Abstract
Throughout the purification of the mdm-2 or mdm-2-p53 protein complexes, a protein with a molecular weight of 34,000 was observed to copurify with these proteins. Several monoclonal antibodies directed against distinct epitopes in the mdm-2 or p53 protein coimmunoprecipitated this 34,000-molecular-weight protein, which did not react to p53 or mdm-2 polyclonal antisera in a Western immunoblot. The N-terminal amino acid sequence of this 34,000-molecular-weight protein demonstrated that the first 40 amino acids were identical to the ribosomal L5 protein, found in the large rRNA subunit and bound to 5S RNA. Partial peptide maps of the authentic L5 protein and the 34,000-molecular-weight protein were identical. mdm-2-L5 and mdm-2-L5-p53 complexes were shown to bind 5S RNA specifically, presumably through the known specificity of L5 protein for 5S RNA. In 5S RNA-L5-mdm-2-p53 ribonucleoprotein complexes, it was also possible to detect the 5.8S RNA which has been suggested to be covalently linked to a percentage of the p53 protein in a cell. These experiments have identified a unique ribonucleoprotein complex composed of 5S RNA, L5 protein, mdm-2 proteins, p53 protein, and possibly the 5.8S RNA. While the function of such a ribonucleoprotein complex is not yet clear, the identity of its component parts suggests a role for these proteins and RNA species in ribosomal biogenesis, ribosomal transport from the nucleus to the cytoplasm, or translational regulation in the cell.
Full text
PDF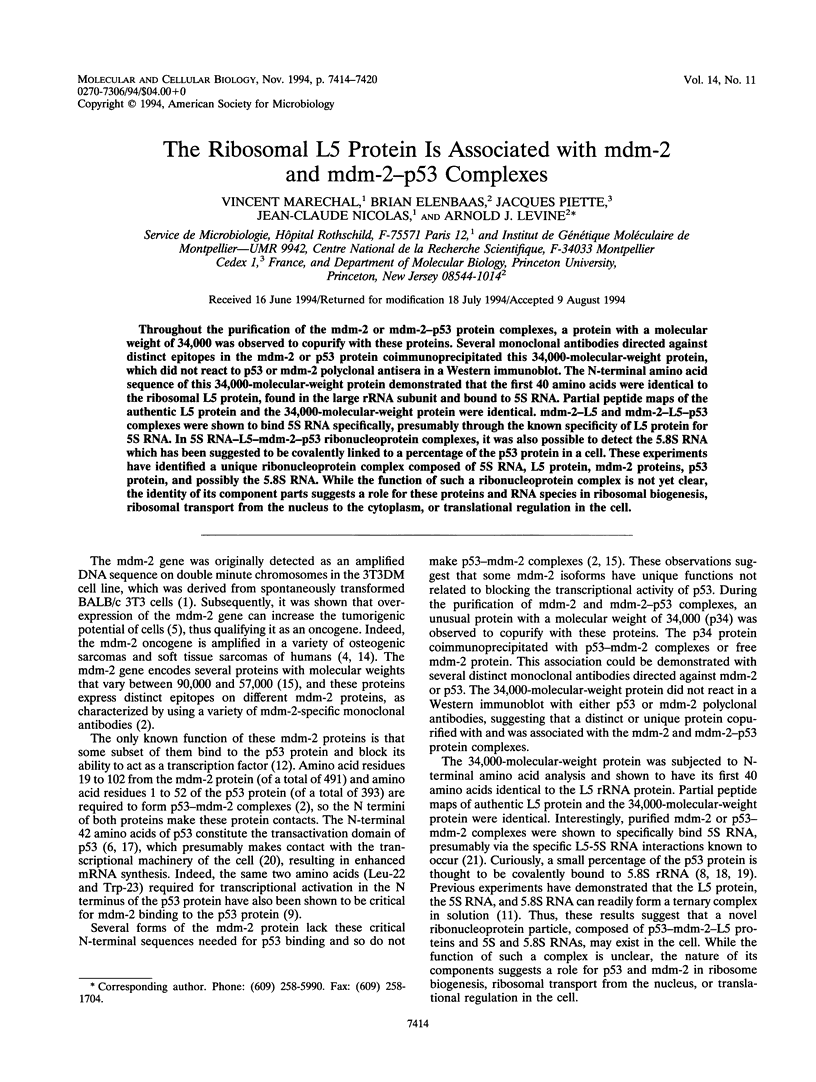
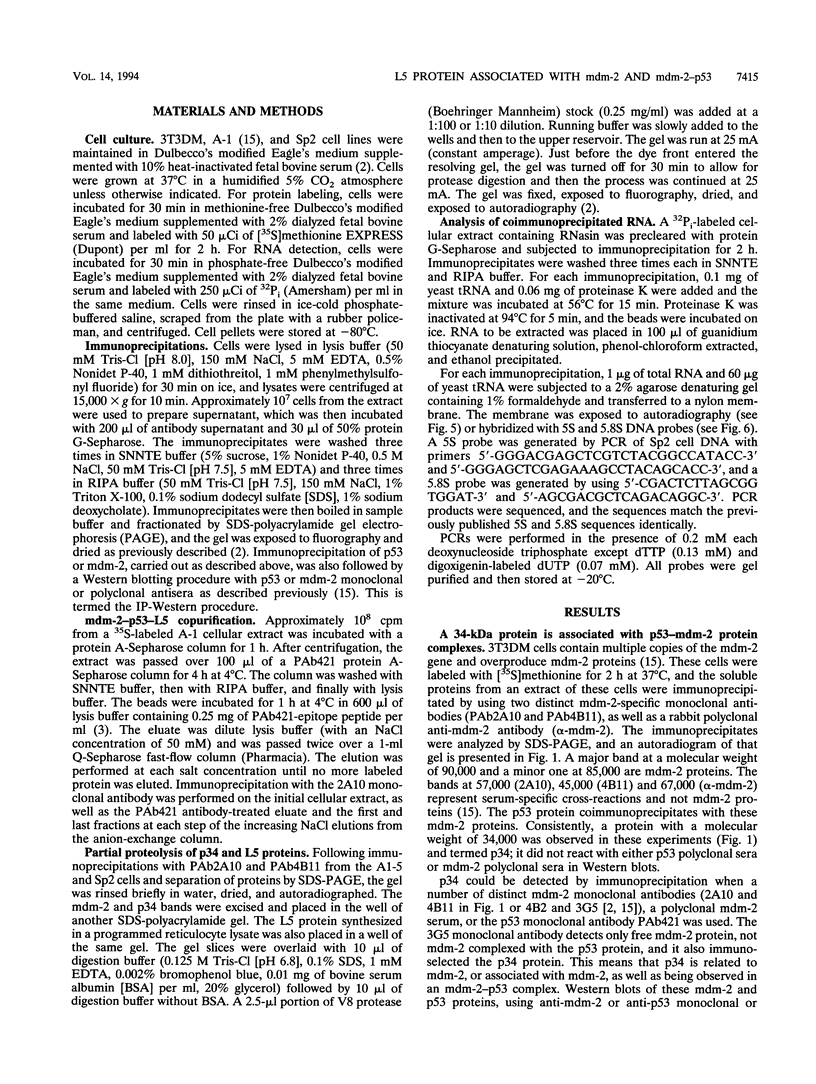
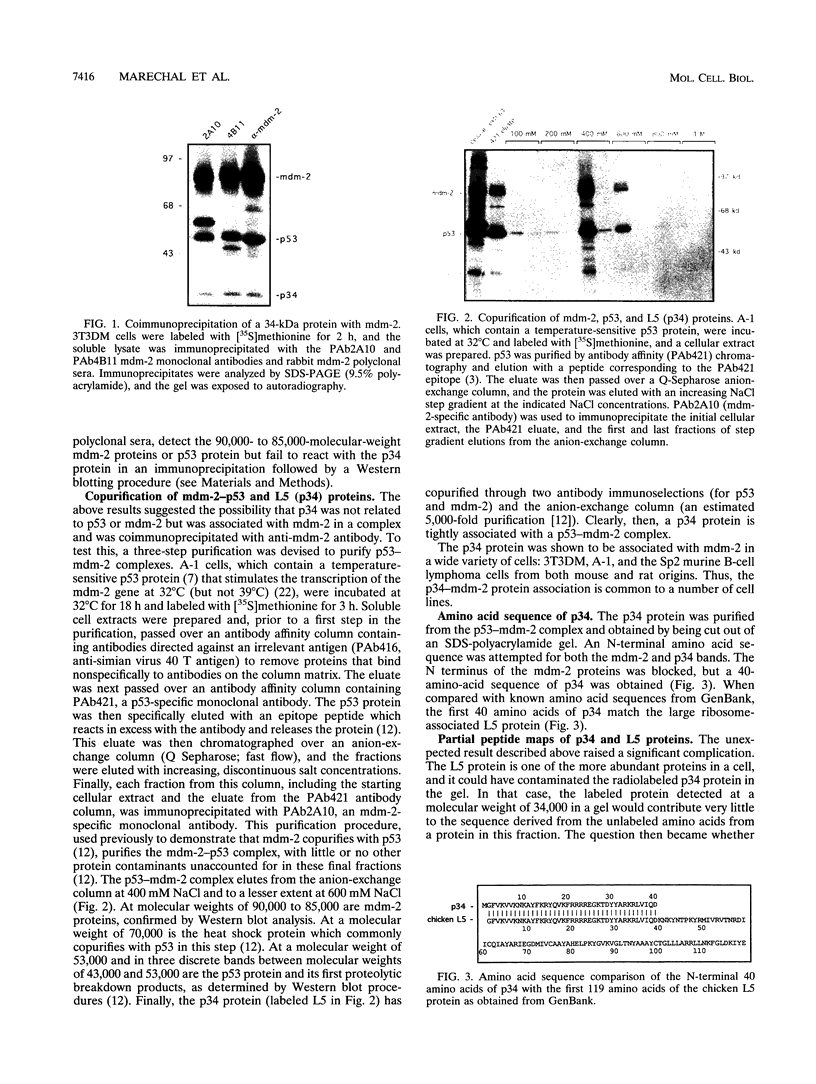
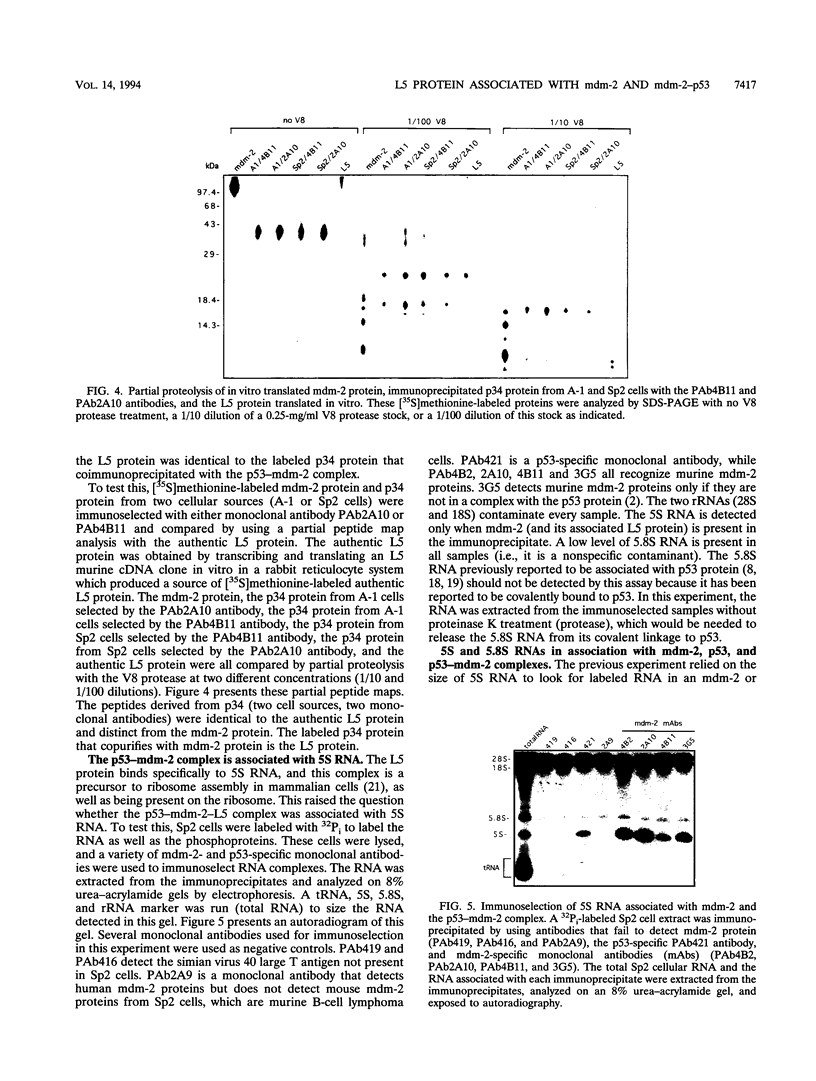
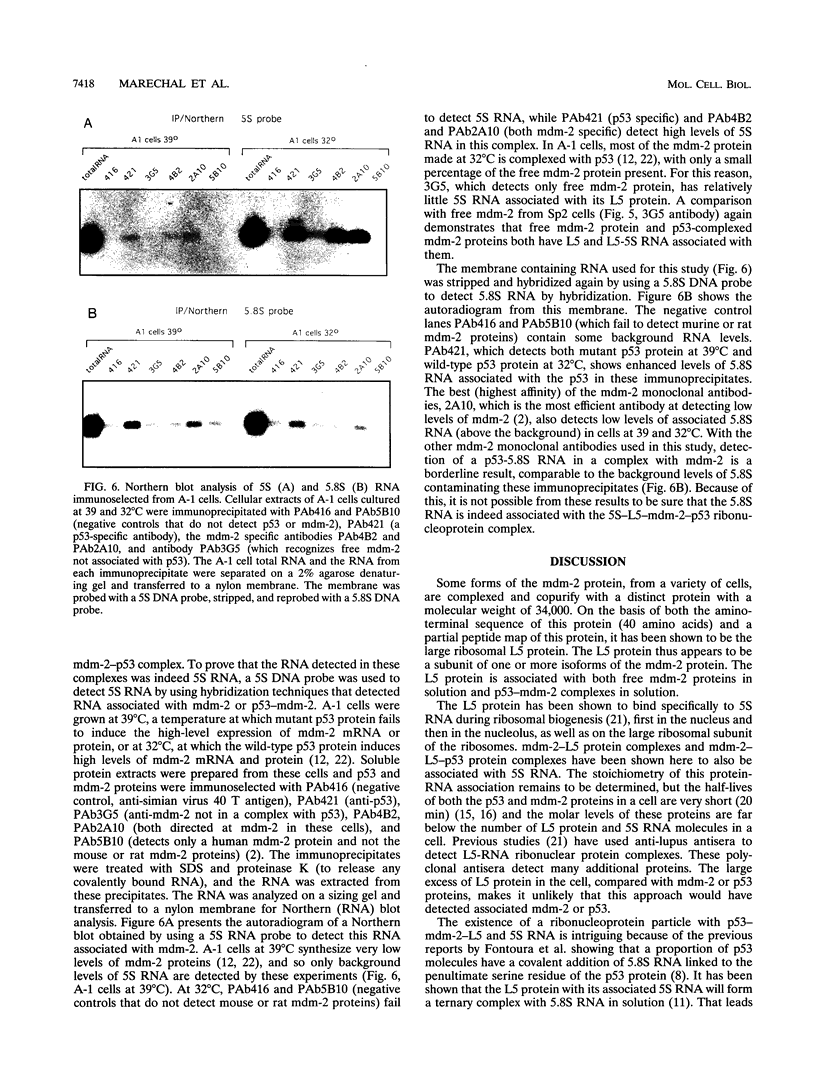
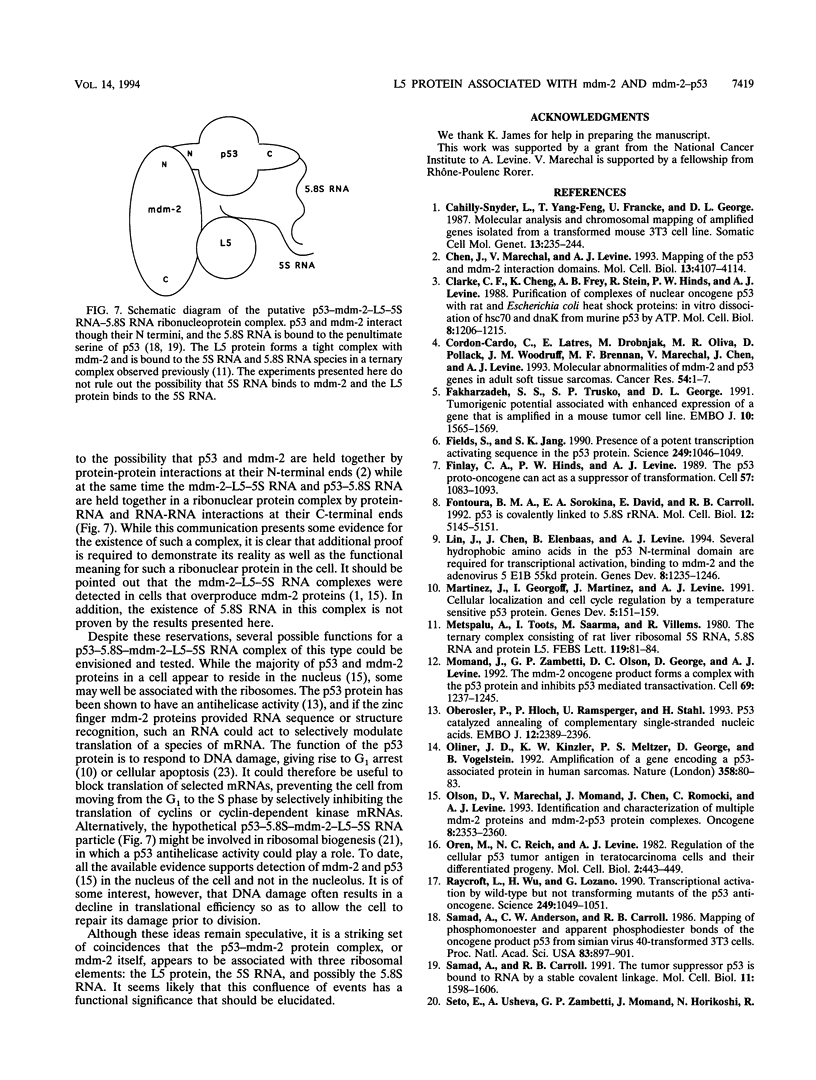
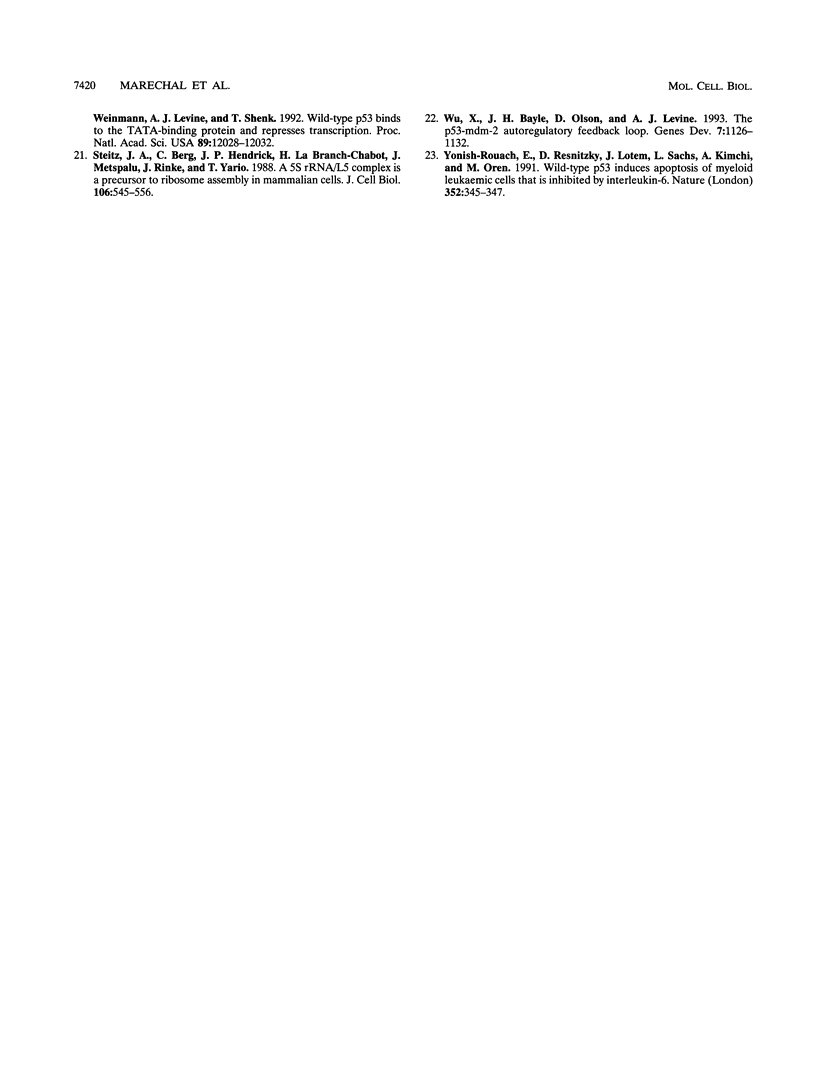
Images in this article
Selected References
These references are in PubMed. This may not be the complete list of references from this article.
- Cahilly-Snyder L., Yang-Feng T., Francke U., George D. L. Molecular analysis and chromosomal mapping of amplified genes isolated from a transformed mouse 3T3 cell line. Somat Cell Mol Genet. 1987 May;13(3):235–244. doi: 10.1007/BF01535205. [DOI] [PubMed] [Google Scholar]
- Chen J., Marechal V., Levine A. J. Mapping of the p53 and mdm-2 interaction domains. Mol Cell Biol. 1993 Jul;13(7):4107–4114. doi: 10.1128/mcb.13.7.4107. [DOI] [PMC free article] [PubMed] [Google Scholar]
- Clarke C. F., Cheng K., Frey A. B., Stein R., Hinds P. W., Levine A. J. Purification of complexes of nuclear oncogene p53 with rat and Escherichia coli heat shock proteins: in vitro dissociation of hsc70 and dnaK from murine p53 by ATP. Mol Cell Biol. 1988 Mar;8(3):1206–1215. doi: 10.1128/mcb.8.3.1206. [DOI] [PMC free article] [PubMed] [Google Scholar]
- Fakharzadeh S. S., Trusko S. P., George D. L. Tumorigenic potential associated with enhanced expression of a gene that is amplified in a mouse tumor cell line. EMBO J. 1991 Jun;10(6):1565–1569. doi: 10.1002/j.1460-2075.1991.tb07676.x. [DOI] [PMC free article] [PubMed] [Google Scholar]
- Fields S., Jang S. K. Presence of a potent transcription activating sequence in the p53 protein. Science. 1990 Aug 31;249(4972):1046–1049. doi: 10.1126/science.2144363. [DOI] [PubMed] [Google Scholar]
- Finlay C. A., Hinds P. W., Levine A. J. The p53 proto-oncogene can act as a suppressor of transformation. Cell. 1989 Jun 30;57(7):1083–1093. doi: 10.1016/0092-8674(89)90045-7. [DOI] [PubMed] [Google Scholar]
- Fontoura B. M., Sorokina E. A., David E., Carroll R. B. p53 is covalently linked to 5.8S rRNA. Mol Cell Biol. 1992 Nov;12(11):5145–5151. doi: 10.1128/mcb.12.11.5145. [DOI] [PMC free article] [PubMed] [Google Scholar]
- Lin J., Chen J., Elenbaas B., Levine A. J. Several hydrophobic amino acids in the p53 amino-terminal domain are required for transcriptional activation, binding to mdm-2 and the adenovirus 5 E1B 55-kD protein. Genes Dev. 1994 May 15;8(10):1235–1246. doi: 10.1101/gad.8.10.1235. [DOI] [PubMed] [Google Scholar]
- Martinez J., Georgoff I., Martinez J., Levine A. J. Cellular localization and cell cycle regulation by a temperature-sensitive p53 protein. Genes Dev. 1991 Feb;5(2):151–159. doi: 10.1101/gad.5.2.151. [DOI] [PubMed] [Google Scholar]
- Metspalu A., Toots I., Saarma M., Villems R. The ternary complex consisting of rat liver ribosomal 5 S RNA, 5.8 S RNA and protein L5. FEBS Lett. 1980 Sep 22;119(1):81–84. doi: 10.1016/0014-5793(80)81002-7. [DOI] [PubMed] [Google Scholar]
- Momand J., Zambetti G. P., Olson D. C., George D., Levine A. J. The mdm-2 oncogene product forms a complex with the p53 protein and inhibits p53-mediated transactivation. Cell. 1992 Jun 26;69(7):1237–1245. doi: 10.1016/0092-8674(92)90644-r. [DOI] [PubMed] [Google Scholar]
- Oberosler P., Hloch P., Ramsperger U., Stahl H. p53-catalyzed annealing of complementary single-stranded nucleic acids. EMBO J. 1993 Jun;12(6):2389–2396. doi: 10.1002/j.1460-2075.1993.tb05893.x. [DOI] [PMC free article] [PubMed] [Google Scholar]
- Oliner J. D., Kinzler K. W., Meltzer P. S., George D. L., Vogelstein B. Amplification of a gene encoding a p53-associated protein in human sarcomas. Nature. 1992 Jul 2;358(6381):80–83. doi: 10.1038/358080a0. [DOI] [PubMed] [Google Scholar]
- Olson D. C., Marechal V., Momand J., Chen J., Romocki C., Levine A. J. Identification and characterization of multiple mdm-2 proteins and mdm-2-p53 protein complexes. Oncogene. 1993 Sep;8(9):2353–2360. [PubMed] [Google Scholar]
- Oren M., Reich N. C., Levine A. J. Regulation of the cellular p53 tumor antigen in teratocarcinoma cells and their differentiated progeny. Mol Cell Biol. 1982 Apr;2(4):443–449. doi: 10.1128/mcb.2.4.443. [DOI] [PMC free article] [PubMed] [Google Scholar]
- Raycroft L., Wu H. Y., Lozano G. Transcriptional activation by wild-type but not transforming mutants of the p53 anti-oncogene. Science. 1990 Aug 31;249(4972):1049–1051. doi: 10.1126/science.2144364. [DOI] [PMC free article] [PubMed] [Google Scholar]
- Samad A., Anderson C. W., Carroll R. B. Mapping of phosphomonoester and apparent phosphodiester bonds of the oncogene product p53 from simian virus 40-transformed 3T3 cells. Proc Natl Acad Sci U S A. 1986 Feb;83(4):897–901. doi: 10.1073/pnas.83.4.897. [DOI] [PMC free article] [PubMed] [Google Scholar]
- Samad A., Carroll R. B. The tumor suppressor p53 is bound to RNA by a stable covalent linkage. Mol Cell Biol. 1991 Mar;11(3):1598–1606. doi: 10.1128/mcb.11.3.1598. [DOI] [PMC free article] [PubMed] [Google Scholar]
- Seto E., Usheva A., Zambetti G. P., Momand J., Horikoshi N., Weinmann R., Levine A. J., Shenk T. Wild-type p53 binds to the TATA-binding protein and represses transcription. Proc Natl Acad Sci U S A. 1992 Dec 15;89(24):12028–12032. doi: 10.1073/pnas.89.24.12028. [DOI] [PMC free article] [PubMed] [Google Scholar]
- Steitz J. A., Berg C., Hendrick J. P., La Branche-Chabot H., Metspalu A., Rinke J., Yario T. A 5S rRNA/L5 complex is a precursor to ribosome assembly in mammalian cells. J Cell Biol. 1988 Mar;106(3):545–556. doi: 10.1083/jcb.106.3.545. [DOI] [PMC free article] [PubMed] [Google Scholar]
- Wu X., Bayle J. H., Olson D., Levine A. J. The p53-mdm-2 autoregulatory feedback loop. Genes Dev. 1993 Jul;7(7A):1126–1132. doi: 10.1101/gad.7.7a.1126. [DOI] [PubMed] [Google Scholar]
- Yonish-Rouach E., Resnitzky D., Lotem J., Sachs L., Kimchi A., Oren M. Wild-type p53 induces apoptosis of myeloid leukaemic cells that is inhibited by interleukin-6. Nature. 1991 Jul 25;352(6333):345–347. doi: 10.1038/352345a0. [DOI] [PubMed] [Google Scholar]