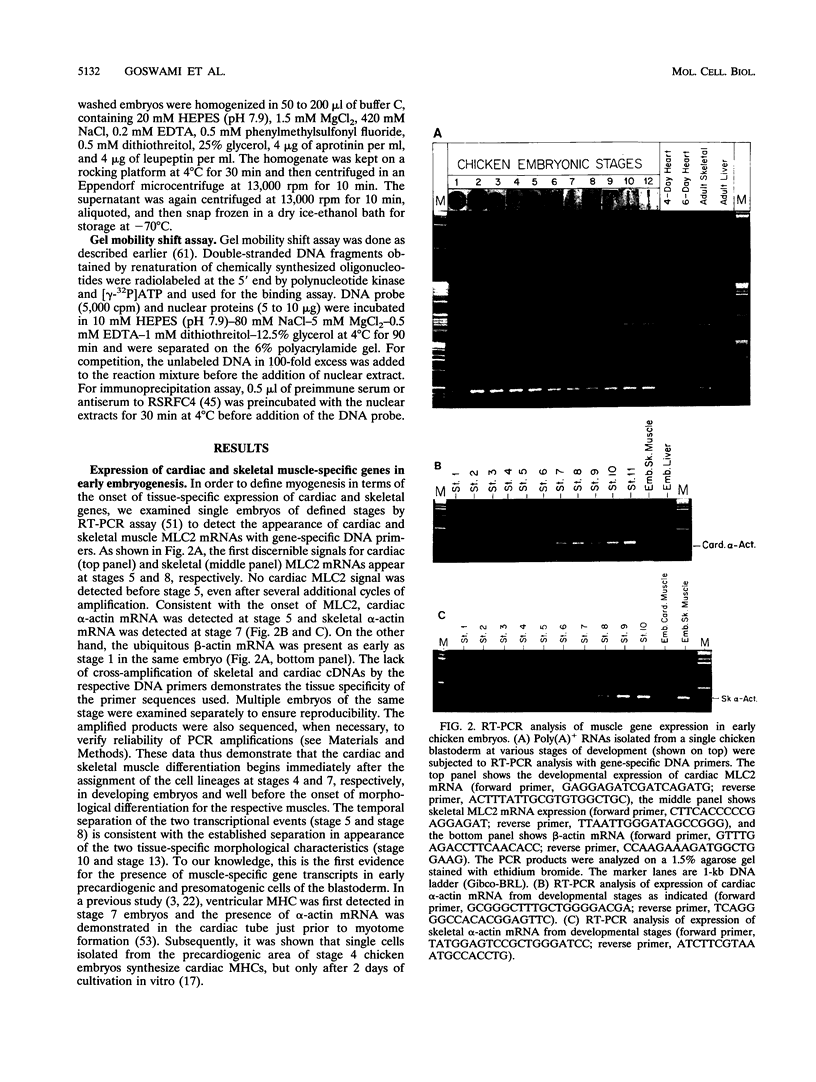
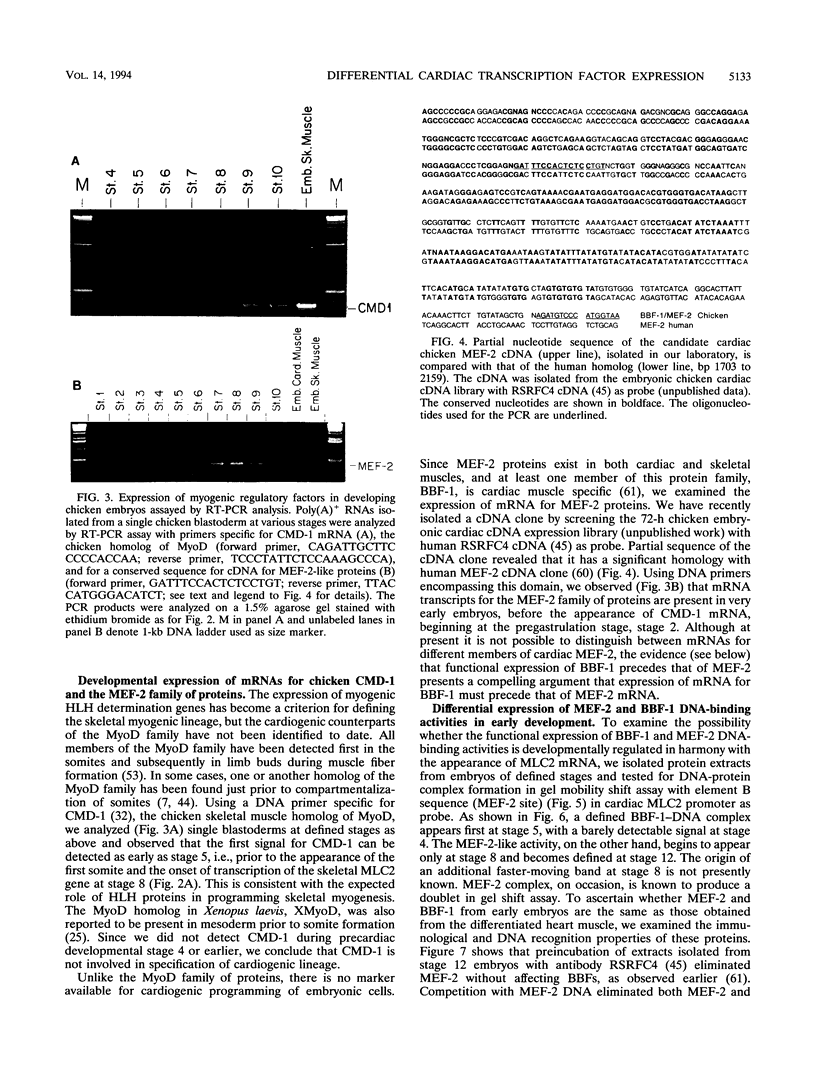
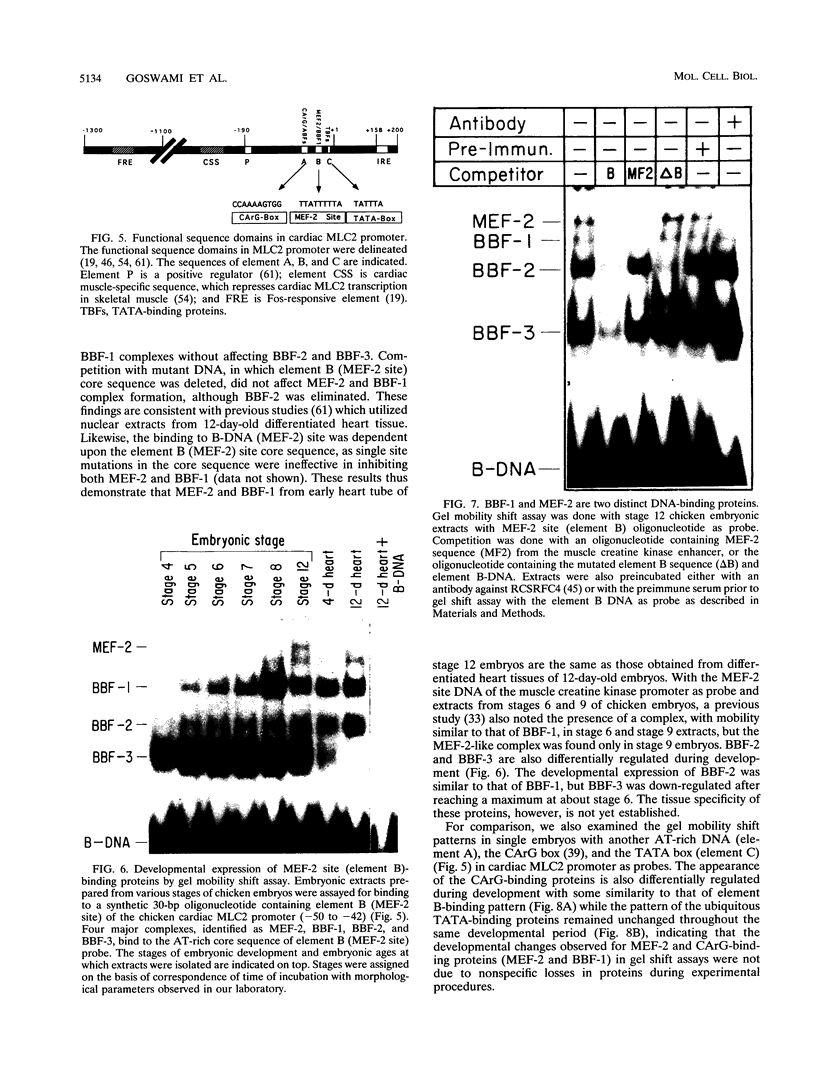
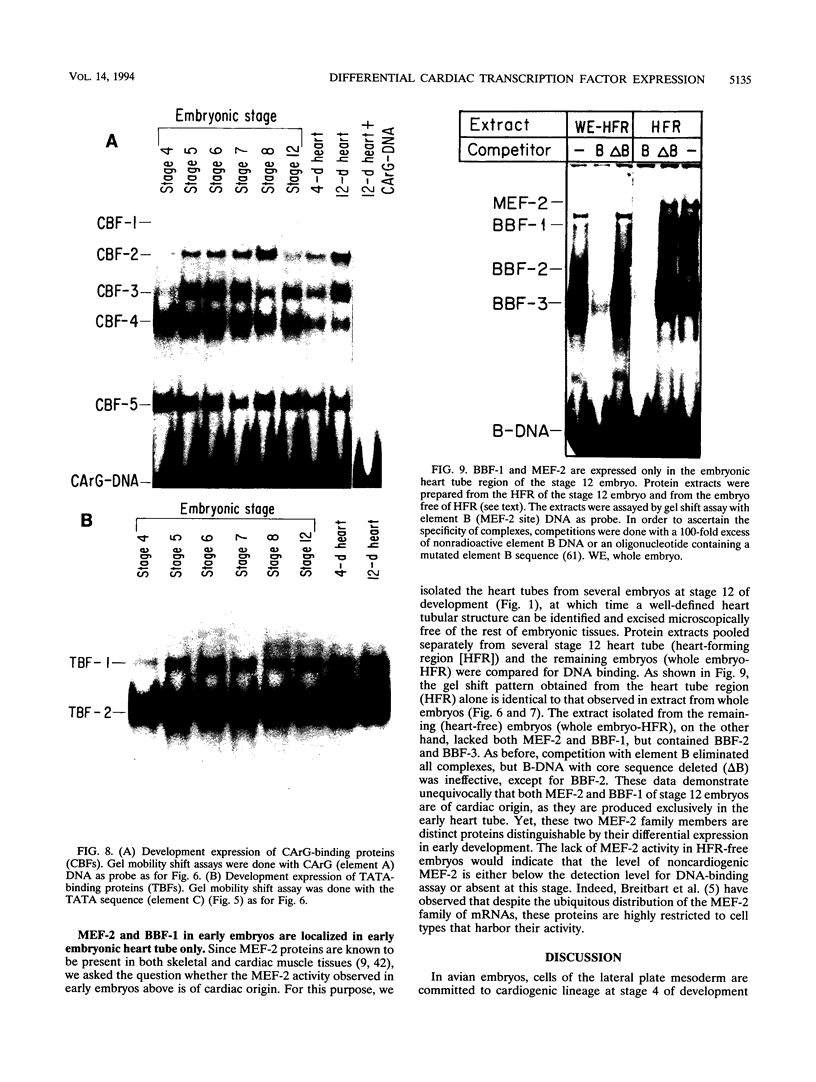
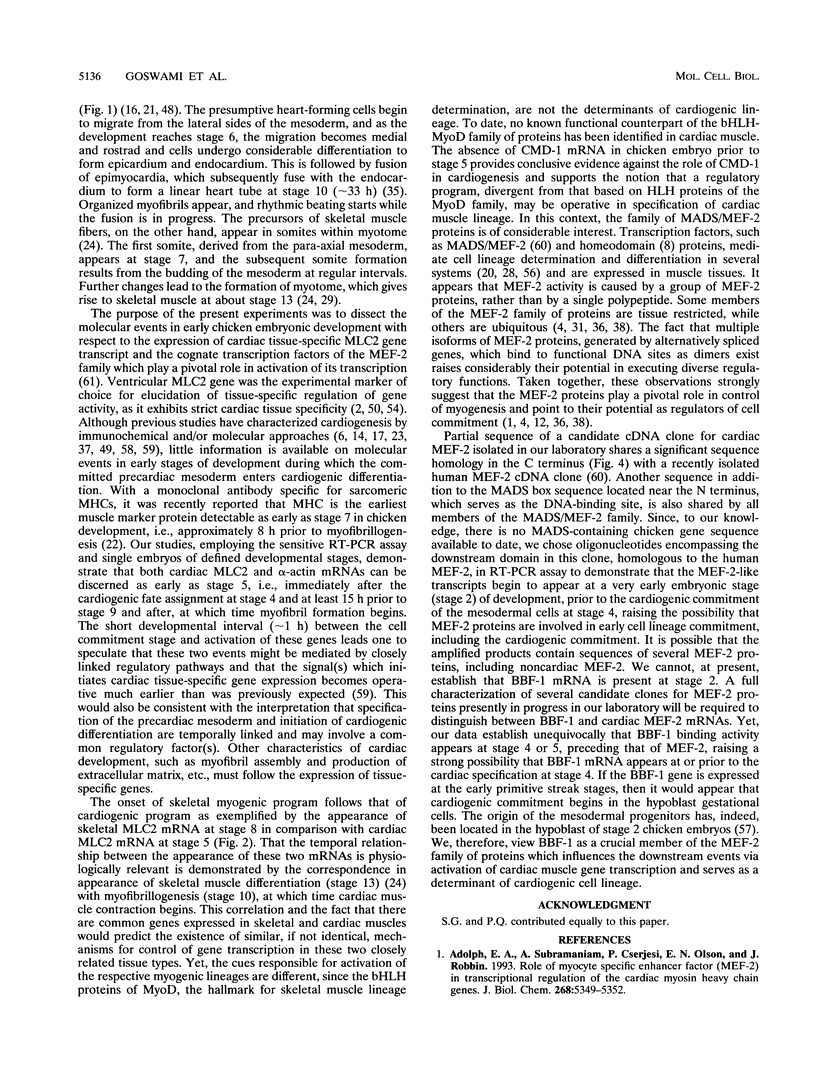
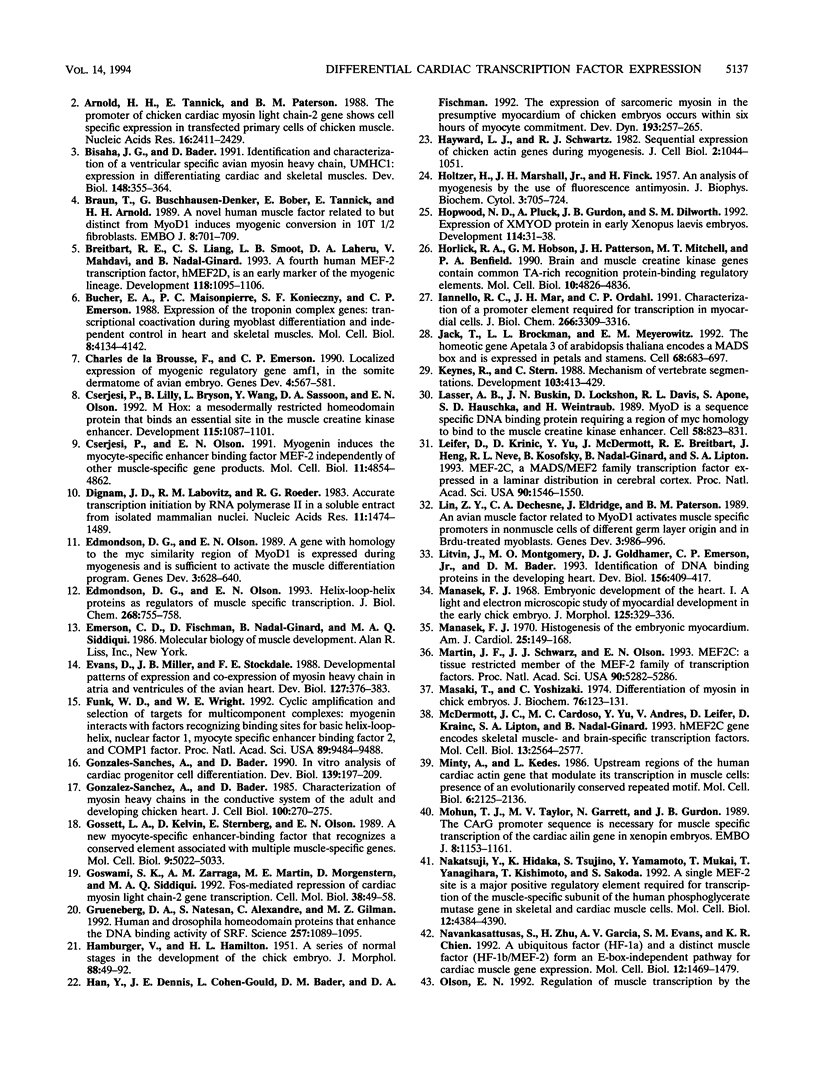
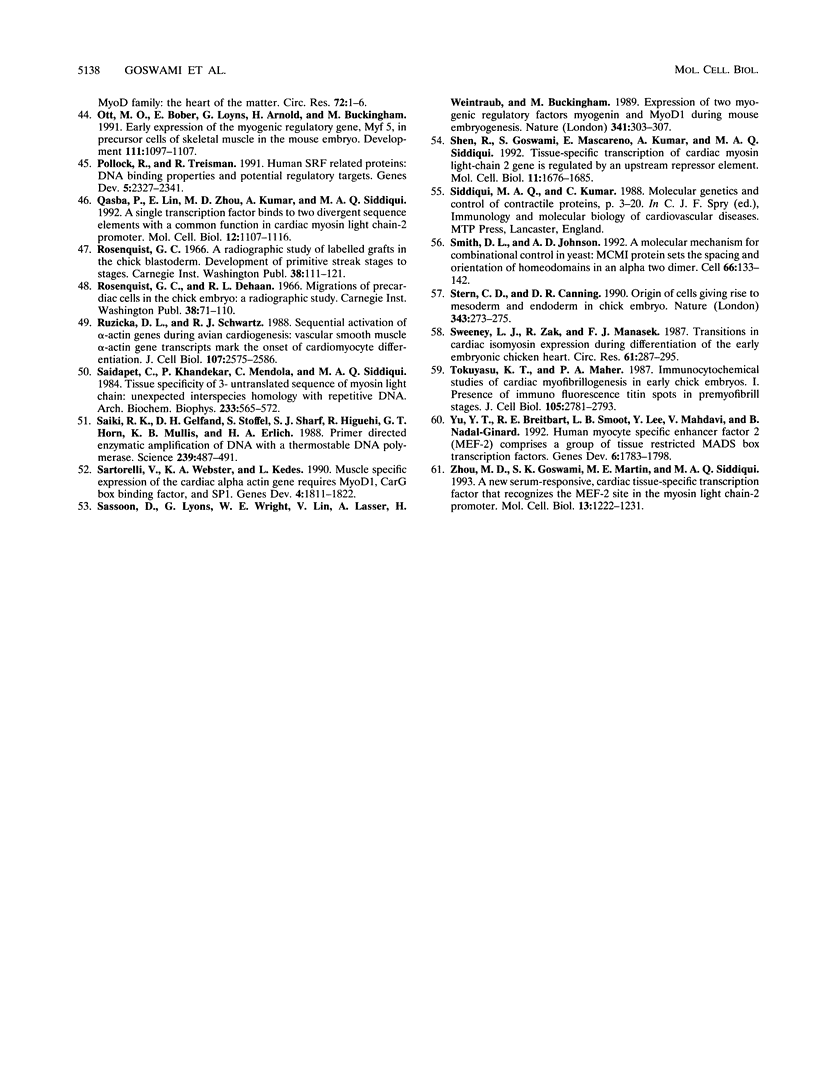
Images in this article
Selected References
These references are in PubMed. This may not be the complete list of references from this article.
- Adolph E. A., Subramaniam A., Cserjesi P., Olson E. N., Robbins J. Role of myocyte-specific enhancer-binding factor (MEF-2) in transcriptional regulation of the alpha-cardiac myosin heavy chain gene. J Biol Chem. 1993 Mar 15;268(8):5349–5352. [PubMed] [Google Scholar]
- Arnold H. H., Tannich E., Paterson B. M. The promoter of the chicken cardiac myosin light chain 2 gene shows cell-specific expression in transfected primary cultures of chicken muscle. Nucleic Acids Res. 1988 Mar 25;16(6):2411–2429. doi: 10.1093/nar/16.6.2411. [DOI] [PMC free article] [PubMed] [Google Scholar]
- Bisaha J. G., Bader D. Identification and characterization of a ventricular-specific avian myosin heavy chain, VMHC1: expression in differentiating cardiac and skeletal muscle. Dev Biol. 1991 Nov;148(1):355–364. doi: 10.1016/0012-1606(91)90343-2. [DOI] [PubMed] [Google Scholar]
- Braun T., Buschhausen-Denker G., Bober E., Tannich E., Arnold H. H. A novel human muscle factor related to but distinct from MyoD1 induces myogenic conversion in 10T1/2 fibroblasts. EMBO J. 1989 Mar;8(3):701–709. doi: 10.1002/j.1460-2075.1989.tb03429.x. [DOI] [PMC free article] [PubMed] [Google Scholar]
- Breitbart R. E., Liang C. S., Smoot L. B., Laheru D. A., Mahdavi V., Nadal-Ginard B. A fourth human MEF2 transcription factor, hMEF2D, is an early marker of the myogenic lineage. Development. 1993 Aug;118(4):1095–1106. doi: 10.1242/dev.118.4.1095. [DOI] [PubMed] [Google Scholar]
- Bucher E. A., Maisonpierre P. C., Konieczny S. F., Emerson C. P., Jr Expression of the troponin complex genes: transcriptional coactivation during myoblast differentiation and independent control in heart and skeletal muscles. Mol Cell Biol. 1988 Oct;8(10):4134–4142. doi: 10.1128/mcb.8.10.4134. [DOI] [PMC free article] [PubMed] [Google Scholar]
- Cserjesi P., Lilly B., Bryson L., Wang Y., Sassoon D. A., Olson E. N. MHox: a mesodermally restricted homeodomain protein that binds an essential site in the muscle creatine kinase enhancer. Development. 1992 Aug;115(4):1087–1101. doi: 10.1242/dev.115.4.1087. [DOI] [PubMed] [Google Scholar]
- Cserjesi P., Olson E. N. Myogenin induces the myocyte-specific enhancer binding factor MEF-2 independently of other muscle-specific gene products. Mol Cell Biol. 1991 Oct;11(10):4854–4862. doi: 10.1128/mcb.11.10.4854. [DOI] [PMC free article] [PubMed] [Google Scholar]
- Dignam J. D., Lebovitz R. M., Roeder R. G. Accurate transcription initiation by RNA polymerase II in a soluble extract from isolated mammalian nuclei. Nucleic Acids Res. 1983 Mar 11;11(5):1475–1489. doi: 10.1093/nar/11.5.1475. [DOI] [PMC free article] [PubMed] [Google Scholar]
- Edmondson D. G., Olson E. N. A gene with homology to the myc similarity region of MyoD1 is expressed during myogenesis and is sufficient to activate the muscle differentiation program. Genes Dev. 1989 May;3(5):628–640. doi: 10.1101/gad.3.5.628. [DOI] [PubMed] [Google Scholar]
- Edmondson D. G., Olson E. N. Helix-loop-helix proteins as regulators of muscle-specific transcription. J Biol Chem. 1993 Jan 15;268(2):755–758. [PubMed] [Google Scholar]
- Evans D., Miller J. B., Stockdale F. E. Developmental patterns of expression and coexpression of myosin heavy chains in atria and ventricles of the avian heart. Dev Biol. 1988 Jun;127(2):376–383. doi: 10.1016/0012-1606(88)90324-7. [DOI] [PubMed] [Google Scholar]
- Funk W. D., Wright W. E. Cyclic amplification and selection of targets for multicomponent complexes: myogenin interacts with factors recognizing binding sites for basic helix-loop-helix, nuclear factor 1, myocyte-specific enhancer-binding factor 2, and COMP1 factor. Proc Natl Acad Sci U S A. 1992 Oct 15;89(20):9484–9488. doi: 10.1073/pnas.89.20.9484. [DOI] [PMC free article] [PubMed] [Google Scholar]
- Gonzalez-Sanchez A., Bader D. In vitro analysis of cardiac progenitor cell differentiation. Dev Biol. 1990 May;139(1):197–209. doi: 10.1016/0012-1606(90)90288-t. [DOI] [PubMed] [Google Scholar]
- González-Sánchez A., Bader D. Characterization of a myosin heavy chain in the conductive system of the adult and developing chicken heart. J Cell Biol. 1985 Jan;100(1):270–275. doi: 10.1083/jcb.100.1.270. [DOI] [PMC free article] [PubMed] [Google Scholar]
- Gossett L. A., Kelvin D. J., Sternberg E. A., Olson E. N. A new myocyte-specific enhancer-binding factor that recognizes a conserved element associated with multiple muscle-specific genes. Mol Cell Biol. 1989 Nov;9(11):5022–5033. doi: 10.1128/mcb.9.11.5022. [DOI] [PMC free article] [PubMed] [Google Scholar]
- Goswami S. K., Zarraga A. M., Martin M. E., Morgenstern D., Siddiqui M. A. fos-mediated repression of cardiac myosin light chain-2 gene transcription. Cell Mol Biol. 1992 Feb;38(1):49–58. [PubMed] [Google Scholar]
- Grueneberg D. A., Natesan S., Alexandre C., Gilman M. Z. Human and Drosophila homeodomain proteins that enhance the DNA-binding activity of serum response factor. Science. 1992 Aug 21;257(5073):1089–1095. doi: 10.1126/science.257.5073.1089. [DOI] [PubMed] [Google Scholar]
- HOLTZER H., MARSHALL J. M., Jr, FINCK H. An analysis of myogenesis by the use of fluorescent antimyosin. J Biophys Biochem Cytol. 1957 Sep 25;3(5):705–724. doi: 10.1083/jcb.3.5.705. [DOI] [PMC free article] [PubMed] [Google Scholar]
- Han Y., Dennis J. E., Cohen-Gould L., Bader D. M., Fischman D. A. Expression of sarcomeric myosin in the presumptive myocardium of chicken embryos occurs within six hours of myocyte commitment. Dev Dyn. 1992 Mar;193(3):257–265. doi: 10.1002/aja.1001930306. [DOI] [PubMed] [Google Scholar]
- Hopwood N. D., Pluck A., Gurdon J. B., Dilworth S. M. Expression of XMyoD protein in early Xenopus laevis embryos. Development. 1992 Jan;114(1):31–38. doi: 10.1242/dev.114.1.31. [DOI] [PubMed] [Google Scholar]
- Horlick R. A., Hobson G. M., Patterson J. H., Mitchell M. T., Benfield P. A. Brain and muscle creatine kinase genes contain common TA-rich recognition protein-binding regulatory elements. Mol Cell Biol. 1990 Sep;10(9):4826–4836. doi: 10.1128/mcb.10.9.4826. [DOI] [PMC free article] [PubMed] [Google Scholar]
- Iannello R. C., Mar J. H., Ordahl C. P. Characterization of a promoter element required for transcription in myocardial cells. J Biol Chem. 1991 Feb 15;266(5):3309–3316. [PubMed] [Google Scholar]
- Jack T., Brockman L. L., Meyerowitz E. M. The homeotic gene APETALA3 of Arabidopsis thaliana encodes a MADS box and is expressed in petals and stamens. Cell. 1992 Feb 21;68(4):683–697. doi: 10.1016/0092-8674(92)90144-2. [DOI] [PubMed] [Google Scholar]
- Keynes R. J., Stern C. D. Mechanisms of vertebrate segmentation. Development. 1988 Jul;103(3):413–429. doi: 10.1242/dev.103.3.413. [DOI] [PubMed] [Google Scholar]
- Lassar A. B., Buskin J. N., Lockshon D., Davis R. L., Apone S., Hauschka S. D., Weintraub H. MyoD is a sequence-specific DNA binding protein requiring a region of myc homology to bind to the muscle creatine kinase enhancer. Cell. 1989 Sep 8;58(5):823–831. doi: 10.1016/0092-8674(89)90935-5. [DOI] [PubMed] [Google Scholar]
- Leifer D., Krainc D., Yu Y. T., McDermott J., Breitbart R. E., Heng J., Neve R. L., Kosofsky B., Nadal-Ginard B., Lipton S. A. MEF2C, a MADS/MEF2-family transcription factor expressed in a laminar distribution in cerebral cortex. Proc Natl Acad Sci U S A. 1993 Feb 15;90(4):1546–1550. doi: 10.1073/pnas.90.4.1546. [DOI] [PMC free article] [PubMed] [Google Scholar]
- Lin Z. Y., Dechesne C. A., Eldridge J., Paterson B. M. An avian muscle factor related to MyoD1 activates muscle-specific promoters in nonmuscle cells of different germ-layer origin and in BrdU-treated myoblasts. Genes Dev. 1989 Jul;3(7):986–996. doi: 10.1101/gad.3.7.986. [DOI] [PubMed] [Google Scholar]
- Litvin J., Montgomery M. O., Goldhamer D. J., Emerson C. P., Jr, Bader D. M. Identification of DNA-binding protein(s) in the developing heart. Dev Biol. 1993 Apr;156(2):409–417. doi: 10.1006/dbio.1993.1088. [DOI] [PubMed] [Google Scholar]
- Manasek F. J. Embryonic development of the heart. I. A light and electron microscopic study of myocardial development in the early chick embryo. J Morphol. 1968 Jul;125(3):329–365. doi: 10.1002/jmor.1051250306. [DOI] [PubMed] [Google Scholar]
- Manasek F. J. Histogenesis of the embryonic myocardium. Am J Cardiol. 1970 Feb;25(2):149–168. doi: 10.1016/0002-9149(70)90576-x. [DOI] [PubMed] [Google Scholar]
- Martin J. F., Schwarz J. J., Olson E. N. Myocyte enhancer factor (MEF) 2C: a tissue-restricted member of the MEF-2 family of transcription factors. Proc Natl Acad Sci U S A. 1993 Jun 1;90(11):5282–5286. doi: 10.1073/pnas.90.11.5282. [DOI] [PMC free article] [PubMed] [Google Scholar]
- Masaki T., Yoshizaki C. Differentiation of myosin in chick embryos. J Biochem. 1974 Jul;76(1):123–131. doi: 10.1093/oxfordjournals.jbchem.a130536. [DOI] [PubMed] [Google Scholar]
- McDermott J. C., Cardoso M. C., Yu Y. T., Andres V., Leifer D., Krainc D., Lipton S. A., Nadal-Ginard B. hMEF2C gene encodes skeletal muscle- and brain-specific transcription factors. Mol Cell Biol. 1993 Apr;13(4):2564–2577. doi: 10.1128/mcb.13.4.2564. [DOI] [PMC free article] [PubMed] [Google Scholar]
- Minty A., Kedes L. Upstream regions of the human cardiac actin gene that modulate its transcription in muscle cells: presence of an evolutionarily conserved repeated motif. Mol Cell Biol. 1986 Jun;6(6):2125–2136. doi: 10.1128/mcb.6.6.2125. [DOI] [PMC free article] [PubMed] [Google Scholar]
- Mohun T. J., Taylor M. V., Garrett N., Gurdon J. B. The CArG promoter sequence is necessary for muscle-specific transcription of the cardiac actin gene in Xenopus embryos. EMBO J. 1989 Apr;8(4):1153–1161. doi: 10.1002/j.1460-2075.1989.tb03486.x. [DOI] [PMC free article] [PubMed] [Google Scholar]
- Nakatsuji Y., Hidaka K., Tsujino S., Yamamoto Y., Mukai T., Yanagihara T., Kishimoto T., Sakoda S. A single MEF-2 site is a major positive regulatory element required for transcription of the muscle-specific subunit of the human phosphoglycerate mutase gene in skeletal and cardiac muscle cells. Mol Cell Biol. 1992 Oct;12(10):4384–4390. doi: 10.1128/mcb.12.10.4384. [DOI] [PMC free article] [PubMed] [Google Scholar]
- Navankasattusas S., Zhu H., Garcia A. V., Evans S. M., Chien K. R. A ubiquitous factor (HF-1a) and a distinct muscle factor (HF-1b/MEF-2) form an E-box-independent pathway for cardiac muscle gene expression. Mol Cell Biol. 1992 Apr;12(4):1469–1479. doi: 10.1128/mcb.12.4.1469. [DOI] [PMC free article] [PubMed] [Google Scholar]
- Ott M. O., Bober E., Lyons G., Arnold H., Buckingham M. Early expression of the myogenic regulatory gene, myf-5, in precursor cells of skeletal muscle in the mouse embryo. Development. 1991 Apr;111(4):1097–1107. doi: 10.1242/dev.111.4.1097. [DOI] [PubMed] [Google Scholar]
- Pollock R., Treisman R. Human SRF-related proteins: DNA-binding properties and potential regulatory targets. Genes Dev. 1991 Dec;5(12A):2327–2341. doi: 10.1101/gad.5.12a.2327. [DOI] [PubMed] [Google Scholar]
- Qasba P., Lin E., Zhou M. D., Kumar A., Siddiqui M. A. A single transcription factor binds to two divergent sequence elements with a common function in cardiac myosin light chain-2 promoter. Mol Cell Biol. 1992 Mar;12(3):1107–1116. doi: 10.1128/mcb.12.3.1107. [DOI] [PMC free article] [PubMed] [Google Scholar]
- Ruzicka D. L., Schwartz R. J. Sequential activation of alpha-actin genes during avian cardiogenesis: vascular smooth muscle alpha-actin gene transcripts mark the onset of cardiomyocyte differentiation. J Cell Biol. 1988 Dec;107(6 Pt 2):2575–2586. doi: 10.1083/jcb.107.6.2575. [DOI] [PMC free article] [PubMed] [Google Scholar]
- Saidapet C., Khandekar P., Mendola C., Siddiqui M. A. Tissue specificity of 3'-untranslated sequence of myosin light chain gene: unexpected interspecies homology with repetitive DNA. Arch Biochem Biophys. 1984 Sep;233(2):565–572. doi: 10.1016/0003-9861(84)90480-6. [DOI] [PubMed] [Google Scholar]
- Saiki R. K., Gelfand D. H., Stoffel S., Scharf S. J., Higuchi R., Horn G. T., Mullis K. B., Erlich H. A. Primer-directed enzymatic amplification of DNA with a thermostable DNA polymerase. Science. 1988 Jan 29;239(4839):487–491. doi: 10.1126/science.2448875. [DOI] [PubMed] [Google Scholar]
- Sartorelli V., Webster K. A., Kedes L. Muscle-specific expression of the cardiac alpha-actin gene requires MyoD1, CArG-box binding factor, and Sp1. Genes Dev. 1990 Oct;4(10):1811–1822. doi: 10.1101/gad.4.10.1811. [DOI] [PubMed] [Google Scholar]
- Sassoon D., Lyons G., Wright W. E., Lin V., Lassar A., Weintraub H., Buckingham M. Expression of two myogenic regulatory factors myogenin and MyoD1 during mouse embryogenesis. Nature. 1989 Sep 28;341(6240):303–307. doi: 10.1038/341303a0. [DOI] [PubMed] [Google Scholar]
- Shen R. A., Goswami S. K., Mascareno E., Kumar A., Siddiqui M. A. Tissue-specific transcription of the cardiac myosin light-chain 2 gene is regulated by an upstream repressor element. Mol Cell Biol. 1991 Mar;11(3):1676–1685. doi: 10.1128/mcb.11.3.1676. [DOI] [PMC free article] [PubMed] [Google Scholar]
- Smith D. L., Johnson A. D. A molecular mechanism for combinatorial control in yeast: MCM1 protein sets the spacing and orientation of the homeodomains of an alpha 2 dimer. Cell. 1992 Jan 10;68(1):133–142. doi: 10.1016/0092-8674(92)90212-u. [DOI] [PubMed] [Google Scholar]
- Stern C. D., Canning D. R. Origin of cells giving rise to mesoderm and endoderm in chick embryo. Nature. 1990 Jan 18;343(6255):273–275. doi: 10.1038/343273a0. [DOI] [PubMed] [Google Scholar]
- Sweeney L. J., Zak R., Manasek F. J. Transitions in cardiac isomyosin expression during differentiation of the embryonic chick heart. Circ Res. 1987 Aug;61(2):287–295. doi: 10.1161/01.res.61.2.287. [DOI] [PubMed] [Google Scholar]
- Tokuyasu K. T., Maher P. A. Immunocytochemical studies of cardiac myofibrillogenesis in early chick embryos. I. Presence of immunofluorescent titin spots in premyofibril stages. J Cell Biol. 1987 Dec;105(6 Pt 1):2781–2793. doi: 10.1083/jcb.105.6.2781. [DOI] [PMC free article] [PubMed] [Google Scholar]
- Yu Y. T., Breitbart R. E., Smoot L. B., Lee Y., Mahdavi V., Nadal-Ginard B. Human myocyte-specific enhancer factor 2 comprises a group of tissue-restricted MADS box transcription factors. Genes Dev. 1992 Sep;6(9):1783–1798. doi: 10.1101/gad.6.9.1783. [DOI] [PubMed] [Google Scholar]
- Zhou M. D., Goswami S. K., Martin M. E., Siddiqui M. A. A new serum-responsive, cardiac tissue-specific transcription factor that recognizes the MEF-2 site in the myosin light chain-2 promoter. Mol Cell Biol. 1993 Feb;13(2):1222–1231. doi: 10.1128/mcb.13.2.1222. [DOI] [PMC free article] [PubMed] [Google Scholar]
- de la Brousse F. C., Emerson C. P., Jr Localized expression of a myogenic regulatory gene, qmf1, in the somite dermatome of avian embryos. Genes Dev. 1990 Apr;4(4):567–581. doi: 10.1101/gad.4.4.567. [DOI] [PubMed] [Google Scholar]