Abstract
We have designed an in vitro system using mammalian nuclear extracts, or fractions derived from them, that can restore the sequences missing at double-strand breaks (gaps) or in deletions. The recombination substrates consist of (i) recipient DNA, pSV2neo with gaps or deletions ranging from 70 to 390 bp in the neo sequence, and (ii) donor DNAs with either complete homology to the recipient (pSV2neo) or plasmids whose homology with pSV2neo is limited to a 1.0- to 1.3-kbp neo segment spanning the gaps or deletions. Incubation of these substrates with various enzyme fractions results in repair of the recipient DNA's disrupted neo gene. The recombinational repair was monitored by transforming recA Escherichia coli to kanamycin resistance and by a new assay which measures the extent of DNA strand transfer from the donor substrate to the recipient DNA. Thus, either streptavidin- or antidigoxigenin-tagged beads are used to separate the biotinylated or digoxigeninylated recipient DNA, respectively, after incubation with the isotopically labeled donor DNA. In contrast to the transfection assay, the DNA strand transfer measurements are direct, quantitative, rapid, and easy, and they provide starting material for the characterization of the recombination products and intermediates. Accordingly, DNA bound to beads serves as a suitable template for the polymerase chain reaction. With appropriate pairs of oligonucleotide primers, we have confirmed that both gaps and deletions are fully repaired, that deletions can be transferred from the recipient DNA to the donor's intact neo sequence, and that cointegrant molecules containing donor and recipient DNA sequences are formed.
Full text
PDF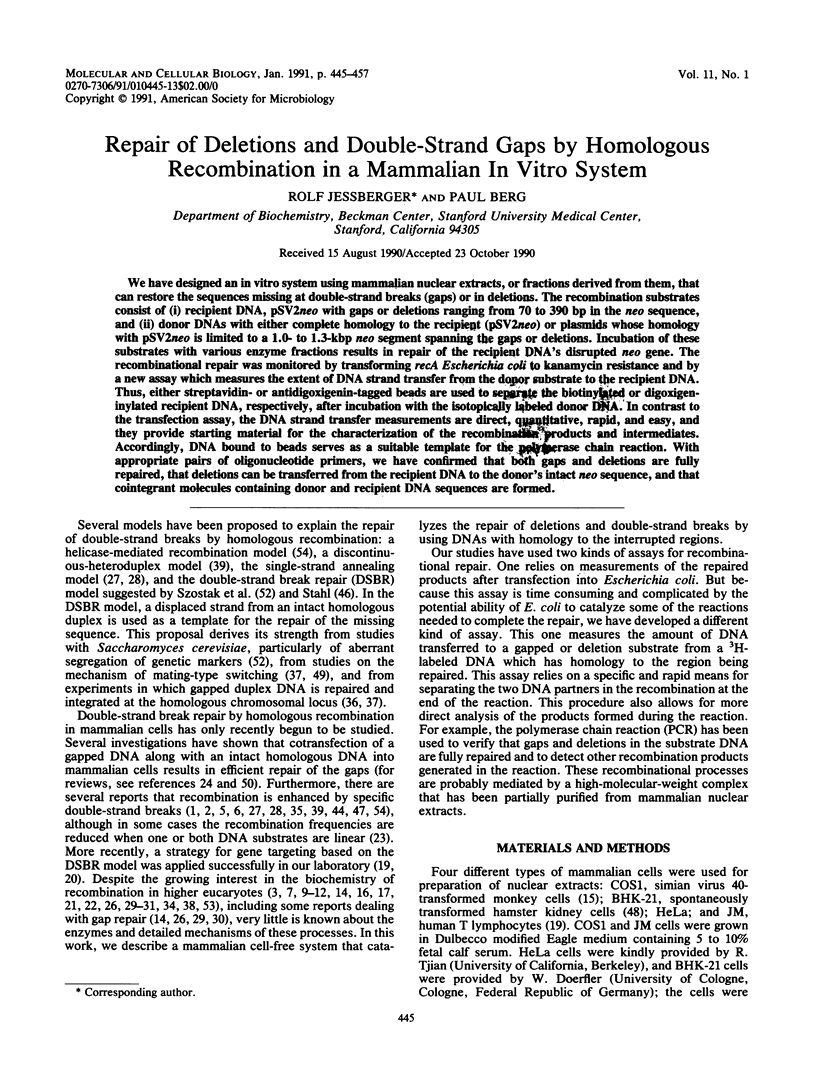
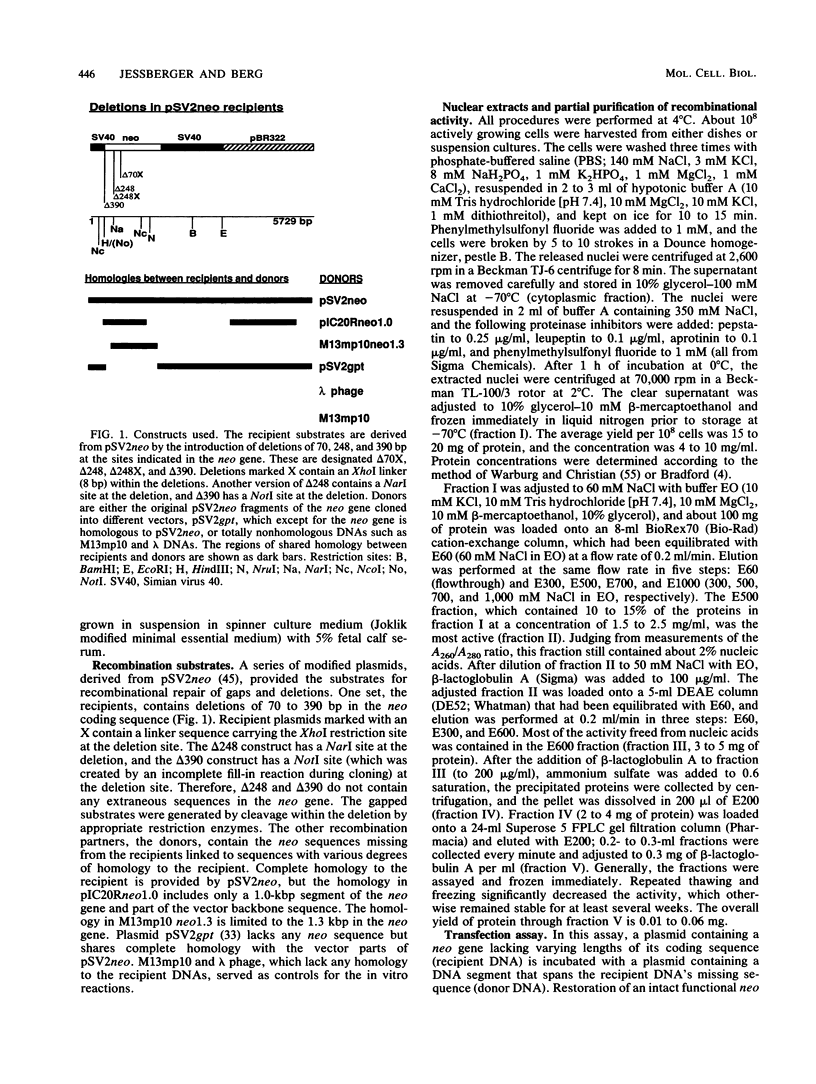
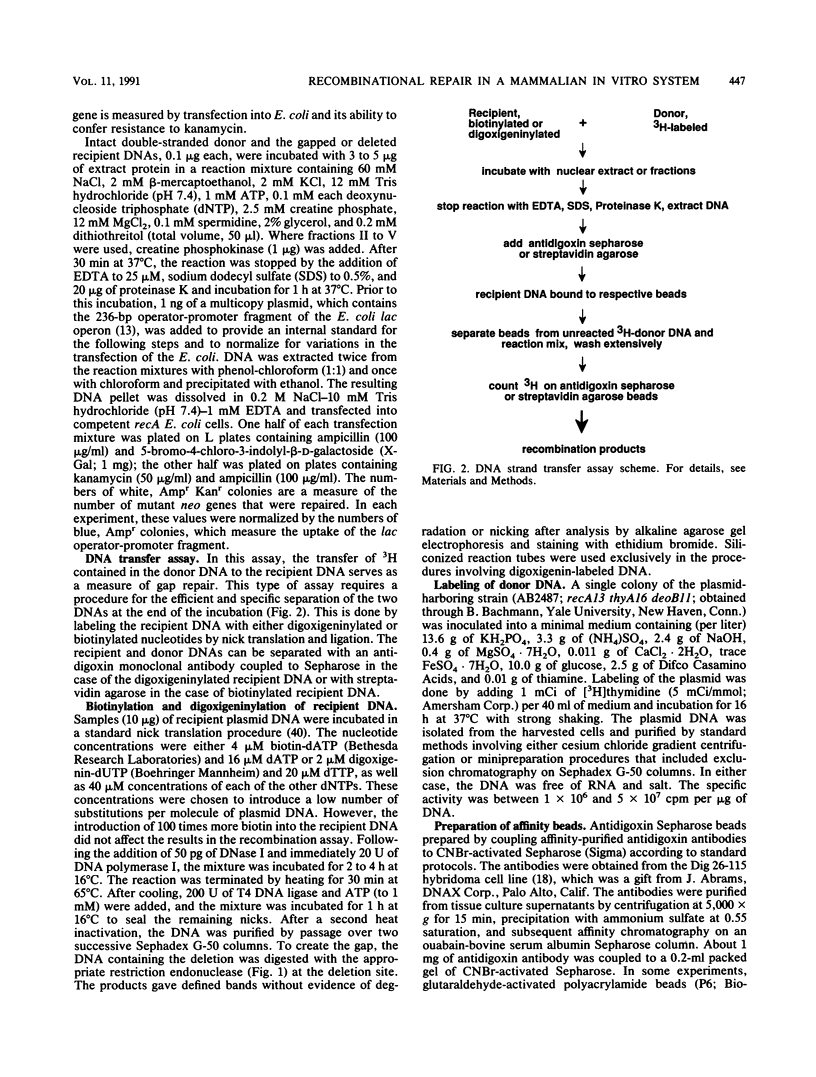
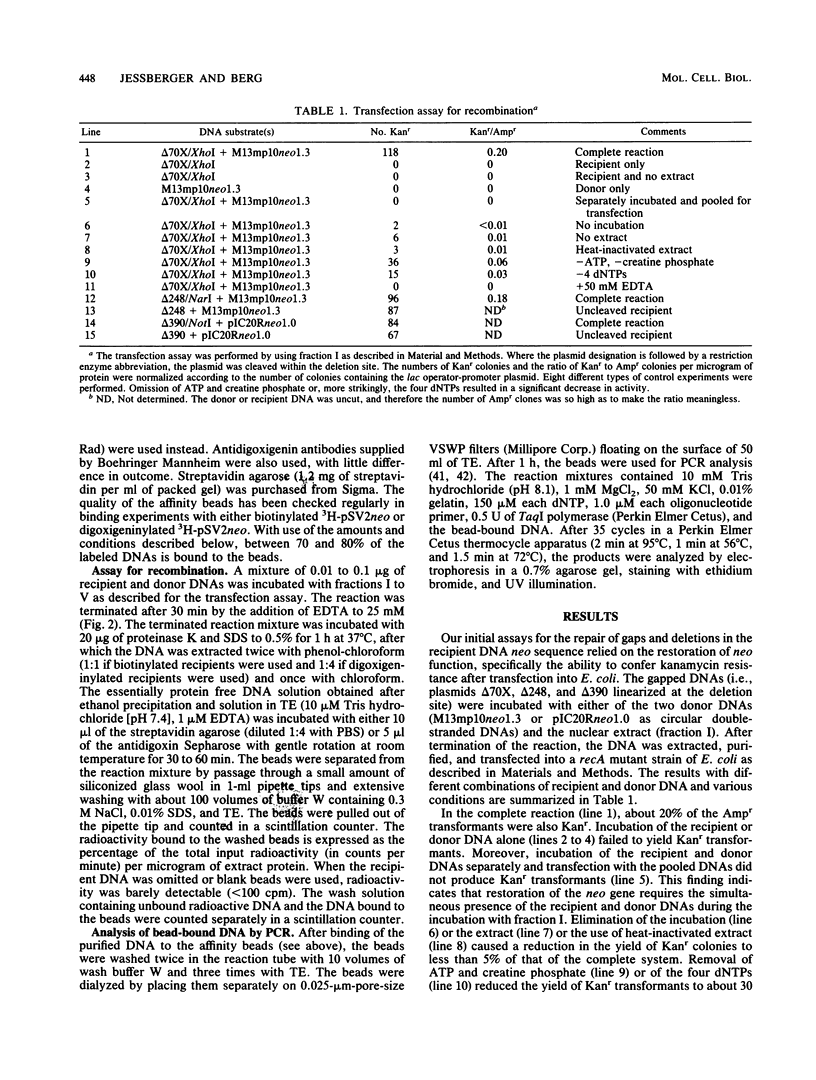
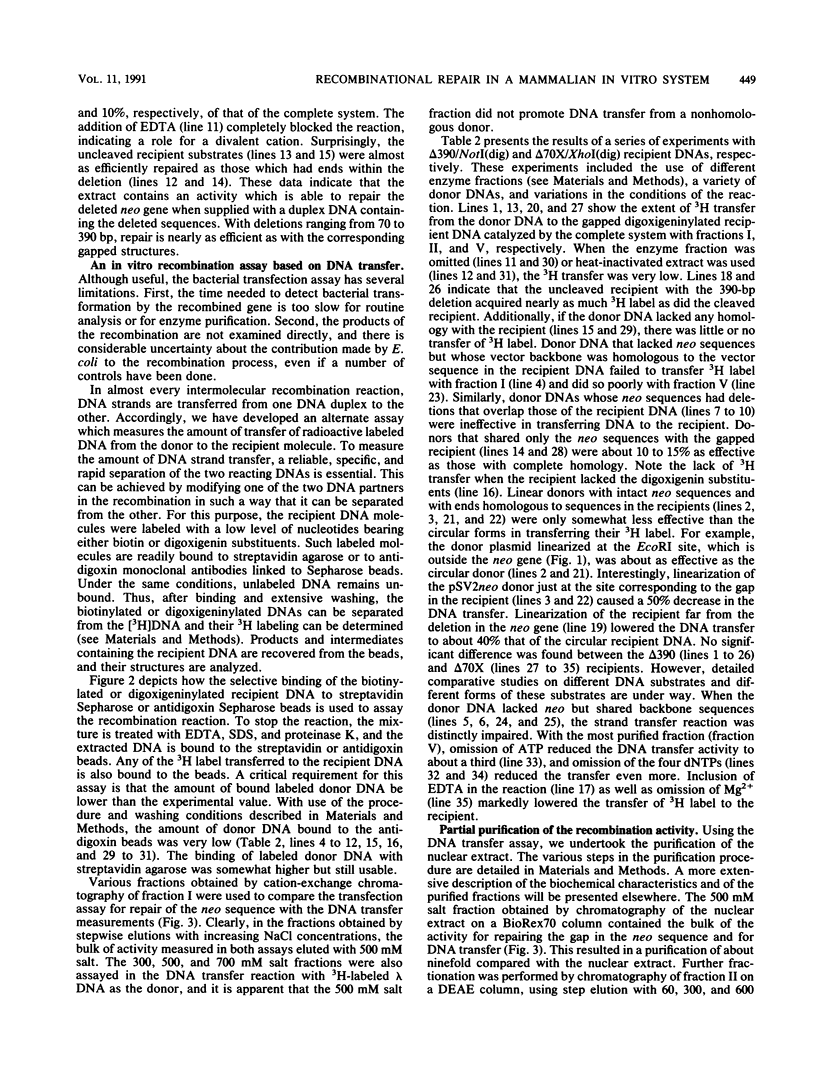
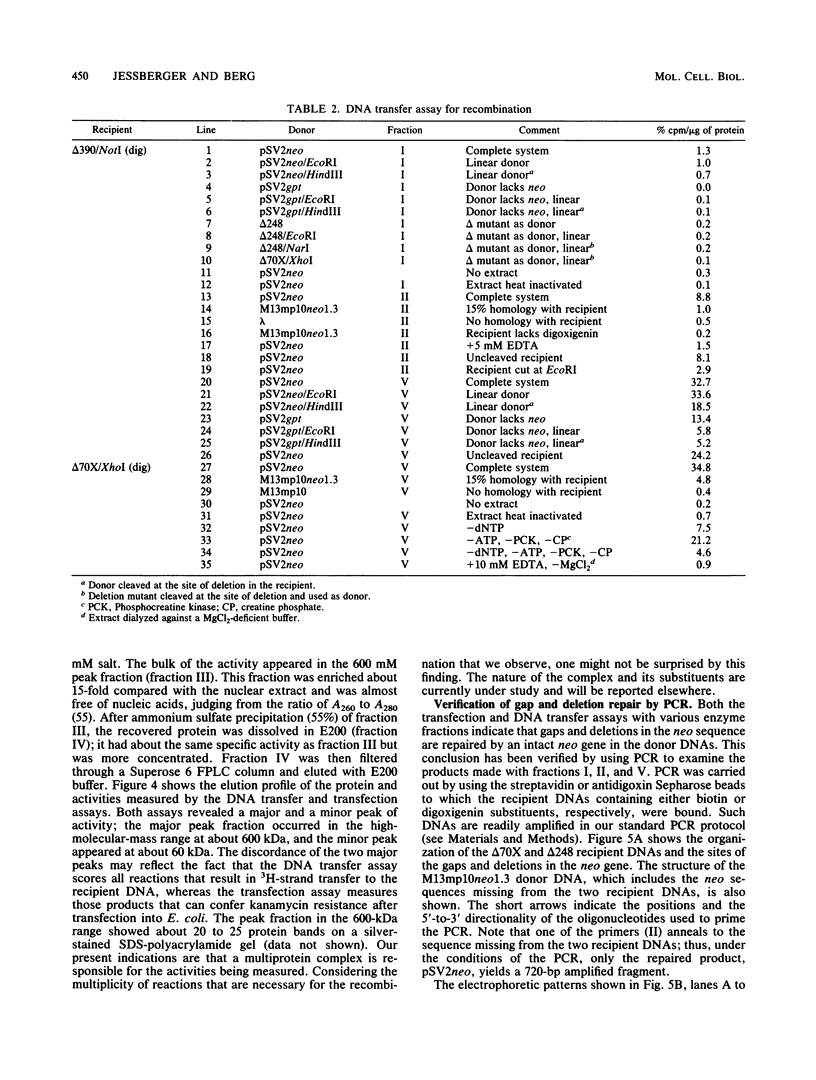
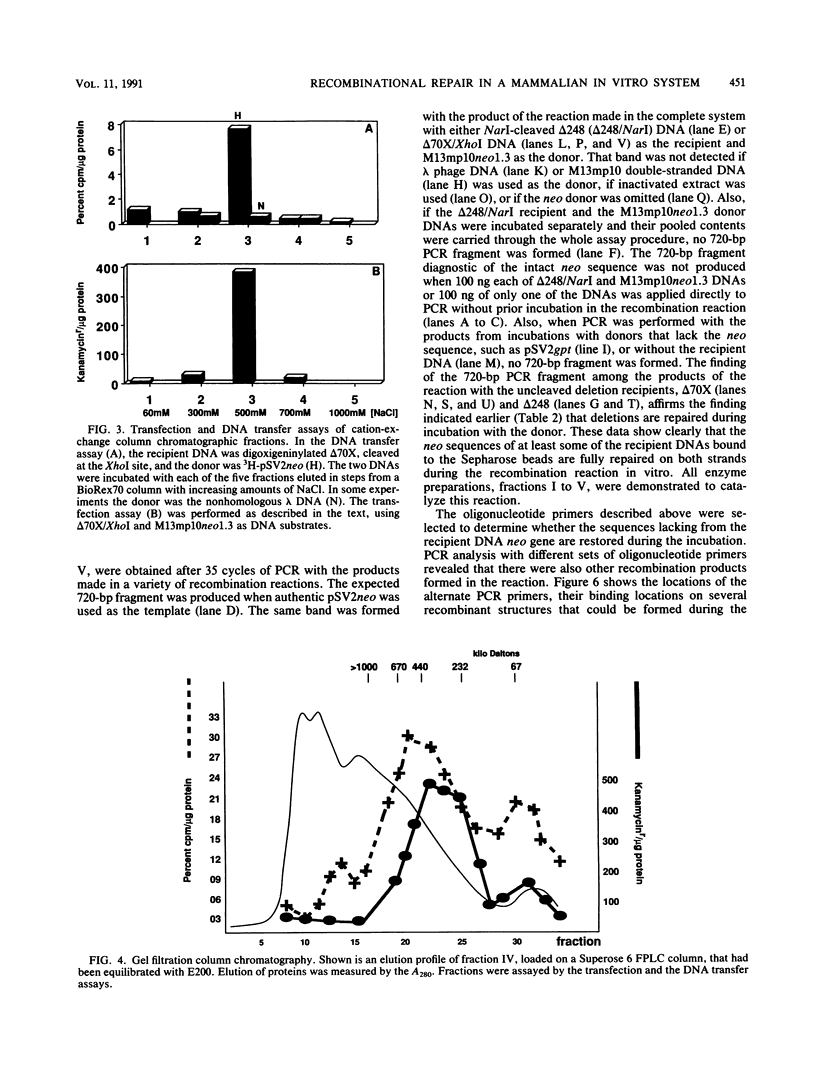
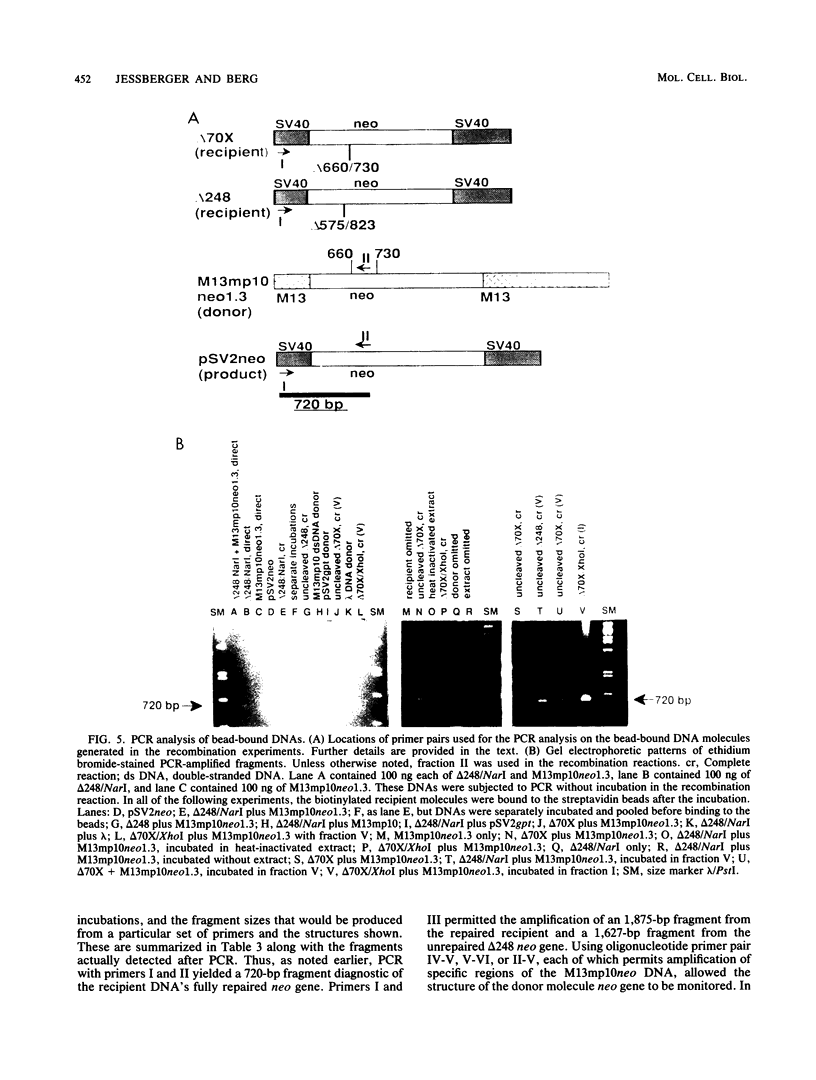
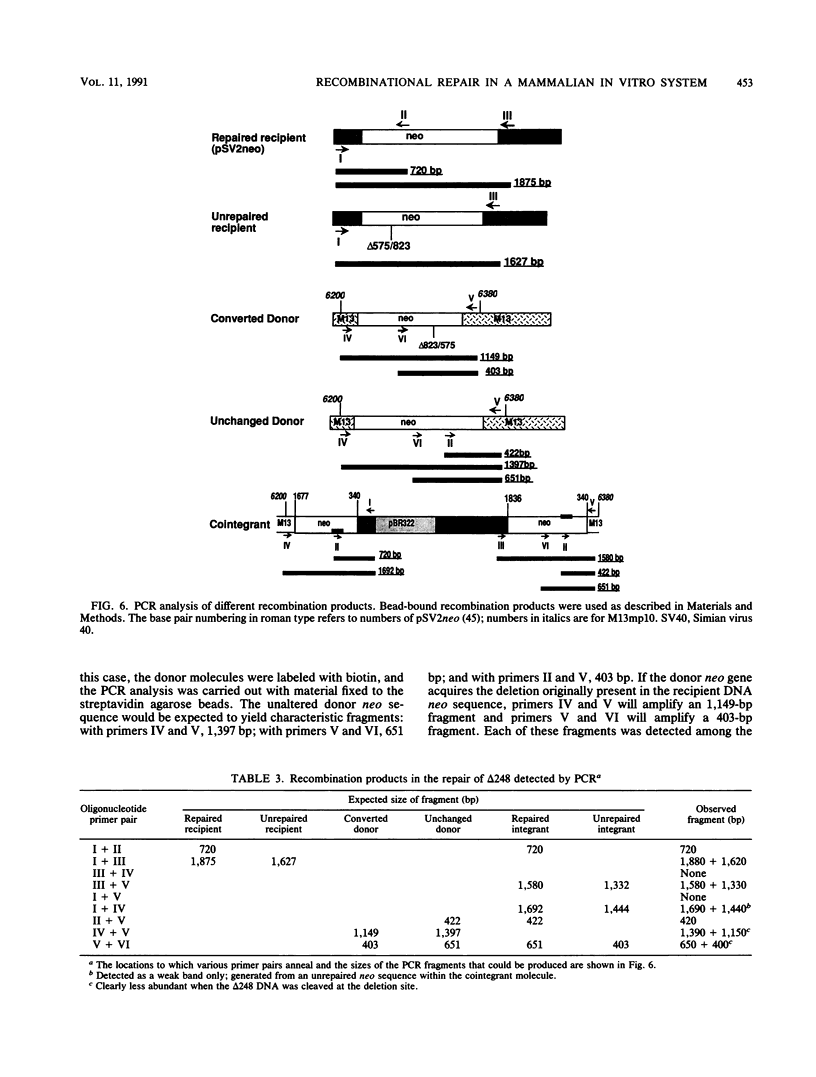
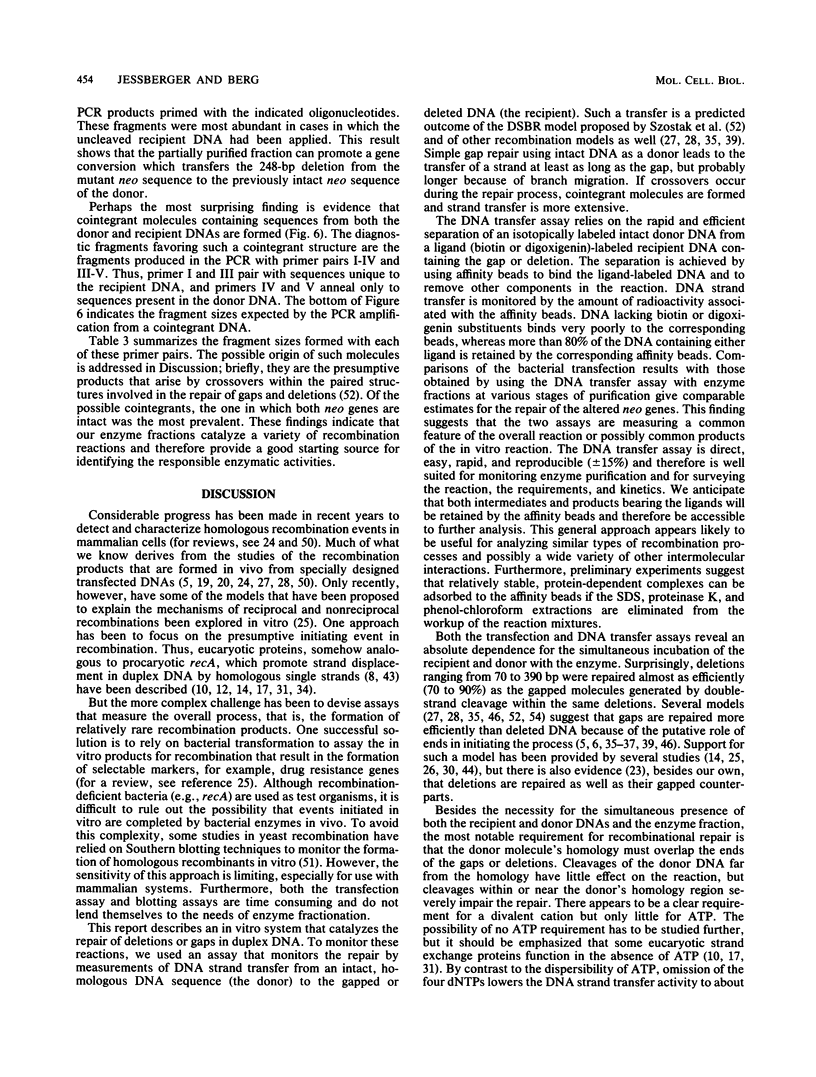
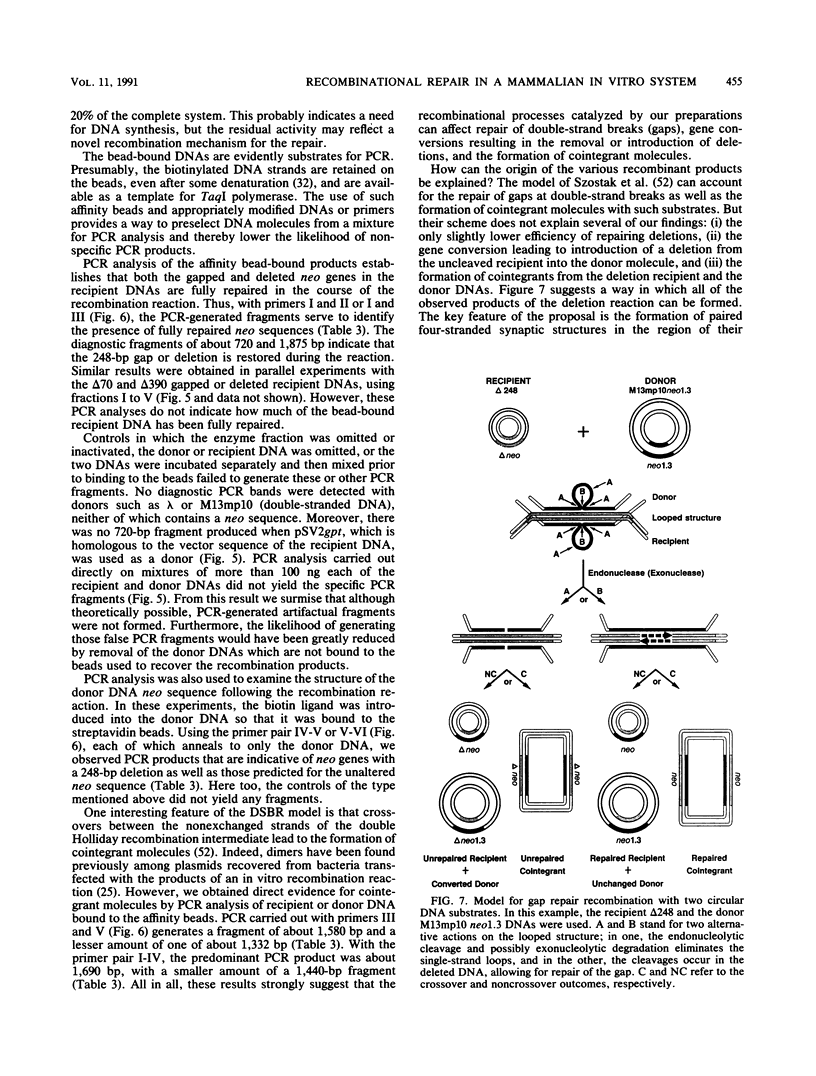
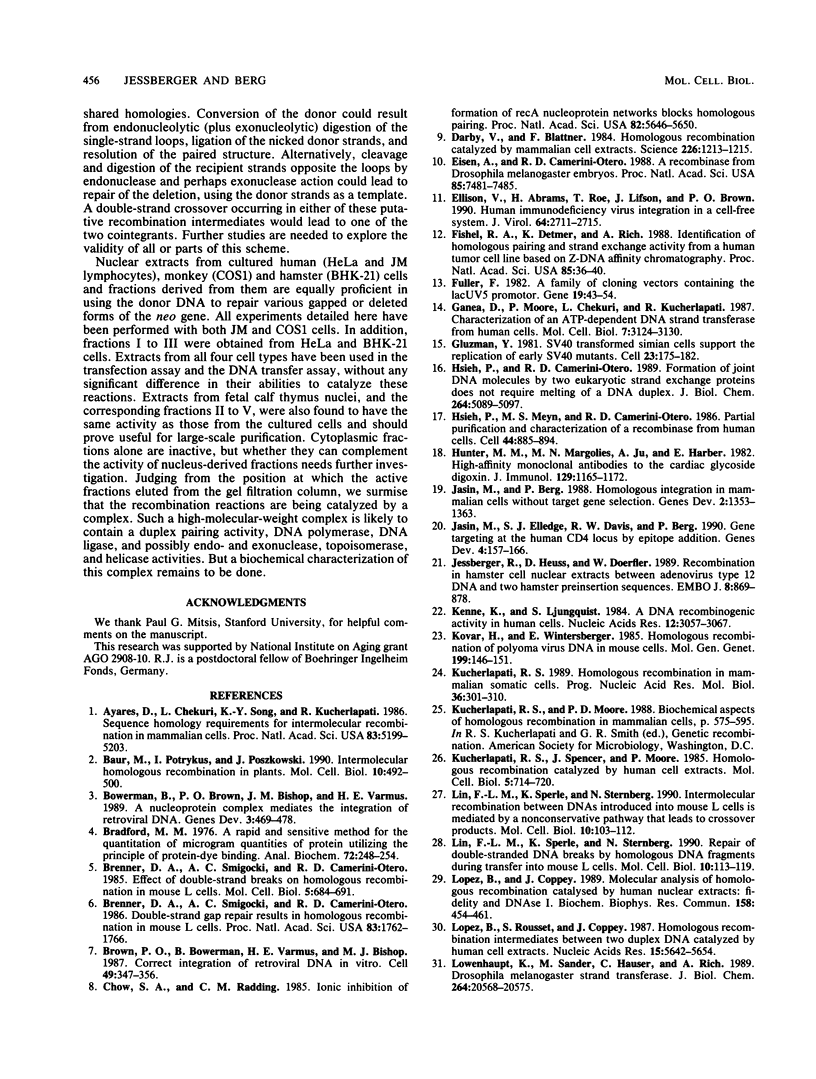
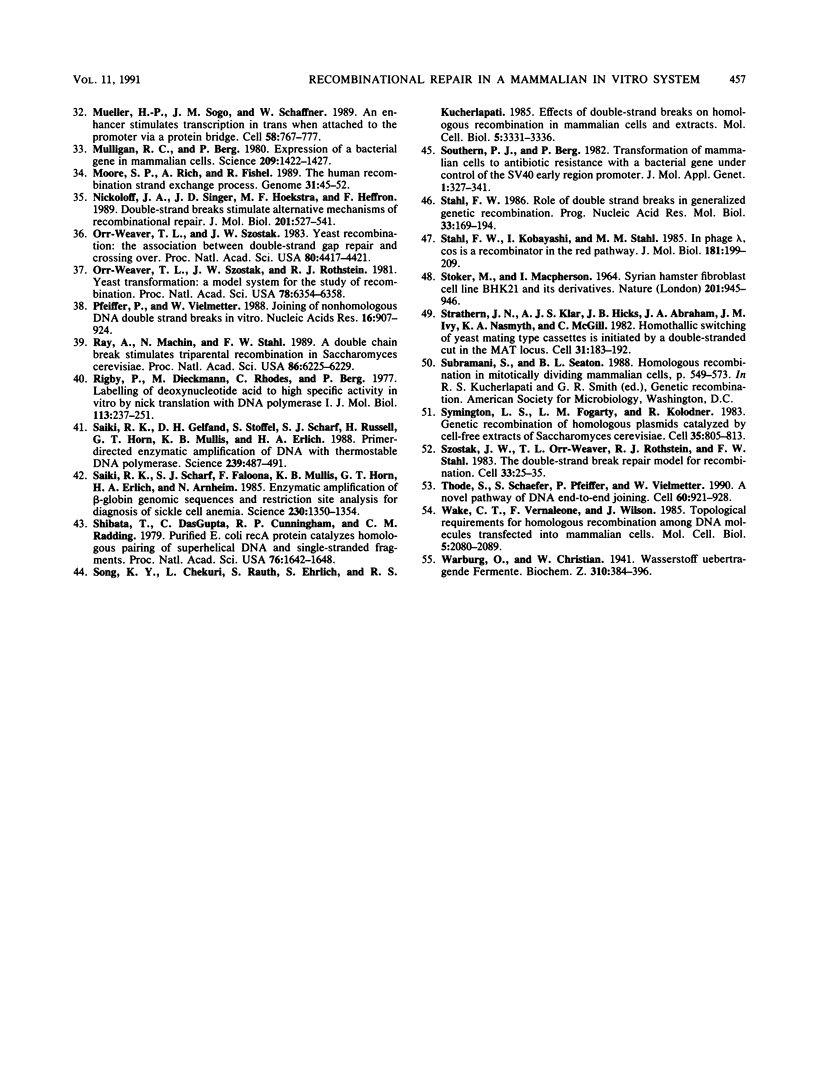
Images in this article
Selected References
These references are in PubMed. This may not be the complete list of references from this article.
- Ayares D., Chekuri L., Song K. Y., Kucherlapati R. Sequence homology requirements for intermolecular recombination in mammalian cells. Proc Natl Acad Sci U S A. 1986 Jul;83(14):5199–5203. doi: 10.1073/pnas.83.14.5199. [DOI] [PMC free article] [PubMed] [Google Scholar]
- Baur M., Potrykus I., Paszkowski J. Intermolecular homologous recombination in plants. Mol Cell Biol. 1990 Feb;10(2):492–500. doi: 10.1128/mcb.10.2.492. [DOI] [PMC free article] [PubMed] [Google Scholar]
- Bowerman B., Brown P. O., Bishop J. M., Varmus H. E. A nucleoprotein complex mediates the integration of retroviral DNA. Genes Dev. 1989 Apr;3(4):469–478. doi: 10.1101/gad.3.4.469. [DOI] [PubMed] [Google Scholar]
- Bradford M. M. A rapid and sensitive method for the quantitation of microgram quantities of protein utilizing the principle of protein-dye binding. Anal Biochem. 1976 May 7;72:248–254. doi: 10.1016/0003-2697(76)90527-3. [DOI] [PubMed] [Google Scholar]
- Brenner D. A., Smigocki A. C., Camerini-Otero R. D. Double-strand gap repair results in homologous recombination in mouse L cells. Proc Natl Acad Sci U S A. 1986 Mar;83(6):1762–1766. doi: 10.1073/pnas.83.6.1762. [DOI] [PMC free article] [PubMed] [Google Scholar]
- Brenner D. A., Smigocki A. C., Camerini-Otero R. D. Effect of insertions, deletions, and double-strand breaks on homologous recombination in mouse L cells. Mol Cell Biol. 1985 Apr;5(4):684–691. doi: 10.1128/mcb.5.4.684. [DOI] [PMC free article] [PubMed] [Google Scholar]
- Brown P. O., Bowerman B., Varmus H. E., Bishop J. M. Correct integration of retroviral DNA in vitro. Cell. 1987 May 8;49(3):347–356. doi: 10.1016/0092-8674(87)90287-x. [DOI] [PubMed] [Google Scholar]
- Chow S. A., Radding C. M. Ionic inhibition of formation of RecA nucleoprotein networks blocks homologous pairing. Proc Natl Acad Sci U S A. 1985 Sep;82(17):5646–5650. doi: 10.1073/pnas.82.17.5646. [DOI] [PMC free article] [PubMed] [Google Scholar]
- Darby V., Blattner F. Homologous recombination catalyzed by mammalian cell extracts in vitro. Science. 1984 Dec 7;226(4679):1213–1215. doi: 10.1126/science.6334360. [DOI] [PubMed] [Google Scholar]
- Eisen A., Camerini-Otero R. D. A recombinase from Drosophila melanogaster embryos. Proc Natl Acad Sci U S A. 1988 Oct;85(20):7481–7485. doi: 10.1073/pnas.85.20.7481. [DOI] [PMC free article] [PubMed] [Google Scholar]
- Ellison V., Abrams H., Roe T., Lifson J., Brown P. Human immunodeficiency virus integration in a cell-free system. J Virol. 1990 Jun;64(6):2711–2715. doi: 10.1128/jvi.64.6.2711-2715.1990. [DOI] [PMC free article] [PubMed] [Google Scholar]
- FORRESTER J. A., AMBROSE E. J., STOKER M. G. MICROELECTROPHORESIS OF NORMAL AND TRANSFORMED CLONES OF HAMSTER KIDNEY FIBROBLASTS. Nature. 1964 Feb 29;201:945–946. doi: 10.1038/201945a0. [DOI] [PubMed] [Google Scholar]
- Fishel R. A., Detmer K., Rich A. Identification of homologous pairing and strand-exchange activity from a human tumor cell line based on Z-DNA affinity chromatography. Proc Natl Acad Sci U S A. 1988 Jan;85(1):36–40. doi: 10.1073/pnas.85.1.36. [DOI] [PMC free article] [PubMed] [Google Scholar]
- Fuller F. A family of cloning vectors containing the lacUV5 promoter. Gene. 1982 Jul-Aug;19(1):43–54. doi: 10.1016/0378-1119(82)90187-1. [DOI] [PubMed] [Google Scholar]
- Ganea D., Moore P., Chekuri L., Kucherlapati R. Characterization of an ATP-dependent DNA strand transferase from human cells. Mol Cell Biol. 1987 Sep;7(9):3124–3130. doi: 10.1128/mcb.7.9.3124. [DOI] [PMC free article] [PubMed] [Google Scholar]
- Gluzman Y. SV40-transformed simian cells support the replication of early SV40 mutants. Cell. 1981 Jan;23(1):175–182. doi: 10.1016/0092-8674(81)90282-8. [DOI] [PubMed] [Google Scholar]
- Hsieh P., Camerini-Otero R. D. Formation of joint DNA molecules by two eukaryotic strand exchange proteins does not require melting of a DNA duplex. J Biol Chem. 1989 Mar 25;264(9):5089–5097. [PubMed] [Google Scholar]
- Hsieh P., Meyn M. S., Camerini-Otero R. D. Partial purification and characterization of a recombinase from human cells. Cell. 1986 Mar 28;44(6):885–894. doi: 10.1016/0092-8674(86)90011-5. [DOI] [PubMed] [Google Scholar]
- Hunter M. M., Margolies M. N., Ju A., Haber E. High-affinity monoclonal antibodies to the cardiac glycoside, digoxin. J Immunol. 1982 Sep;129(3):1165–1172. [PubMed] [Google Scholar]
- Jasin M., Berg P. Homologous integration in mammalian cells without target gene selection. Genes Dev. 1988 Nov;2(11):1353–1363. doi: 10.1101/gad.2.11.1353. [DOI] [PubMed] [Google Scholar]
- Jasin M., Elledge S. J., Davis R. W., Berg P. Gene targeting at the human CD4 locus by epitope addition. Genes Dev. 1990 Feb;4(2):157–166. doi: 10.1101/gad.4.2.157. [DOI] [PubMed] [Google Scholar]
- Jessberger R., Heuss D., Doerfler W. Recombination in hamster cell nuclear extracts between adenovirus type 12 DNA and two hamster preinsertion sequences. EMBO J. 1989 Mar;8(3):869–878. doi: 10.1002/j.1460-2075.1989.tb03448.x. [DOI] [PMC free article] [PubMed] [Google Scholar]
- Kenne K., Ljungquist S. A DNA-recombinogenic activity in human cells. Nucleic Acids Res. 1984 Apr 11;12(7):3057–3068. doi: 10.1093/nar/12.7.3057. [DOI] [PMC free article] [PubMed] [Google Scholar]
- Kovar H., Wintersberger E. Homologous recombination of polyoma virus DNA in mouse cells. Mol Gen Genet. 1985;199(1):146–151. doi: 10.1007/BF00327524. [DOI] [PubMed] [Google Scholar]
- Kucherlapati R. S. Homologous recombination in mammalian somatic cells. Prog Nucleic Acid Res Mol Biol. 1989;36:301–310. doi: 10.1016/s0079-6603(08)60178-6. [DOI] [PubMed] [Google Scholar]
- Kucherlapati R. S., Spencer J., Moore P. D. Homologous recombination catalyzed by human cell extracts. Mol Cell Biol. 1985 Apr;5(4):714–720. doi: 10.1128/mcb.5.4.714. [DOI] [PMC free article] [PubMed] [Google Scholar]
- Lin F. L., Sperle K., Sternberg N. Intermolecular recombination between DNAs introduced into mouse L cells is mediated by a nonconservative pathway that leads to crossover products. Mol Cell Biol. 1990 Jan;10(1):103–112. doi: 10.1128/mcb.10.1.103. [DOI] [PMC free article] [PubMed] [Google Scholar]
- Lin F. L., Sperle K., Sternberg N. Repair of double-stranded DNA breaks by homologous DNA fragments during transfer of DNA into mouse L cells. Mol Cell Biol. 1990 Jan;10(1):113–119. doi: 10.1128/mcb.10.1.113. [DOI] [PMC free article] [PubMed] [Google Scholar]
- Lopez B., Coppey J. Molecular analysis of homologous recombination catalysed by human nuclear extract: fidelity and DNase protection. Biochem Biophys Res Commun. 1989 Jan 31;158(2):454–461. doi: 10.1016/s0006-291x(89)80069-5. [DOI] [PubMed] [Google Scholar]
- Lowenhaupt K., Sander M., Hauser C., Rich A. Drosophila melanogaster strand transferase. A protein that forms heteroduplex DNA in the absence of both ATP and single-strand DNA binding protein. J Biol Chem. 1989 Dec 5;264(34):20568–20575. [PubMed] [Google Scholar]
- Moore S. P., Rich A., Fishel R. The human recombination strand exchange process. Genome. 1989;31(1):45–52. doi: 10.1139/g89-012. [DOI] [PubMed] [Google Scholar]
- Mulligan R. C., Berg P. Expression of a bacterial gene in mammalian cells. Science. 1980 Sep 19;209(4463):1422–1427. doi: 10.1126/science.6251549. [DOI] [PubMed] [Google Scholar]
- Müeller-Storm H. P., Sogo J. M., Schaffner W. An enhancer stimulates transcription in trans when attached to the promoter via a protein bridge. Cell. 1989 Aug 25;58(4):767–777. doi: 10.1016/0092-8674(89)90110-4. [DOI] [PubMed] [Google Scholar]
- Nickoloff J. A., Singer J. D., Hoekstra M. F., Heffron F. Double-strand breaks stimulate alternative mechanisms of recombination repair. J Mol Biol. 1989 Jun 5;207(3):527–541. doi: 10.1016/0022-2836(89)90462-2. [DOI] [PubMed] [Google Scholar]
- Orr-Weaver T. L., Szostak J. W., Rothstein R. J. Yeast transformation: a model system for the study of recombination. Proc Natl Acad Sci U S A. 1981 Oct;78(10):6354–6358. doi: 10.1073/pnas.78.10.6354. [DOI] [PMC free article] [PubMed] [Google Scholar]
- Orr-Weaver T. L., Szostak J. W. Yeast recombination: the association between double-strand gap repair and crossing-over. Proc Natl Acad Sci U S A. 1983 Jul;80(14):4417–4421. doi: 10.1073/pnas.80.14.4417. [DOI] [PMC free article] [PubMed] [Google Scholar]
- Pfeiffer P., Vielmetter W. Joining of nonhomologous DNA double strand breaks in vitro. Nucleic Acids Res. 1988 Feb 11;16(3):907–924. doi: 10.1093/nar/16.3.907. [DOI] [PMC free article] [PubMed] [Google Scholar]
- Ray A., Machin N., Stahl F. W. A DNA double chain break stimulates triparental recombination in Saccharomyces cerevisiae. Proc Natl Acad Sci U S A. 1989 Aug;86(16):6225–6229. doi: 10.1073/pnas.86.16.6225. [DOI] [PMC free article] [PubMed] [Google Scholar]
- Rigby P. W., Dieckmann M., Rhodes C., Berg P. Labeling deoxyribonucleic acid to high specific activity in vitro by nick translation with DNA polymerase I. J Mol Biol. 1977 Jun 15;113(1):237–251. doi: 10.1016/0022-2836(77)90052-3. [DOI] [PubMed] [Google Scholar]
- Saiki R. K., Gelfand D. H., Stoffel S., Scharf S. J., Higuchi R., Horn G. T., Mullis K. B., Erlich H. A. Primer-directed enzymatic amplification of DNA with a thermostable DNA polymerase. Science. 1988 Jan 29;239(4839):487–491. doi: 10.1126/science.2448875. [DOI] [PubMed] [Google Scholar]
- Saiki R. K., Scharf S., Faloona F., Mullis K. B., Horn G. T., Erlich H. A., Arnheim N. Enzymatic amplification of beta-globin genomic sequences and restriction site analysis for diagnosis of sickle cell anemia. Science. 1985 Dec 20;230(4732):1350–1354. doi: 10.1126/science.2999980. [DOI] [PubMed] [Google Scholar]
- Shibata T., DasGupta C., Cunningham R. P., Radding C. M. Purified Escherichia coli recA protein catalyzes homologous pairing of superhelical DNA and single-stranded fragments. Proc Natl Acad Sci U S A. 1979 Apr;76(4):1638–1642. doi: 10.1073/pnas.76.4.1638. [DOI] [PMC free article] [PubMed] [Google Scholar]
- Song K. Y., Chekuri L., Rauth S., Ehrlich S., Kucherlapati R. Effect of double-strand breaks on homologous recombination in mammalian cells and extracts. Mol Cell Biol. 1985 Dec;5(12):3331–3336. doi: 10.1128/mcb.5.12.3331. [DOI] [PMC free article] [PubMed] [Google Scholar]
- Southern P. J., Berg P. Transformation of mammalian cells to antibiotic resistance with a bacterial gene under control of the SV40 early region promoter. J Mol Appl Genet. 1982;1(4):327–341. [PubMed] [Google Scholar]
- Stahl F. W., Kobayashi I., Stahl M. M. In phage lambda, cos is a recombinator in the red pathway. J Mol Biol. 1985 Jan 20;181(2):199–209. doi: 10.1016/0022-2836(85)90085-3. [DOI] [PubMed] [Google Scholar]
- Stahl F. W. Roles of double-strand breaks in generalized genetic recombination. Prog Nucleic Acid Res Mol Biol. 1986;33:169–194. doi: 10.1016/s0079-6603(08)60023-9. [DOI] [PubMed] [Google Scholar]
- Strathern J. N., Klar A. J., Hicks J. B., Abraham J. A., Ivy J. M., Nasmyth K. A., McGill C. Homothallic switching of yeast mating type cassettes is initiated by a double-stranded cut in the MAT locus. Cell. 1982 Nov;31(1):183–192. doi: 10.1016/0092-8674(82)90418-4. [DOI] [PubMed] [Google Scholar]
- Symington L. S., Fogarty L. M., Kolodner R. Genetic recombination of homologous plasmids catalyzed by cell-free extracts of Saccharomyces cerevisiae. Cell. 1983 Dec;35(3 Pt 2):805–813. doi: 10.1016/0092-8674(83)90113-7. [DOI] [PubMed] [Google Scholar]
- Szostak J. W., Orr-Weaver T. L., Rothstein R. J., Stahl F. W. The double-strand-break repair model for recombination. Cell. 1983 May;33(1):25–35. doi: 10.1016/0092-8674(83)90331-8. [DOI] [PubMed] [Google Scholar]
- Thode S., Schäfer A., Pfeiffer P., Vielmetter W. A novel pathway of DNA end-to-end joining. Cell. 1990 Mar 23;60(6):921–928. doi: 10.1016/0092-8674(90)90340-k. [DOI] [PubMed] [Google Scholar]
- Wake C. T., Vernaleone F., Wilson J. H. Topological requirements for homologous recombination among DNA molecules transfected into mammalian cells. Mol Cell Biol. 1985 Aug;5(8):2080–2089. doi: 10.1128/mcb.5.8.2080. [DOI] [PMC free article] [PubMed] [Google Scholar]