Abstract
We have used transient transfections in MM14 skeletal muscle cells, newborn rat primary ventricular myocardiocytes, and nonmuscle cells to characterize regulatory elements of the mouse muscle creatine kinase (MCK) gene. Deletion analysis of MCK 5'-flanking sequence reveals a striated muscle-specific, positive regulatory region between -1256 and -1020. A 206-bp fragment from this region acts as a skeletal muscle enhancer and confers orientation-dependent activity in myocardiocytes. A 110-bp enhancer subfragment confers high-level expression in skeletal myocytes but is inactive in myocardiocytes, indicating that skeletal and cardiac muscle MCK regulatory sites are distinguishable. To further delineate muscle regulatory sequences, we tested six sites within the MCK enhancer for their functional importance. Mutations at five sites decrease expression in skeletal muscle, cardiac muscle, and nonmuscle cells. Mutations at two of these sites, Left E box and MEF2, cause similar decreases in all three cell types. Mutations at three sites have larger effects in muscle than nonmuscle cells; an A/T-rich site mutation has a pronounced effect in both striated muscle types, mutations at the MEF1 (Right E-box) site are relatively specific to expression in skeletal muscle, and mutations at the CArG site are relatively specific to expression in cardiac muscle. Changes at the AP2 site tend to increase expression in muscle cells but decrease it in nonmuscle cells. In contrast to reports involving cotransfection of 10T1/2 cells with plasmids expressing the myogenic determination factor MyoD, we show that the skeletal myocyte activity of multimerized MEF1 sites is 30-fold lower than that of the 206-bp enhancer. Thus, MyoD binding sites alone are not sufficient for high-level expression in skeletal myocytes containing endogenous levels of MyoD and other myogenic determination factors.
Full text
PDF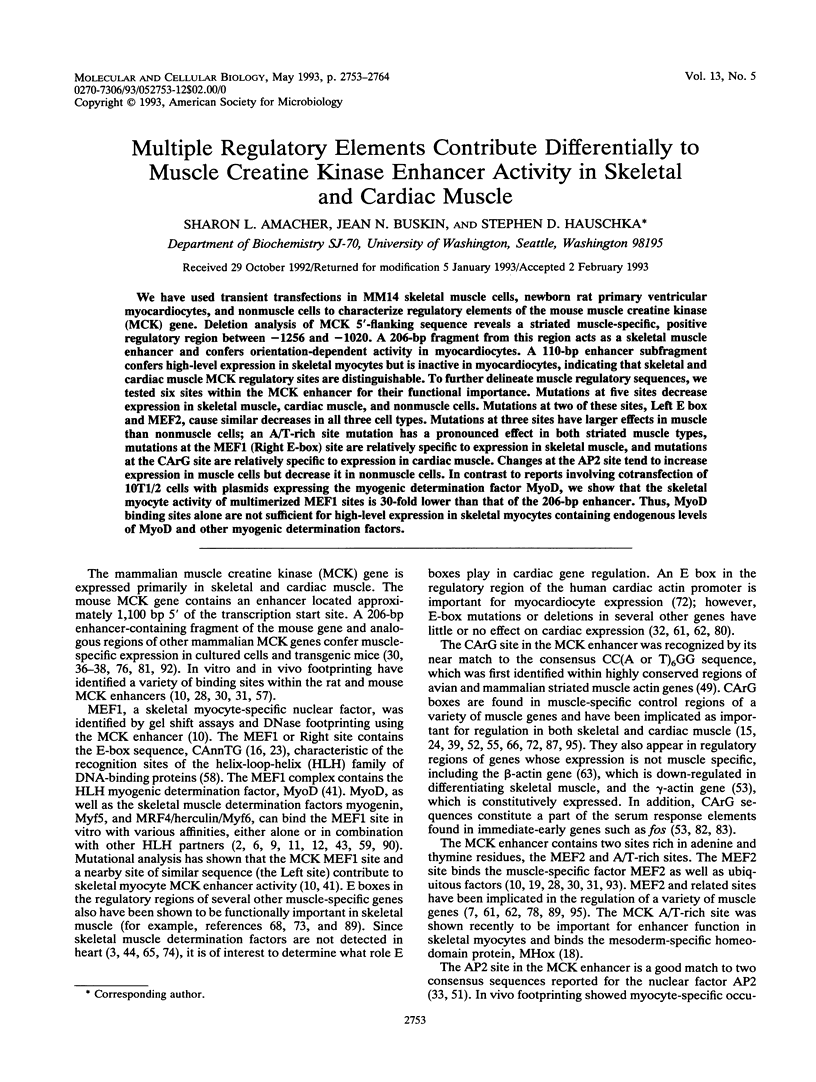
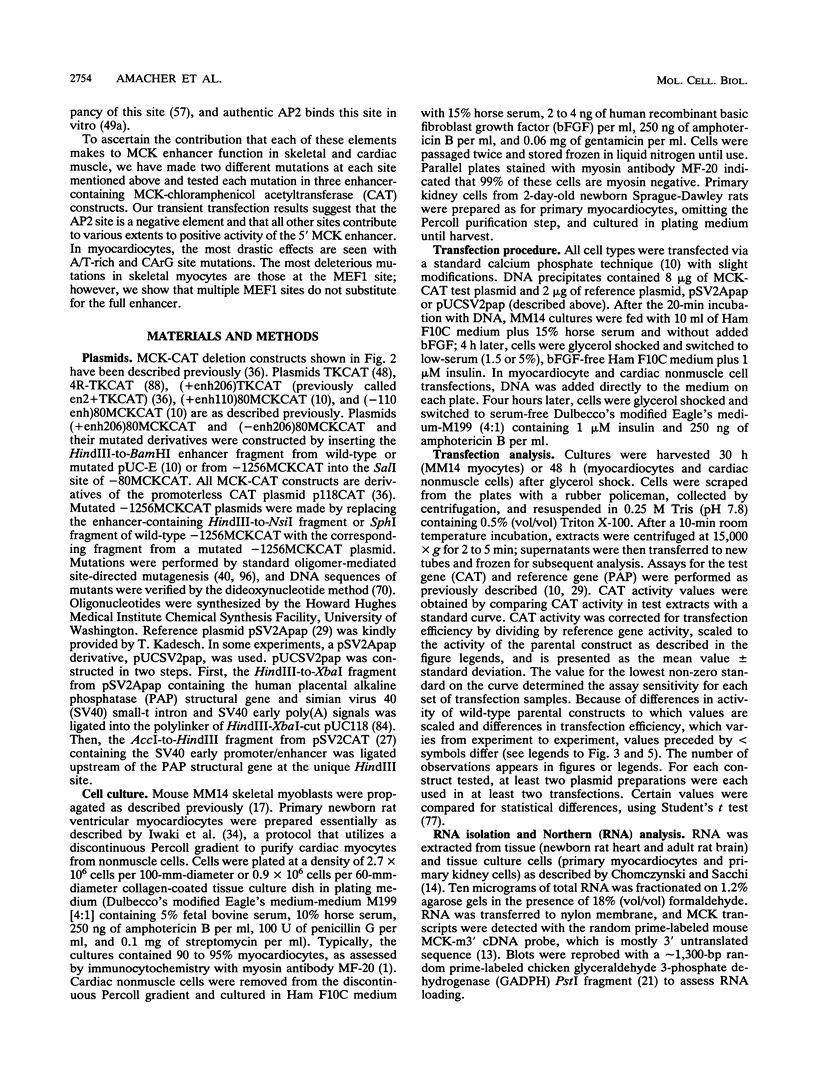
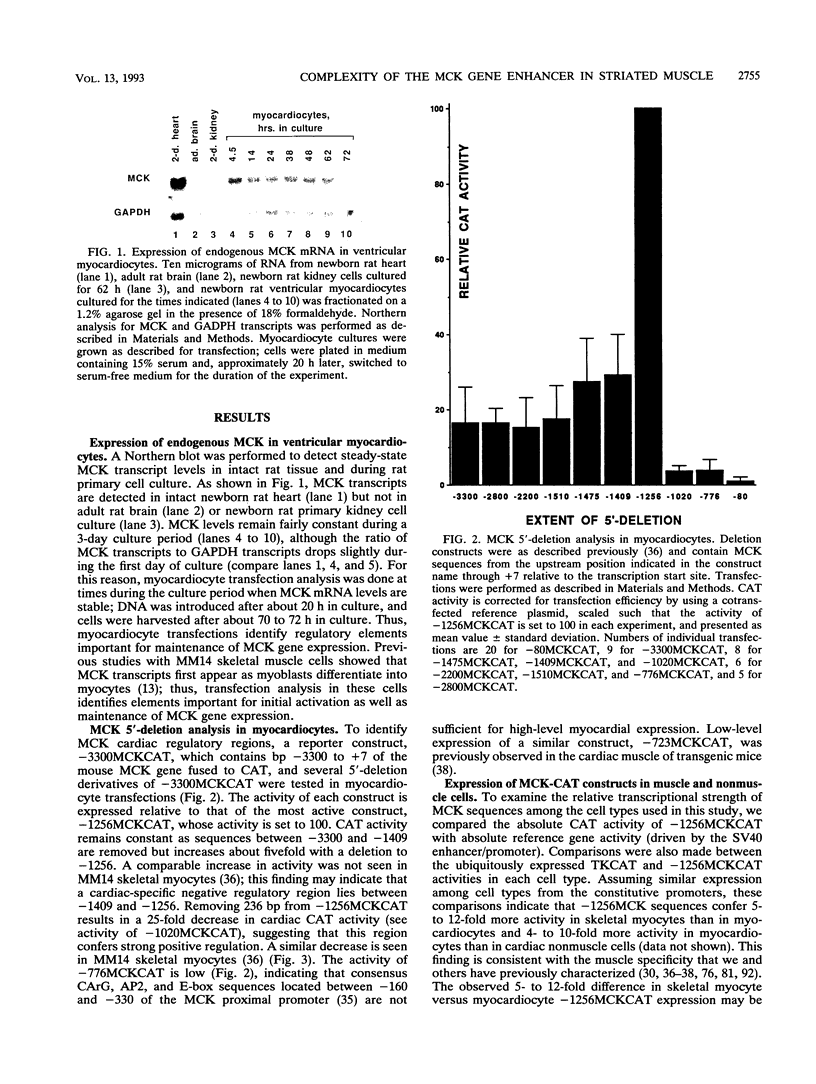
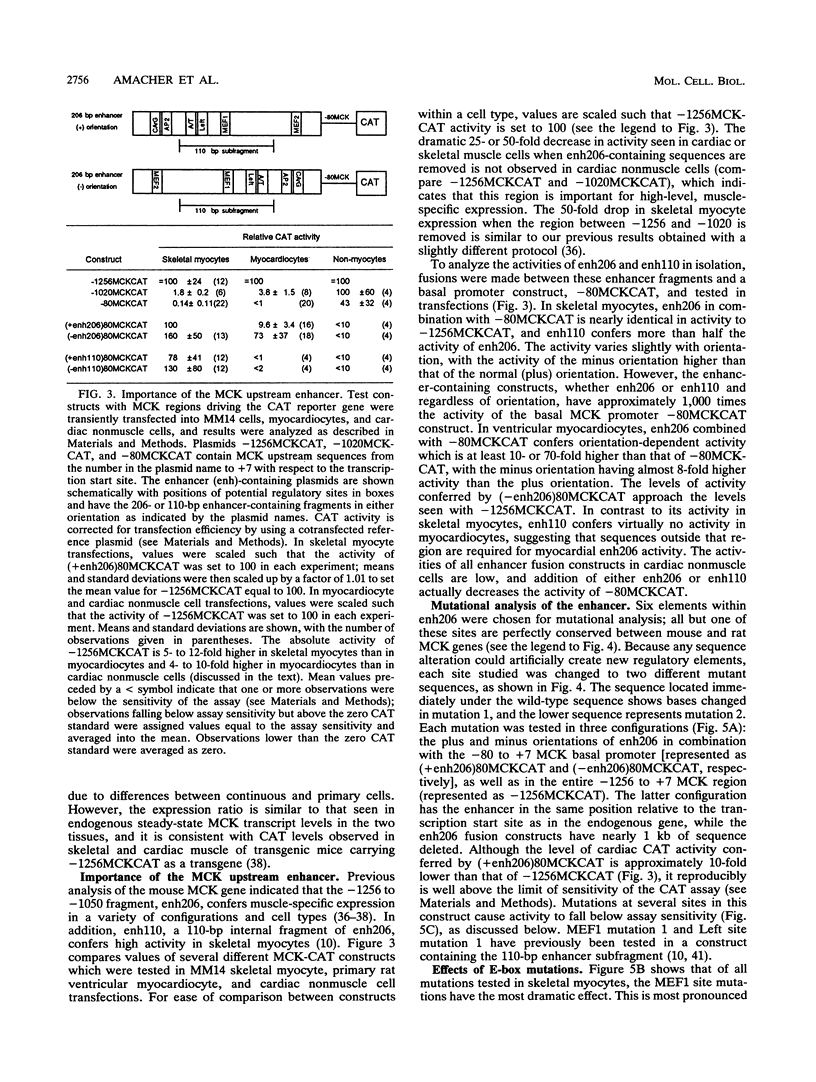
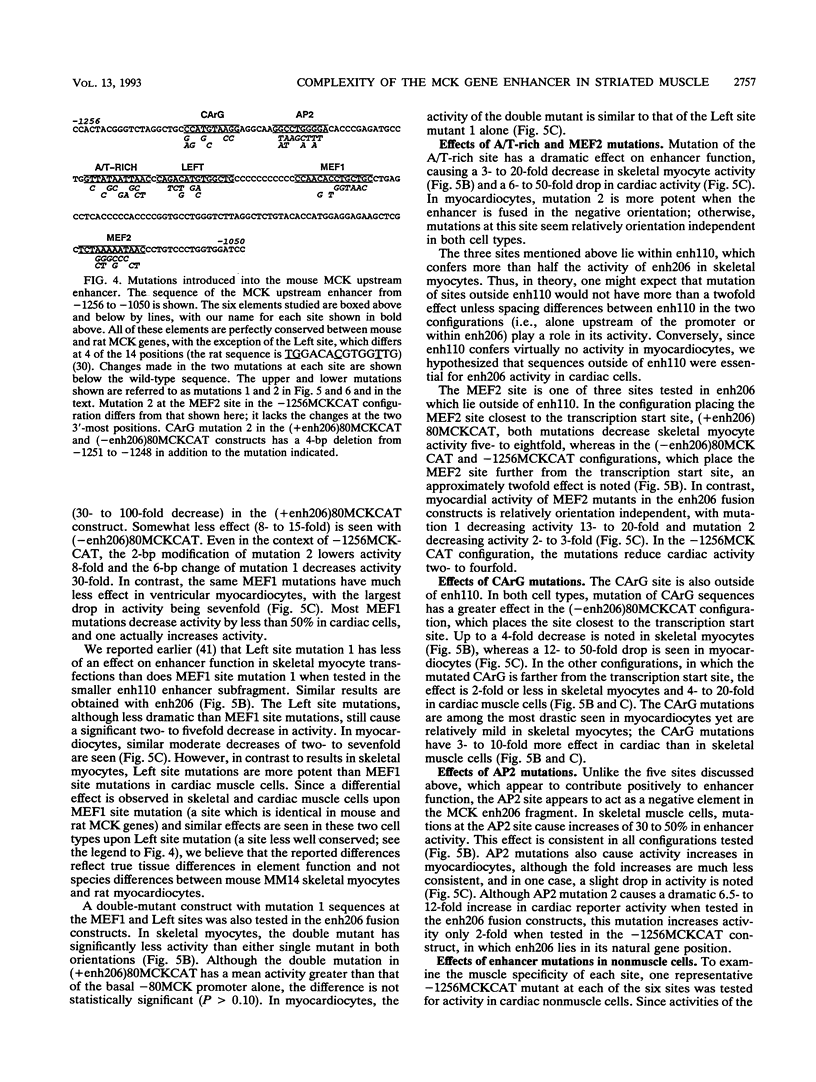
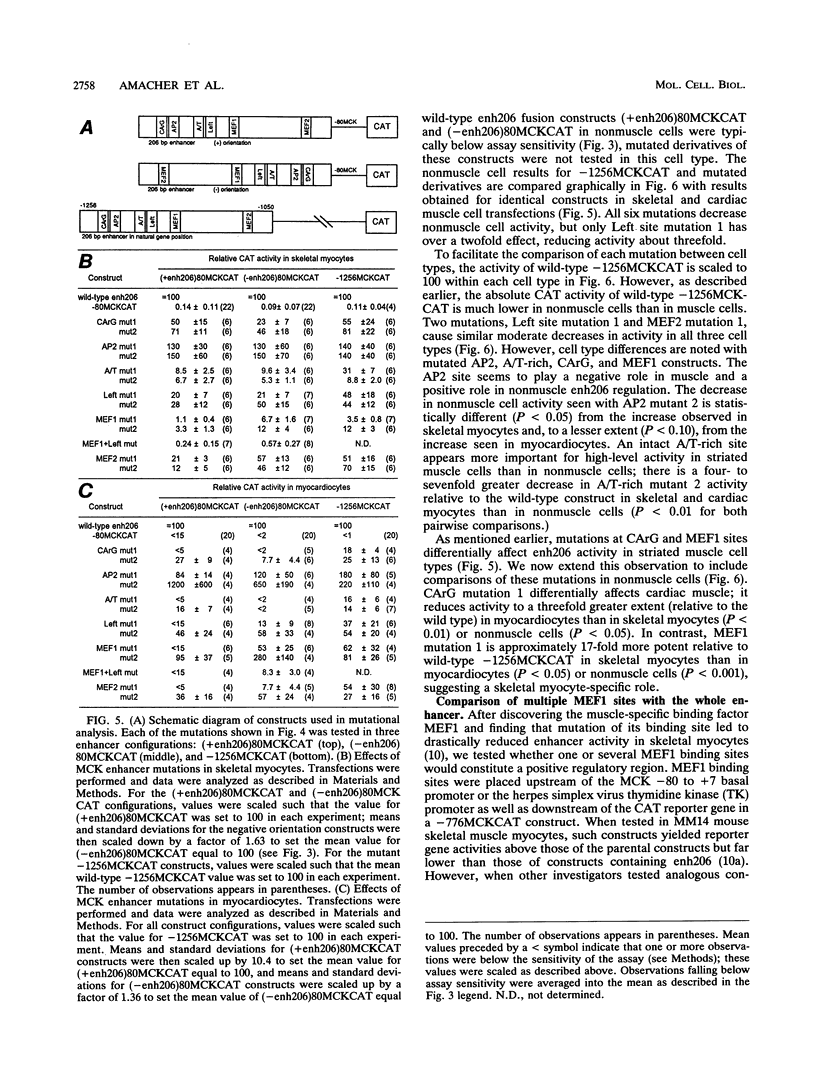
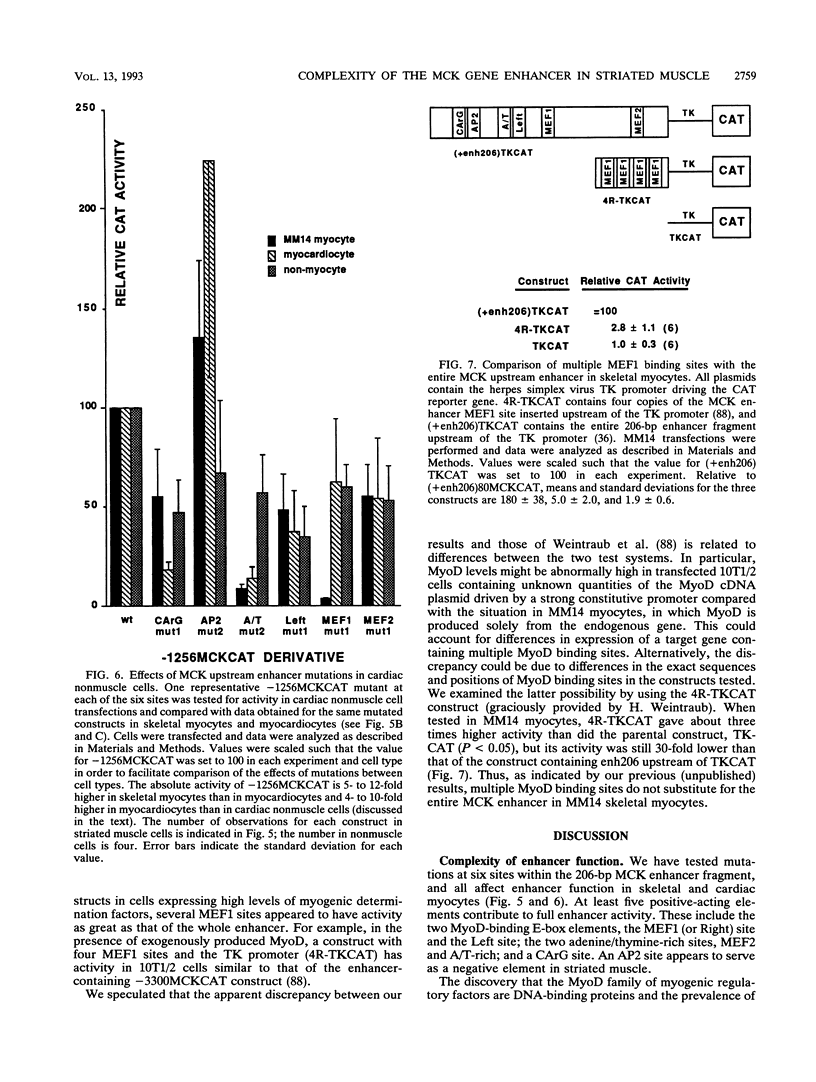
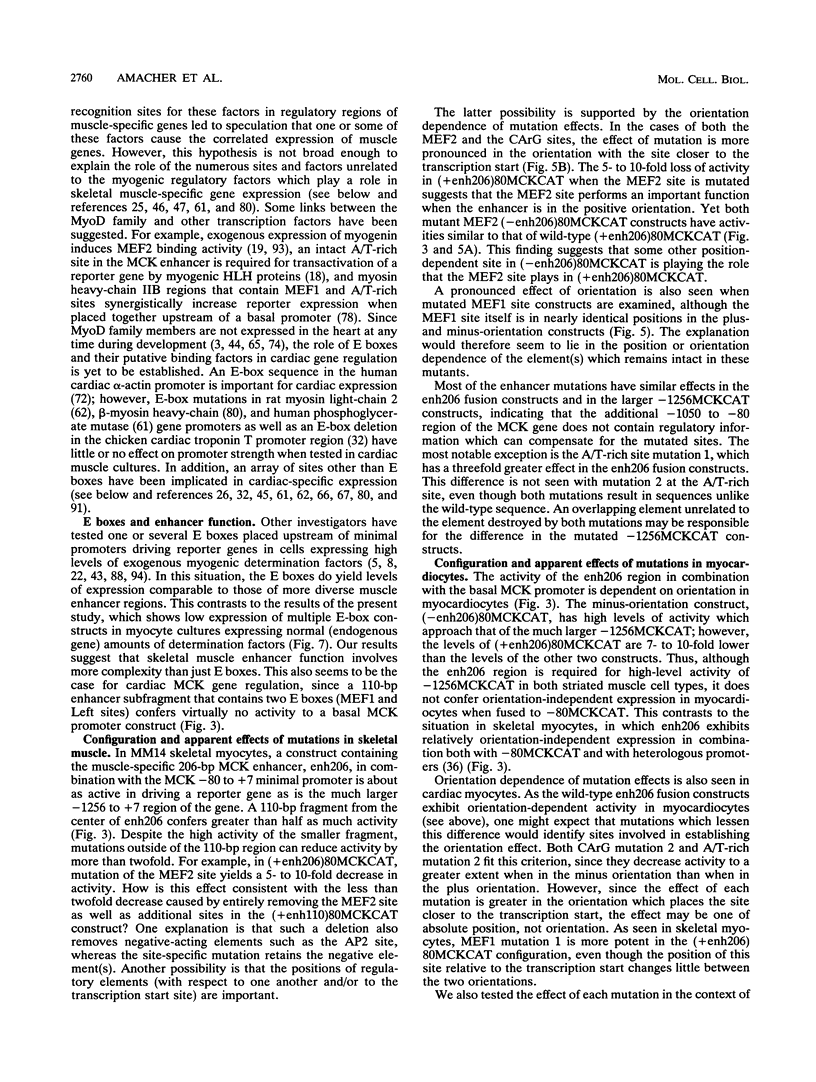
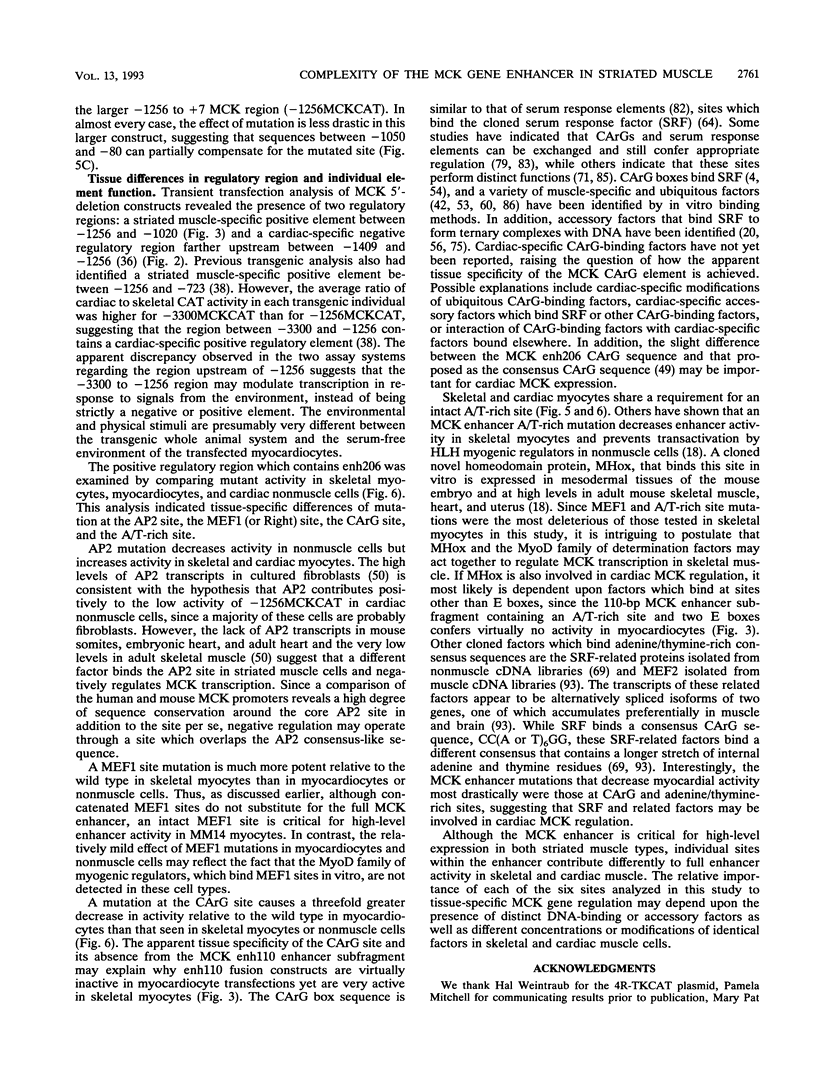
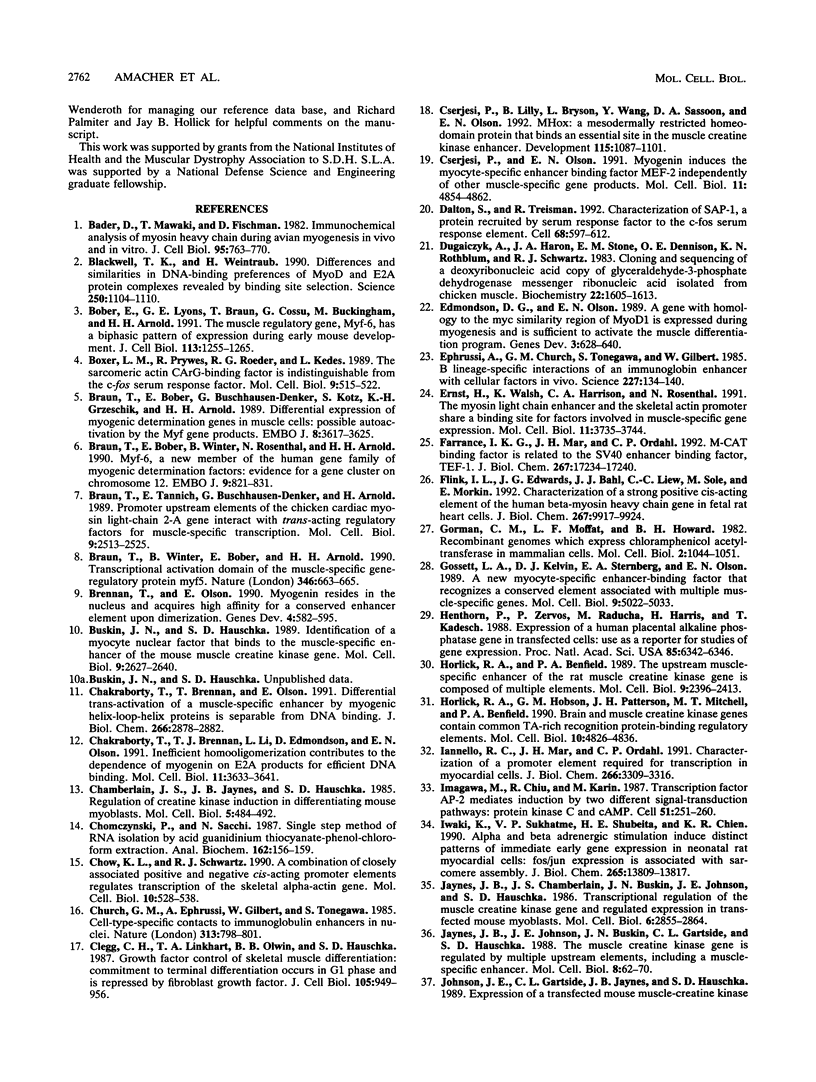
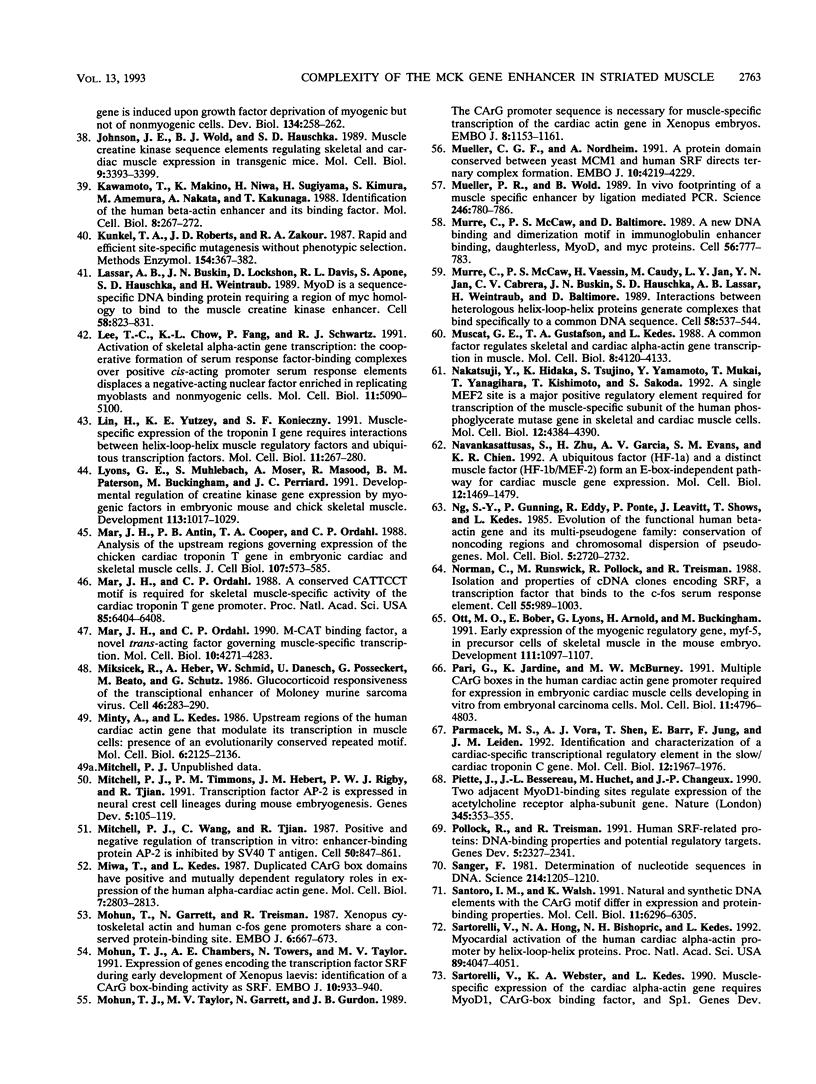
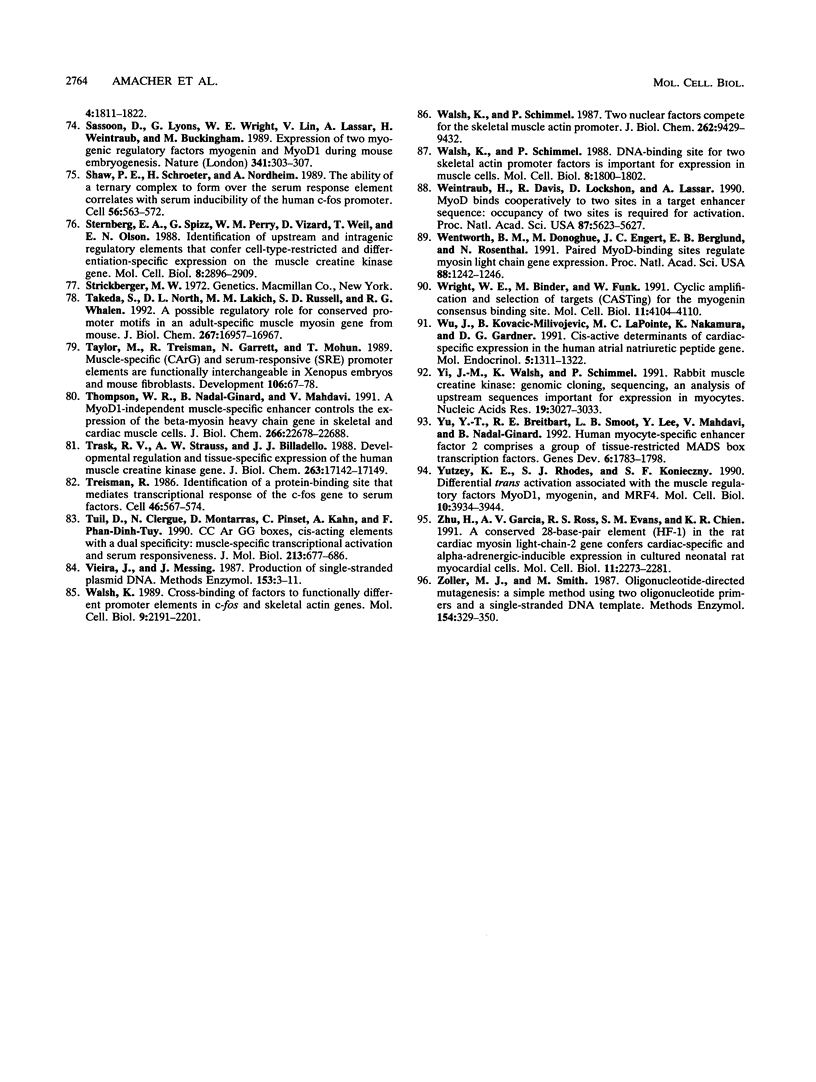
Images in this article
Selected References
These references are in PubMed. This may not be the complete list of references from this article.
- Bader D., Masaki T., Fischman D. A. Immunochemical analysis of myosin heavy chain during avian myogenesis in vivo and in vitro. J Cell Biol. 1982 Dec;95(3):763–770. doi: 10.1083/jcb.95.3.763. [DOI] [PMC free article] [PubMed] [Google Scholar]
- Blackwell T. K., Weintraub H. Differences and similarities in DNA-binding preferences of MyoD and E2A protein complexes revealed by binding site selection. Science. 1990 Nov 23;250(4984):1104–1110. doi: 10.1126/science.2174572. [DOI] [PubMed] [Google Scholar]
- Bober E., Lyons G. E., Braun T., Cossu G., Buckingham M., Arnold H. H. The muscle regulatory gene, Myf-6, has a biphasic pattern of expression during early mouse development. J Cell Biol. 1991 Jun;113(6):1255–1265. doi: 10.1083/jcb.113.6.1255. [DOI] [PMC free article] [PubMed] [Google Scholar]
- Boxer L. M., Prywes R., Roeder R. G., Kedes L. The sarcomeric actin CArG-binding factor is indistinguishable from the c-fos serum response factor. Mol Cell Biol. 1989 Feb;9(2):515–522. doi: 10.1128/mcb.9.2.515. [DOI] [PMC free article] [PubMed] [Google Scholar]
- Braun T., Bober E., Buschhausen-Denker G., Kohtz S., Grzeschik K. H., Arnold H. H., Kotz S. Differential expression of myogenic determination genes in muscle cells: possible autoactivation by the Myf gene products. EMBO J. 1989 Dec 1;8(12):3617–3625. doi: 10.1002/j.1460-2075.1989.tb08535.x. [DOI] [PMC free article] [PubMed] [Google Scholar]
- Braun T., Bober E., Winter B., Rosenthal N., Arnold H. H. Myf-6, a new member of the human gene family of myogenic determination factors: evidence for a gene cluster on chromosome 12. EMBO J. 1990 Mar;9(3):821–831. doi: 10.1002/j.1460-2075.1990.tb08179.x. [DOI] [PMC free article] [PubMed] [Google Scholar]
- Braun T., Tannich E., Buschhausen-Denker G., Arnold H. H. Promoter upstream elements of the chicken cardiac myosin light-chain 2-A gene interact with trans-acting regulatory factors for muscle-specific transcription. Mol Cell Biol. 1989 Jun;9(6):2513–2525. doi: 10.1128/mcb.9.6.2513. [DOI] [PMC free article] [PubMed] [Google Scholar]
- Braun T., Winter B., Bober E., Arnold H. H. Transcriptional activation domain of the muscle-specific gene-regulatory protein myf5. Nature. 1990 Aug 16;346(6285):663–665. doi: 10.1038/346663a0. [DOI] [PubMed] [Google Scholar]
- Brennan T. J., Olson E. N. Myogenin resides in the nucleus and acquires high affinity for a conserved enhancer element on heterodimerization. Genes Dev. 1990 Apr;4(4):582–595. doi: 10.1101/gad.4.4.582. [DOI] [PubMed] [Google Scholar]
- Buskin J. N., Hauschka S. D. Identification of a myocyte nuclear factor that binds to the muscle-specific enhancer of the mouse muscle creatine kinase gene. Mol Cell Biol. 1989 Jun;9(6):2627–2640. doi: 10.1128/mcb.9.6.2627. [DOI] [PMC free article] [PubMed] [Google Scholar]
- Chakraborty T., Brennan T. J., Li L., Edmondson D., Olson E. N. Inefficient homooligomerization contributes to the dependence of myogenin on E2A products for efficient DNA binding. Mol Cell Biol. 1991 Jul;11(7):3633–3641. doi: 10.1128/mcb.11.7.3633. [DOI] [PMC free article] [PubMed] [Google Scholar]
- Chakraborty T., Brennan T., Olson E. Differential trans-activation of a muscle-specific enhancer by myogenic helix-loop-helix proteins is separable from DNA binding. J Biol Chem. 1991 Feb 15;266(5):2878–2882. [PubMed] [Google Scholar]
- Chamberlain J. S., Jaynes J. B., Hauschka S. D. Regulation of creatine kinase induction in differentiating mouse myoblasts. Mol Cell Biol. 1985 Mar;5(3):484–492. doi: 10.1128/mcb.5.3.484. [DOI] [PMC free article] [PubMed] [Google Scholar]
- Chomczynski P., Sacchi N. Single-step method of RNA isolation by acid guanidinium thiocyanate-phenol-chloroform extraction. Anal Biochem. 1987 Apr;162(1):156–159. doi: 10.1006/abio.1987.9999. [DOI] [PubMed] [Google Scholar]
- Chow K. L., Schwartz R. J. A combination of closely associated positive and negative cis-acting promoter elements regulates transcription of the skeletal alpha-actin gene. Mol Cell Biol. 1990 Feb;10(2):528–538. doi: 10.1128/mcb.10.2.528. [DOI] [PMC free article] [PubMed] [Google Scholar]
- Church G. M., Ephrussi A., Gilbert W., Tonegawa S. Cell-type-specific contacts to immunoglobulin enhancers in nuclei. 1985 Feb 28-Mar 6Nature. 313(6005):798–801. doi: 10.1038/313798a0. [DOI] [PubMed] [Google Scholar]
- Clegg C. H., Linkhart T. A., Olwin B. B., Hauschka S. D. Growth factor control of skeletal muscle differentiation: commitment to terminal differentiation occurs in G1 phase and is repressed by fibroblast growth factor. J Cell Biol. 1987 Aug;105(2):949–956. doi: 10.1083/jcb.105.2.949. [DOI] [PMC free article] [PubMed] [Google Scholar]
- Cserjesi P., Lilly B., Bryson L., Wang Y., Sassoon D. A., Olson E. N. MHox: a mesodermally restricted homeodomain protein that binds an essential site in the muscle creatine kinase enhancer. Development. 1992 Aug;115(4):1087–1101. doi: 10.1242/dev.115.4.1087. [DOI] [PubMed] [Google Scholar]
- Cserjesi P., Olson E. N. Myogenin induces the myocyte-specific enhancer binding factor MEF-2 independently of other muscle-specific gene products. Mol Cell Biol. 1991 Oct;11(10):4854–4862. doi: 10.1128/mcb.11.10.4854. [DOI] [PMC free article] [PubMed] [Google Scholar]
- Dalton S., Treisman R. Characterization of SAP-1, a protein recruited by serum response factor to the c-fos serum response element. Cell. 1992 Feb 7;68(3):597–612. doi: 10.1016/0092-8674(92)90194-h. [DOI] [PubMed] [Google Scholar]
- Dugaiczyk A., Haron J. A., Stone E. M., Dennison O. E., Rothblum K. N., Schwartz R. J. Cloning and sequencing of a deoxyribonucleic acid copy of glyceraldehyde-3-phosphate dehydrogenase messenger ribonucleic acid isolated from chicken muscle. Biochemistry. 1983 Mar 29;22(7):1605–1613. doi: 10.1021/bi00276a013. [DOI] [PubMed] [Google Scholar]
- Edmondson D. G., Olson E. N. A gene with homology to the myc similarity region of MyoD1 is expressed during myogenesis and is sufficient to activate the muscle differentiation program. Genes Dev. 1989 May;3(5):628–640. doi: 10.1101/gad.3.5.628. [DOI] [PubMed] [Google Scholar]
- Ephrussi A., Church G. M., Tonegawa S., Gilbert W. B lineage--specific interactions of an immunoglobulin enhancer with cellular factors in vivo. Science. 1985 Jan 11;227(4683):134–140. doi: 10.1126/science.3917574. [DOI] [PubMed] [Google Scholar]
- Ernst H., Walsh K., Harrison C. A., Rosenthal N. The myosin light chain enhancer and the skeletal actin promoter share a binding site for factors involved in muscle-specific gene expression. Mol Cell Biol. 1991 Jul;11(7):3735–3744. doi: 10.1128/mcb.11.7.3735. [DOI] [PMC free article] [PubMed] [Google Scholar]
- Farrance I. K., Mar J. H., Ordahl C. P. M-CAT binding factor is related to the SV40 enhancer binding factor, TEF-1. J Biol Chem. 1992 Aug 25;267(24):17234–17240. [PubMed] [Google Scholar]
- Flink I. L., Edwards J. G., Bahl J. J., Liew C. C., Sole M., Morkin E. Characterization of a strong positive cis-acting element of the human beta-myosin heavy chain gene in fetal rat heart cells. J Biol Chem. 1992 May 15;267(14):9917–9924. [PubMed] [Google Scholar]
- Gorman C. M., Moffat L. F., Howard B. H. Recombinant genomes which express chloramphenicol acetyltransferase in mammalian cells. Mol Cell Biol. 1982 Sep;2(9):1044–1051. doi: 10.1128/mcb.2.9.1044. [DOI] [PMC free article] [PubMed] [Google Scholar]
- Gossett L. A., Kelvin D. J., Sternberg E. A., Olson E. N. A new myocyte-specific enhancer-binding factor that recognizes a conserved element associated with multiple muscle-specific genes. Mol Cell Biol. 1989 Nov;9(11):5022–5033. doi: 10.1128/mcb.9.11.5022. [DOI] [PMC free article] [PubMed] [Google Scholar]
- Henthorn P., Zervos P., Raducha M., Harris H., Kadesch T. Expression of a human placental alkaline phosphatase gene in transfected cells: use as a reporter for studies of gene expression. Proc Natl Acad Sci U S A. 1988 Sep;85(17):6342–6346. doi: 10.1073/pnas.85.17.6342. [DOI] [PMC free article] [PubMed] [Google Scholar]
- Horlick R. A., Benfield P. A. The upstream muscle-specific enhancer of the rat muscle creatine kinase gene is composed of multiple elements. Mol Cell Biol. 1989 Jun;9(6):2396–2413. doi: 10.1128/mcb.9.6.2396. [DOI] [PMC free article] [PubMed] [Google Scholar]
- Horlick R. A., Hobson G. M., Patterson J. H., Mitchell M. T., Benfield P. A. Brain and muscle creatine kinase genes contain common TA-rich recognition protein-binding regulatory elements. Mol Cell Biol. 1990 Sep;10(9):4826–4836. doi: 10.1128/mcb.10.9.4826. [DOI] [PMC free article] [PubMed] [Google Scholar]
- Iannello R. C., Mar J. H., Ordahl C. P. Characterization of a promoter element required for transcription in myocardial cells. J Biol Chem. 1991 Feb 15;266(5):3309–3316. [PubMed] [Google Scholar]
- Imagawa M., Chiu R., Karin M. Transcription factor AP-2 mediates induction by two different signal-transduction pathways: protein kinase C and cAMP. Cell. 1987 Oct 23;51(2):251–260. doi: 10.1016/0092-8674(87)90152-8. [DOI] [PubMed] [Google Scholar]
- Iwaki K., Sukhatme V. P., Shubeita H. E., Chien K. R. Alpha- and beta-adrenergic stimulation induces distinct patterns of immediate early gene expression in neonatal rat myocardial cells. fos/jun expression is associated with sarcomere assembly; Egr-1 induction is primarily an alpha 1-mediated response. J Biol Chem. 1990 Aug 15;265(23):13809–13817. [PubMed] [Google Scholar]
- Jaynes J. B., Chamberlain J. S., Buskin J. N., Johnson J. E., Hauschka S. D. Transcriptional regulation of the muscle creatine kinase gene and regulated expression in transfected mouse myoblasts. Mol Cell Biol. 1986 Aug;6(8):2855–2864. doi: 10.1128/mcb.6.8.2855. [DOI] [PMC free article] [PubMed] [Google Scholar]
- Jaynes J. B., Johnson J. E., Buskin J. N., Gartside C. L., Hauschka S. D. The muscle creatine kinase gene is regulated by multiple upstream elements, including a muscle-specific enhancer. Mol Cell Biol. 1988 Jan;8(1):62–70. doi: 10.1128/mcb.8.1.62. [DOI] [PMC free article] [PubMed] [Google Scholar]
- Johnson J. E., Gartside C. L., Jaynes J. B., Hauschka S. D. Expression of a transfected mouse muscle-creatine kinase gene is induced upon growth factor deprivation of myogenic but not of nonmyogenic cells. Dev Biol. 1989 Jul;134(1):258–262. doi: 10.1016/0012-1606(89)90095-x. [DOI] [PubMed] [Google Scholar]
- Johnson J. E., Wold B. J., Hauschka S. D. Muscle creatine kinase sequence elements regulating skeletal and cardiac muscle expression in transgenic mice. Mol Cell Biol. 1989 Aug;9(8):3393–3399. doi: 10.1128/mcb.9.8.3393. [DOI] [PMC free article] [PubMed] [Google Scholar]
- Kawamoto T., Makino K., Niwa H., Sugiyama H., Kimura S., Amemura M., Nakata A., Kakunaga T. Identification of the human beta-actin enhancer and its binding factor. Mol Cell Biol. 1988 Jan;8(1):267–272. doi: 10.1128/mcb.8.1.267. [DOI] [PMC free article] [PubMed] [Google Scholar]
- Kunkel T. A., Roberts J. D., Zakour R. A. Rapid and efficient site-specific mutagenesis without phenotypic selection. Methods Enzymol. 1987;154:367–382. doi: 10.1016/0076-6879(87)54085-x. [DOI] [PubMed] [Google Scholar]
- Lassar A. B., Buskin J. N., Lockshon D., Davis R. L., Apone S., Hauschka S. D., Weintraub H. MyoD is a sequence-specific DNA binding protein requiring a region of myc homology to bind to the muscle creatine kinase enhancer. Cell. 1989 Sep 8;58(5):823–831. doi: 10.1016/0092-8674(89)90935-5. [DOI] [PubMed] [Google Scholar]
- Lee T. C., Chow K. L., Fang P., Schwartz R. J. Activation of skeletal alpha-actin gene transcription: the cooperative formation of serum response factor-binding complexes over positive cis-acting promoter serum response elements displaces a negative-acting nuclear factor enriched in replicating myoblasts and nonmyogenic cells. Mol Cell Biol. 1991 Oct;11(10):5090–5100. doi: 10.1128/mcb.11.10.5090. [DOI] [PMC free article] [PubMed] [Google Scholar]
- Lin H., Yutzey K. E., Konieczny S. F. Muscle-specific expression of the troponin I gene requires interactions between helix-loop-helix muscle regulatory factors and ubiquitous transcription factors. Mol Cell Biol. 1991 Jan;11(1):267–280. doi: 10.1128/mcb.11.1.267. [DOI] [PMC free article] [PubMed] [Google Scholar]
- Lyons G. E., Mühlebach S., Moser A., Masood R., Paterson B. M., Buckingham M. E., Perriard J. C. Developmental regulation of creatine kinase gene expression by myogenic factors in embryonic mouse and chick skeletal muscle. Development. 1991 Nov;113(3):1017–1029. doi: 10.1242/dev.113.3.1017. [DOI] [PubMed] [Google Scholar]
- Mar J. H., Antin P. B., Cooper T. A., Ordahl C. P. Analysis of the upstream regions governing expression of the chicken cardiac troponin T gene in embryonic cardiac and skeletal muscle cells. J Cell Biol. 1988 Aug;107(2):573–585. doi: 10.1083/jcb.107.2.573. [DOI] [PMC free article] [PubMed] [Google Scholar]
- Mar J. H., Ordahl C. P. A conserved CATTCCT motif is required for skeletal muscle-specific activity of the cardiac troponin T gene promoter. Proc Natl Acad Sci U S A. 1988 Sep;85(17):6404–6408. doi: 10.1073/pnas.85.17.6404. [DOI] [PMC free article] [PubMed] [Google Scholar]
- Mar J. H., Ordahl C. P. M-CAT binding factor, a novel trans-acting factor governing muscle-specific transcription. Mol Cell Biol. 1990 Aug;10(8):4271–4283. doi: 10.1128/mcb.10.8.4271. [DOI] [PMC free article] [PubMed] [Google Scholar]
- Miksicek R., Heber A., Schmid W., Danesch U., Posseckert G., Beato M., Schütz G. Glucocorticoid responsiveness of the transcriptional enhancer of Moloney murine sarcoma virus. Cell. 1986 Jul 18;46(2):283–290. doi: 10.1016/0092-8674(86)90745-2. [DOI] [PubMed] [Google Scholar]
- Minty A., Kedes L. Upstream regions of the human cardiac actin gene that modulate its transcription in muscle cells: presence of an evolutionarily conserved repeated motif. Mol Cell Biol. 1986 Jun;6(6):2125–2136. doi: 10.1128/mcb.6.6.2125. [DOI] [PMC free article] [PubMed] [Google Scholar]
- Mitchell P. J., Timmons P. M., Hébert J. M., Rigby P. W., Tjian R. Transcription factor AP-2 is expressed in neural crest cell lineages during mouse embryogenesis. Genes Dev. 1991 Jan;5(1):105–119. doi: 10.1101/gad.5.1.105. [DOI] [PubMed] [Google Scholar]
- Mitchell P. J., Wang C., Tjian R. Positive and negative regulation of transcription in vitro: enhancer-binding protein AP-2 is inhibited by SV40 T antigen. Cell. 1987 Sep 11;50(6):847–861. doi: 10.1016/0092-8674(87)90512-5. [DOI] [PubMed] [Google Scholar]
- Miwa T., Kedes L. Duplicated CArG box domains have positive and mutually dependent regulatory roles in expression of the human alpha-cardiac actin gene. Mol Cell Biol. 1987 Aug;7(8):2803–2813. doi: 10.1128/mcb.7.8.2803. [DOI] [PMC free article] [PubMed] [Google Scholar]
- Mohun T. J., Chambers A. E., Towers N., Taylor M. V. Expression of genes encoding the transcription factor SRF during early development of Xenopus laevis: identification of a CArG box-binding activity as SRF. EMBO J. 1991 Apr;10(4):933–940. doi: 10.1002/j.1460-2075.1991.tb08027.x. [DOI] [PMC free article] [PubMed] [Google Scholar]
- Mohun T. J., Taylor M. V., Garrett N., Gurdon J. B. The CArG promoter sequence is necessary for muscle-specific transcription of the cardiac actin gene in Xenopus embryos. EMBO J. 1989 Apr;8(4):1153–1161. doi: 10.1002/j.1460-2075.1989.tb03486.x. [DOI] [PMC free article] [PubMed] [Google Scholar]
- Mohun T., Garrett N., Treisman R. Xenopus cytoskeletal actin and human c-fos gene promoters share a conserved protein-binding site. EMBO J. 1987 Mar;6(3):667–673. doi: 10.1002/j.1460-2075.1987.tb04806.x. [DOI] [PMC free article] [PubMed] [Google Scholar]
- Mueller C. G., Nordheim A. A protein domain conserved between yeast MCM1 and human SRF directs ternary complex formation. EMBO J. 1991 Dec;10(13):4219–4229. doi: 10.1002/j.1460-2075.1991.tb05000.x. [DOI] [PMC free article] [PubMed] [Google Scholar]
- Mueller P. R., Wold B. In vivo footprinting of a muscle specific enhancer by ligation mediated PCR. Science. 1989 Nov 10;246(4931):780–786. doi: 10.1126/science.2814500. [DOI] [PubMed] [Google Scholar]
- Murre C., McCaw P. S., Baltimore D. A new DNA binding and dimerization motif in immunoglobulin enhancer binding, daughterless, MyoD, and myc proteins. Cell. 1989 Mar 10;56(5):777–783. doi: 10.1016/0092-8674(89)90682-x. [DOI] [PubMed] [Google Scholar]
- Murre C., McCaw P. S., Vaessin H., Caudy M., Jan L. Y., Jan Y. N., Cabrera C. V., Buskin J. N., Hauschka S. D., Lassar A. B. Interactions between heterologous helix-loop-helix proteins generate complexes that bind specifically to a common DNA sequence. Cell. 1989 Aug 11;58(3):537–544. doi: 10.1016/0092-8674(89)90434-0. [DOI] [PubMed] [Google Scholar]
- Muscat G. E., Gustafson T. A., Kedes L. A common factor regulates skeletal and cardiac alpha-actin gene transcription in muscle. Mol Cell Biol. 1988 Oct;8(10):4120–4133. doi: 10.1128/mcb.8.10.4120. [DOI] [PMC free article] [PubMed] [Google Scholar]
- Nakatsuji Y., Hidaka K., Tsujino S., Yamamoto Y., Mukai T., Yanagihara T., Kishimoto T., Sakoda S. A single MEF-2 site is a major positive regulatory element required for transcription of the muscle-specific subunit of the human phosphoglycerate mutase gene in skeletal and cardiac muscle cells. Mol Cell Biol. 1992 Oct;12(10):4384–4390. doi: 10.1128/mcb.12.10.4384. [DOI] [PMC free article] [PubMed] [Google Scholar]
- Navankasattusas S., Zhu H., Garcia A. V., Evans S. M., Chien K. R. A ubiquitous factor (HF-1a) and a distinct muscle factor (HF-1b/MEF-2) form an E-box-independent pathway for cardiac muscle gene expression. Mol Cell Biol. 1992 Apr;12(4):1469–1479. doi: 10.1128/mcb.12.4.1469. [DOI] [PMC free article] [PubMed] [Google Scholar]
- Ng S. Y., Gunning P., Eddy R., Ponte P., Leavitt J., Shows T., Kedes L. Evolution of the functional human beta-actin gene and its multi-pseudogene family: conservation of noncoding regions and chromosomal dispersion of pseudogenes. Mol Cell Biol. 1985 Oct;5(10):2720–2732. doi: 10.1128/mcb.5.10.2720. [DOI] [PMC free article] [PubMed] [Google Scholar]
- Norman C., Runswick M., Pollock R., Treisman R. Isolation and properties of cDNA clones encoding SRF, a transcription factor that binds to the c-fos serum response element. Cell. 1988 Dec 23;55(6):989–1003. doi: 10.1016/0092-8674(88)90244-9. [DOI] [PubMed] [Google Scholar]
- Ott M. O., Bober E., Lyons G., Arnold H., Buckingham M. Early expression of the myogenic regulatory gene, myf-5, in precursor cells of skeletal muscle in the mouse embryo. Development. 1991 Apr;111(4):1097–1107. doi: 10.1242/dev.111.4.1097. [DOI] [PubMed] [Google Scholar]
- Pari G., Jardine K., McBurney M. W. Multiple CArG boxes in the human cardiac actin gene promoter required for expression in embryonic cardiac muscle cells developing in vitro from embryonal carcinoma cells. Mol Cell Biol. 1991 Sep;11(9):4796–4803. doi: 10.1128/mcb.11.9.4796. [DOI] [PMC free article] [PubMed] [Google Scholar]
- Parmacek M. S., Vora A. J., Shen T., Barr E., Jung F., Leiden J. M. Identification and characterization of a cardiac-specific transcriptional regulatory element in the slow/cardiac troponin C gene. Mol Cell Biol. 1992 May;12(5):1967–1976. doi: 10.1128/mcb.12.5.1967. [DOI] [PMC free article] [PubMed] [Google Scholar]
- Piette J., Bessereau J. L., Huchet M., Changeux J. P. Two adjacent MyoD1-binding sites regulate expression of the acetylcholine receptor alpha-subunit gene. Nature. 1990 May 24;345(6273):353–355. doi: 10.1038/345353a0. [DOI] [PubMed] [Google Scholar]
- Pollock R., Treisman R. Human SRF-related proteins: DNA-binding properties and potential regulatory targets. Genes Dev. 1991 Dec;5(12A):2327–2341. doi: 10.1101/gad.5.12a.2327. [DOI] [PubMed] [Google Scholar]
- Sanger F. Determination of nucleotide sequences in DNA. Science. 1981 Dec 11;214(4526):1205–1210. doi: 10.1126/science.7302589. [DOI] [PubMed] [Google Scholar]
- Santoro I. M., Walsh K. Natural and synthetic DNA elements with the CArG motif differ in expression and protein-binding properties. Mol Cell Biol. 1991 Dec;11(12):6296–6305. doi: 10.1128/mcb.11.12.6296. [DOI] [PMC free article] [PubMed] [Google Scholar]
- Sartorelli V., Hong N. A., Bishopric N. H., Kedes L. Myocardial activation of the human cardiac alpha-actin promoter by helix-loop-helix proteins. Proc Natl Acad Sci U S A. 1992 May 1;89(9):4047–4051. doi: 10.1073/pnas.89.9.4047. [DOI] [PMC free article] [PubMed] [Google Scholar]
- Sassoon D., Lyons G., Wright W. E., Lin V., Lassar A., Weintraub H., Buckingham M. Expression of two myogenic regulatory factors myogenin and MyoD1 during mouse embryogenesis. Nature. 1989 Sep 28;341(6240):303–307. doi: 10.1038/341303a0. [DOI] [PubMed] [Google Scholar]
- Shaw P. E., Schröter H., Nordheim A. The ability of a ternary complex to form over the serum response element correlates with serum inducibility of the human c-fos promoter. Cell. 1989 Feb 24;56(4):563–572. doi: 10.1016/0092-8674(89)90579-5. [DOI] [PubMed] [Google Scholar]
- Sternberg E. A., Spizz G., Perry W. M., Vizard D., Weil T., Olson E. N. Identification of upstream and intragenic regulatory elements that confer cell-type-restricted and differentiation-specific expression on the muscle creatine kinase gene. Mol Cell Biol. 1988 Jul;8(7):2896–2909. doi: 10.1128/mcb.8.7.2896. [DOI] [PMC free article] [PubMed] [Google Scholar]
- Takeda S., North D. L., Lakich M. M., Russell S. D., Whalen R. G. A possible regulatory role for conserved promoter motifs in an adult-specific muscle myosin gene from mouse. J Biol Chem. 1992 Aug 25;267(24):16957–16967. [PubMed] [Google Scholar]
- Taylor M., Treisman R., Garrett N., Mohun T. Muscle-specific (CArG) and serum-responsive (SRE) promoter elements are functionally interchangeable in Xenopus embryos and mouse fibroblasts. Development. 1989 May;106(1):67–78. doi: 10.1242/dev.106.1.67. [DOI] [PubMed] [Google Scholar]
- Thompson W. R., Nadal-Ginard B., Mahdavi V. A MyoD1-independent muscle-specific enhancer controls the expression of the beta-myosin heavy chain gene in skeletal and cardiac muscle cells. J Biol Chem. 1991 Nov 25;266(33):22678–22688. [PubMed] [Google Scholar]
- Trask R. V., Strauss A. W., Billadello J. J. Developmental regulation and tissue-specific expression of the human muscle creatine kinase gene. J Biol Chem. 1988 Nov 15;263(32):17142–17149. [PubMed] [Google Scholar]
- Treisman R. Identification of a protein-binding site that mediates transcriptional response of the c-fos gene to serum factors. Cell. 1986 Aug 15;46(4):567–574. doi: 10.1016/0092-8674(86)90882-2. [DOI] [PubMed] [Google Scholar]
- Tuil D., Clergue N., Montarras D., Pinset C., Kahn A., Phan-Dinh-Tuy F. CC Ar GG boxes, cis-acting elements with a dual specificity. Muscle-specific transcriptional activation and serum responsiveness. J Mol Biol. 1990 Jun 20;213(4):677–686. doi: 10.1016/S0022-2836(05)80255-4. [DOI] [PubMed] [Google Scholar]
- Vieira J., Messing J. Production of single-stranded plasmid DNA. Methods Enzymol. 1987;153:3–11. doi: 10.1016/0076-6879(87)53044-0. [DOI] [PubMed] [Google Scholar]
- Walsh K. Cross-binding of factors to functionally different promoter elements in c-fos and skeletal actin genes. Mol Cell Biol. 1989 May;9(5):2191–2201. doi: 10.1128/mcb.9.5.2191. [DOI] [PMC free article] [PubMed] [Google Scholar]
- Walsh K., Schimmel P. DNA-binding site for two skeletal actin promoter factors is important for expression in muscle cells. Mol Cell Biol. 1988 Apr;8(4):1800–1802. doi: 10.1128/mcb.8.4.1800. [DOI] [PMC free article] [PubMed] [Google Scholar]
- Walsh K., Schimmel P. Two nuclear factors compete for the skeletal muscle actin promoter. J Biol Chem. 1987 Jul 15;262(20):9429–9432. [PubMed] [Google Scholar]
- Weintraub H., Davis R., Lockshon D., Lassar A. MyoD binds cooperatively to two sites in a target enhancer sequence: occupancy of two sites is required for activation. Proc Natl Acad Sci U S A. 1990 Aug;87(15):5623–5627. doi: 10.1073/pnas.87.15.5623. [DOI] [PMC free article] [PubMed] [Google Scholar]
- Wentworth B. M., Donoghue M., Engert J. C., Berglund E. B., Rosenthal N. Paired MyoD-binding sites regulate myosin light chain gene expression. Proc Natl Acad Sci U S A. 1991 Feb 15;88(4):1242–1246. doi: 10.1073/pnas.88.4.1242. [DOI] [PMC free article] [PubMed] [Google Scholar]
- Wright W. E., Binder M., Funk W. Cyclic amplification and selection of targets (CASTing) for the myogenin consensus binding site. Mol Cell Biol. 1991 Aug;11(8):4104–4110. doi: 10.1128/mcb.11.8.4104. [DOI] [PMC free article] [PubMed] [Google Scholar]
- Wu J. P., Kovacic-Milivojević B., Lapointe M. C., Nakamura K., Gardner D. G. Cis-active determinants of cardiac-specific expression in the human atrial natriuretic peptide gene. Mol Endocrinol. 1991 Sep;5(9):1311–1322. doi: 10.1210/mend-5-9-1311. [DOI] [PubMed] [Google Scholar]
- Yi T. M., Walsh K., Schimmel P. Rabbit muscle creatine kinase: genomic cloning, sequencing, and analysis of upstream sequences important for expression in myocytes. Nucleic Acids Res. 1991 Jun 11;19(11):3027–3033. doi: 10.1093/nar/19.11.3027. [DOI] [PMC free article] [PubMed] [Google Scholar]
- Yu Y. T., Breitbart R. E., Smoot L. B., Lee Y., Mahdavi V., Nadal-Ginard B. Human myocyte-specific enhancer factor 2 comprises a group of tissue-restricted MADS box transcription factors. Genes Dev. 1992 Sep;6(9):1783–1798. doi: 10.1101/gad.6.9.1783. [DOI] [PubMed] [Google Scholar]
- Yutzey K. E., Rhodes S. J., Konieczny S. F. Differential trans activation associated with the muscle regulatory factors MyoD1, myogenin, and MRF4. Mol Cell Biol. 1990 Aug;10(8):3934–3944. doi: 10.1128/mcb.10.8.3934. [DOI] [PMC free article] [PubMed] [Google Scholar]
- Zhu H., Garcia A. V., Ross R. S., Evans S. M., Chien K. R. A conserved 28-base-pair element (HF-1) in the rat cardiac myosin light-chain-2 gene confers cardiac-specific and alpha-adrenergic-inducible expression in cultured neonatal rat myocardial cells. Mol Cell Biol. 1991 Apr;11(4):2273–2281. doi: 10.1128/mcb.11.4.2273. [DOI] [PMC free article] [PubMed] [Google Scholar]
- Zoller M. J., Smith M. Oligonucleotide-directed mutagenesis: a simple method using two oligonucleotide primers and a single-stranded DNA template. Methods Enzymol. 1987;154:329–350. doi: 10.1016/0076-6879(87)54083-6. [DOI] [PubMed] [Google Scholar]