Abstract
The Cdc2 protein kinase requires cyclin binding for activity and also binds to a small protein, Suc1. Charged-to-alanine scanning mutagenesis of Cdc2 was used previously to localize cyclin A- and B- and Suc1-binding sites (B. Ducommun, P. Brambilla, and G. Draetta, Mol. Cell. Biol. 11:6177-6184, 1991). Those sites were mapped by building a Cdc2 model based on the crystallographic coordinates of the catalytic subunit of cyclic AMP-dependent protein kinase (cAPK) (D. R. Knighton, J. Zheng, L. F. Ten Eyck, V. A. Ashford, N.-H. Xuong, S. S. Taylor, and J. M. Sowadski, Science 253:407-414, 1991). On the basis of this model, additional mutations were made and tested for cyclin A and Suc1 binding and for kinase activity. Mutations that interfere with cyclin A binding are localized primarily on the small lobe near its interface with the cleft and include an acidic patch on the B helix and R-50 in the highly conserved PSTAIRE sequence. Two residues in the large lobe, R-151 and T-161, influence cyclin binding, and both are at the surface of the cleft near its interface with the PSTAIRE motif. Cyclin-dependent phosphorylation of T-161 in Cdc2 is essential for activation, and the model provides insights into the importance of this site. T-161 is equivalent to T-197, a stable phosphorylation site in cAPK. On the basis of the model, cyclin binding very likely alters the surface surrounding T-161 to allow for T-161 phosphorylation. The two major ligands to T-197 in cAPK are conserved as R-127 and R-151 in Cdc2. The equivalent of the third ligand, H-87, is T-47 in the PSTAIRE sequence motif. Once phosphorylated, T-161 is predicted to play a major structural role in Cdc2, comparable to that of T-197 in cAPK, by assembling the active conformation required for peptide recognition. The inhibitory phosphorylation at Y-15 also comes close to the cleft interface and on the basis of this model would disrupt the cleft interface and the adjacent peptide recognition site rather than prevent ATP binding. In contrast to cyclin A, both lobes influence Suc1 binding; however, the Suc1-binding sites are far from the active site. Several mutants map to the surface in cAPK, which is masked in part by the N-terminal 40 residues that lie outside the conserved catalytic core. The other Suc1-binding site maps to the large lobe near a 25-residue insert and includes R-215.
Full text
PDF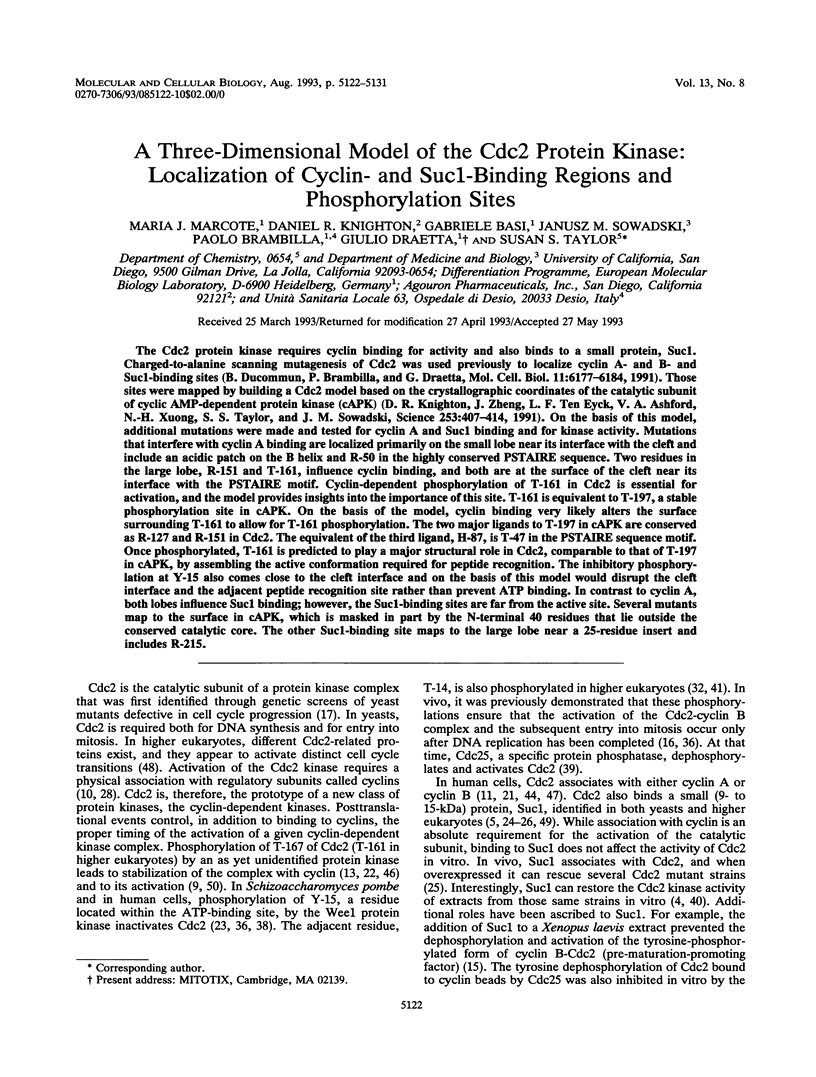
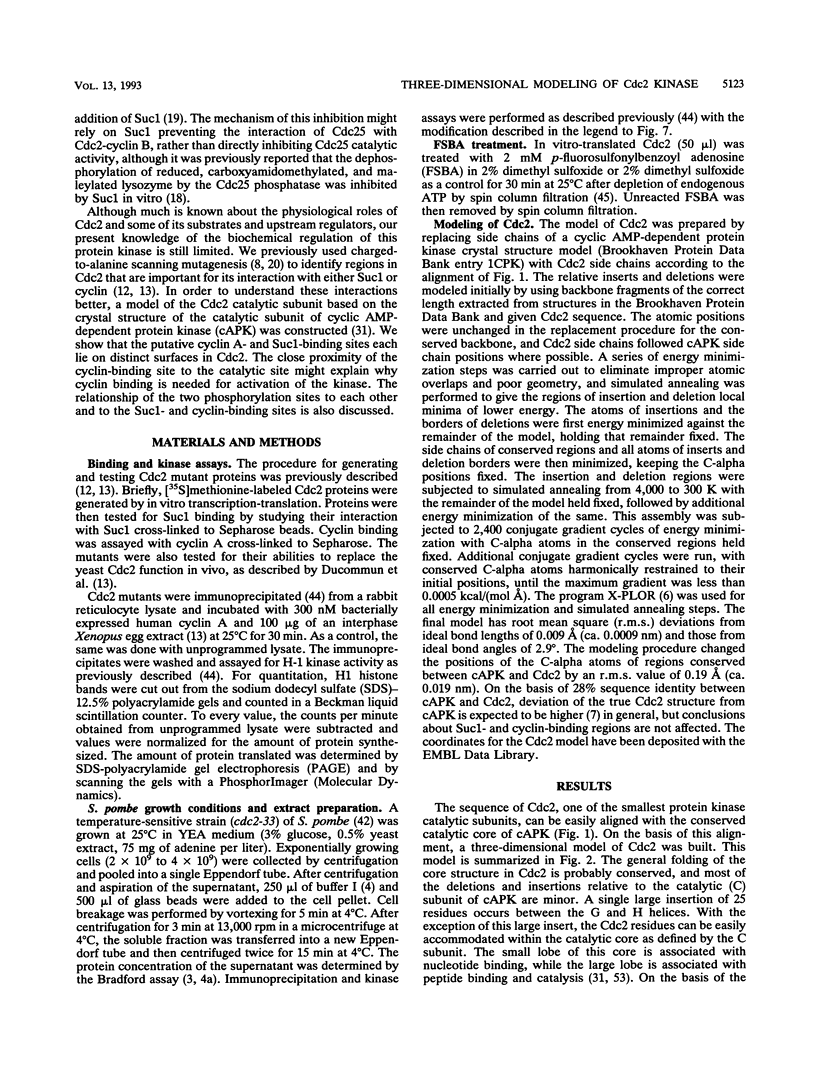
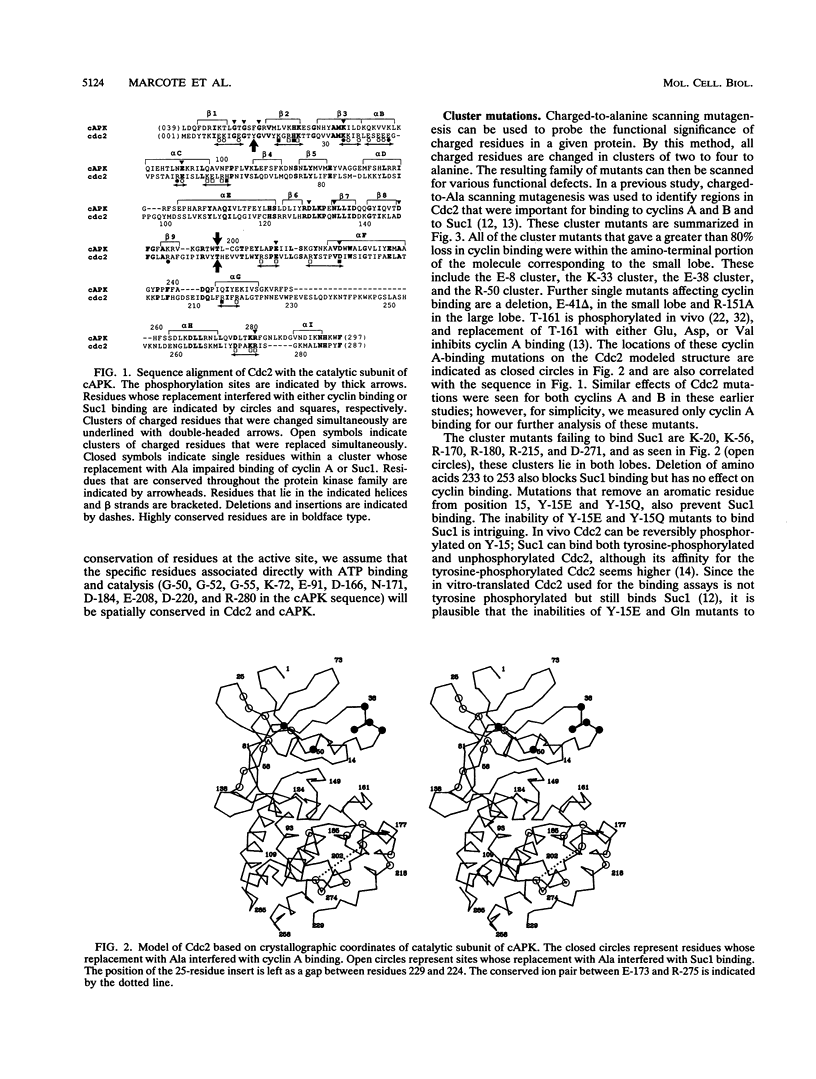
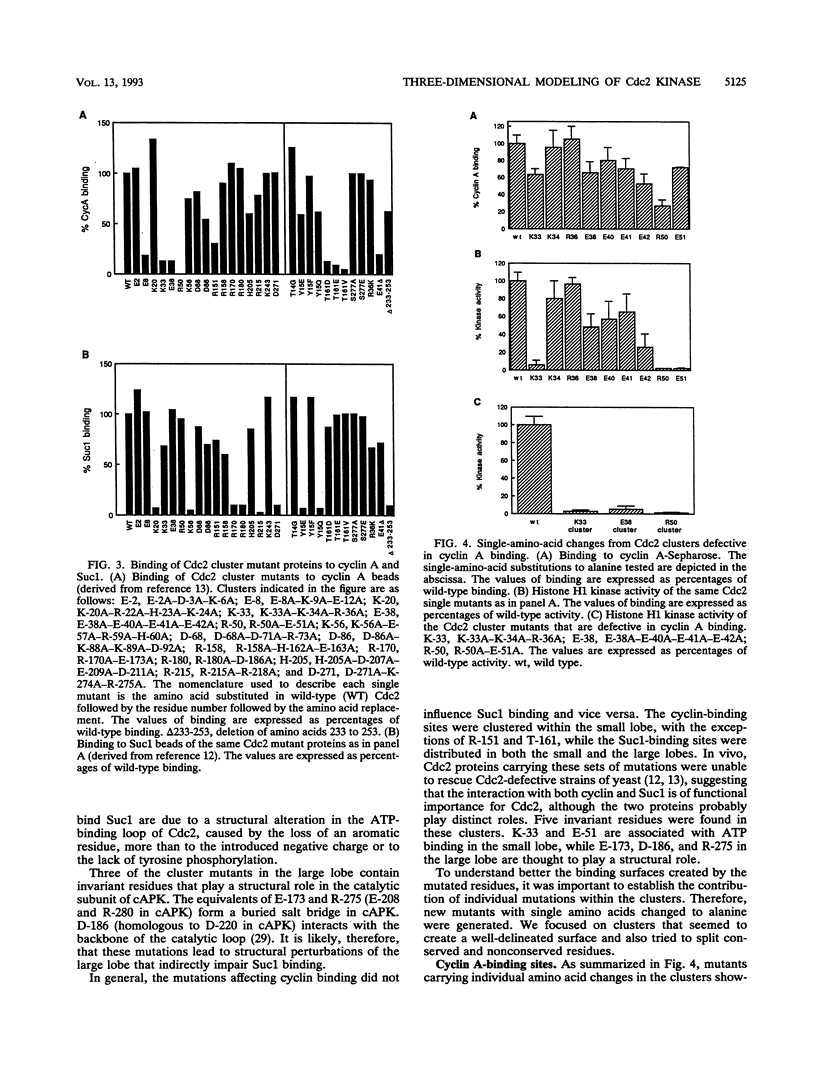
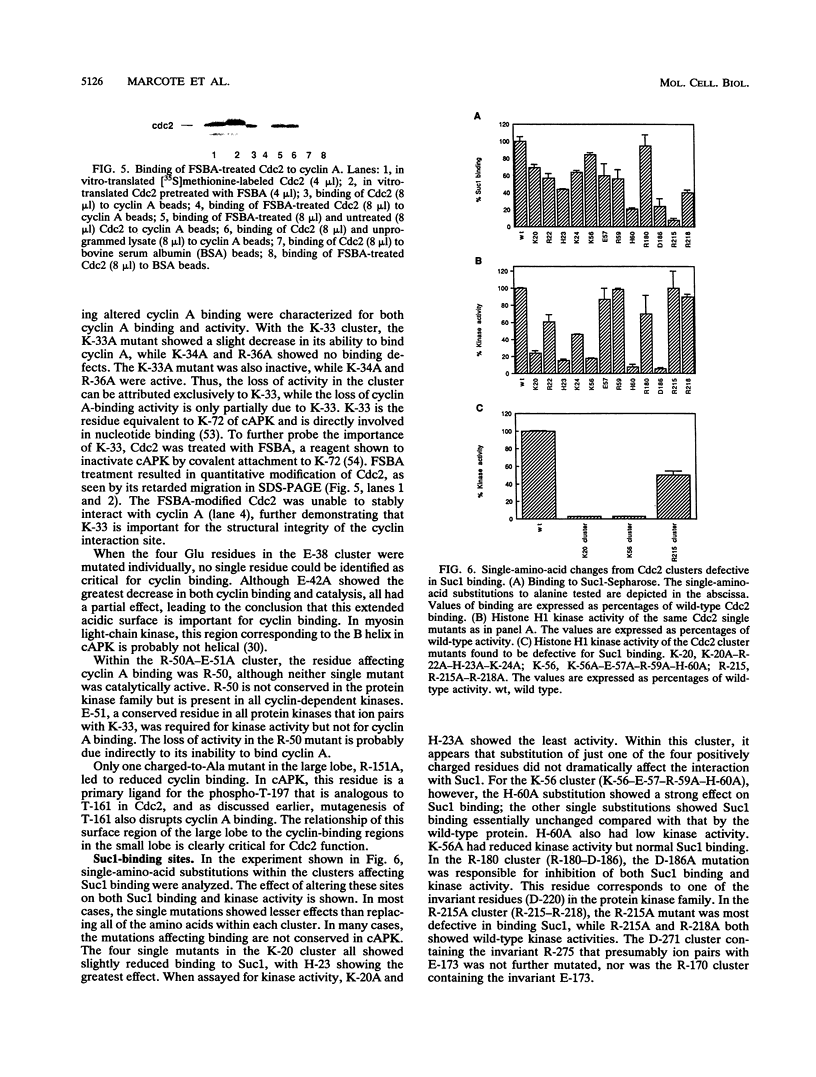
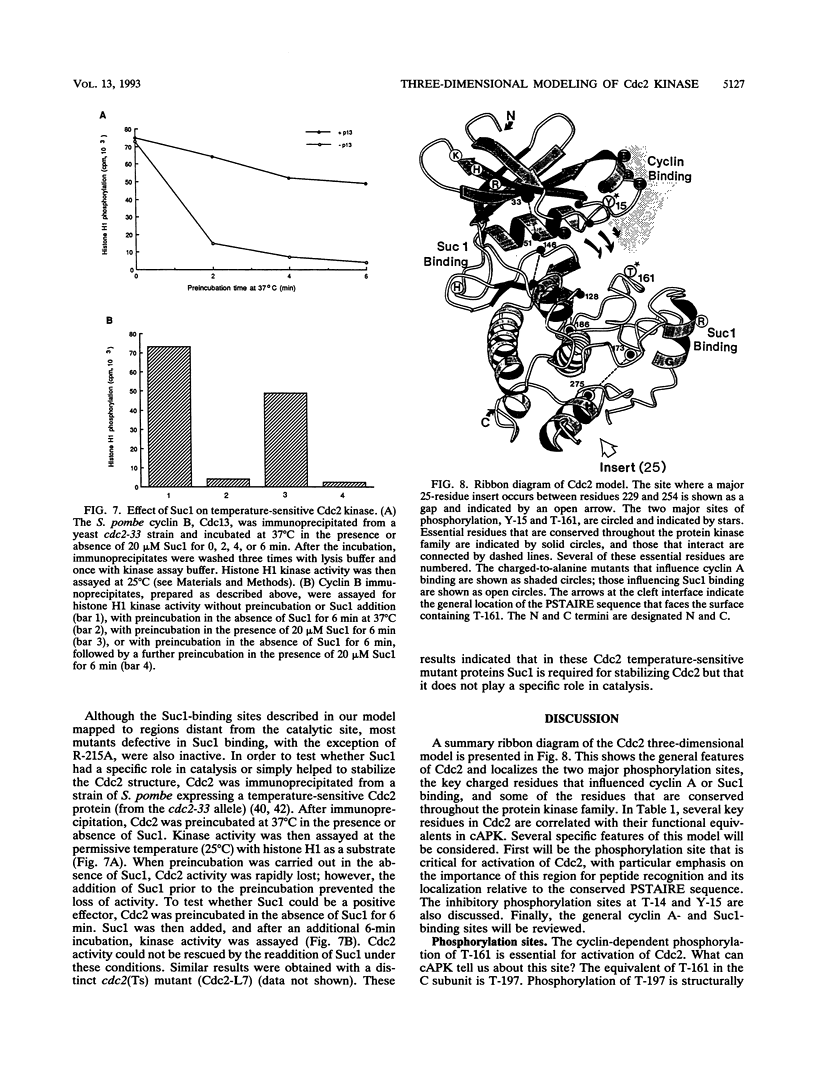
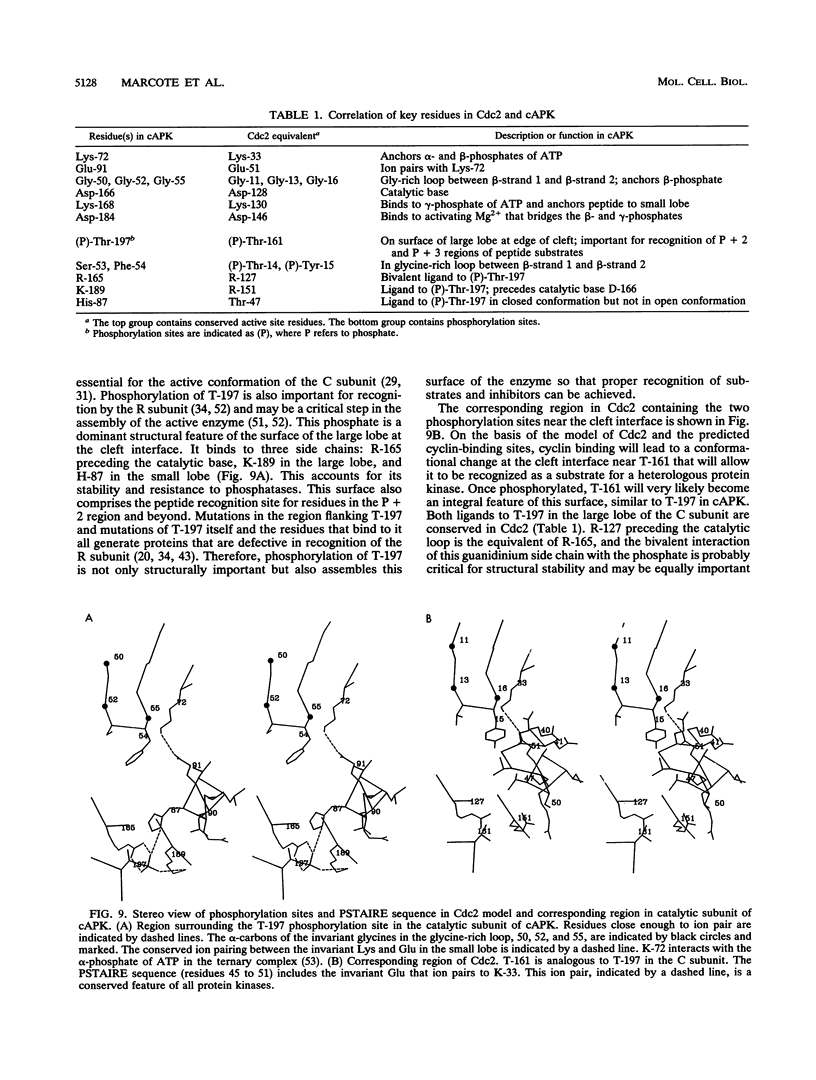
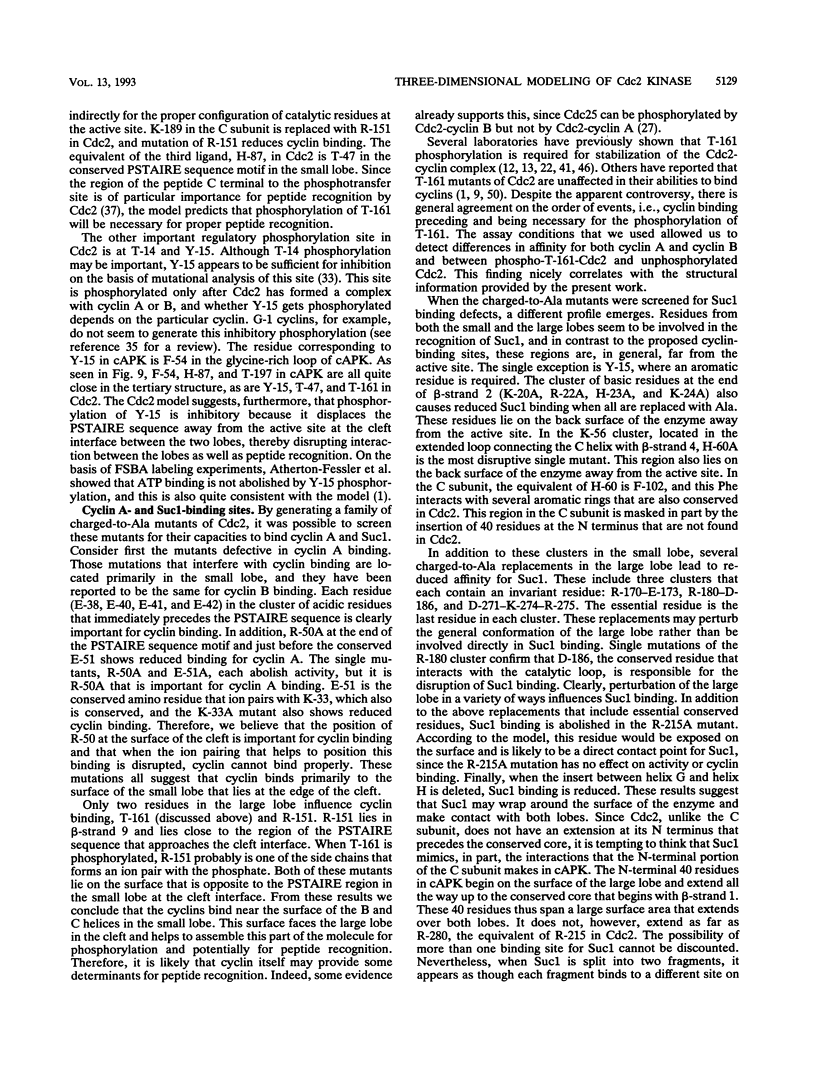
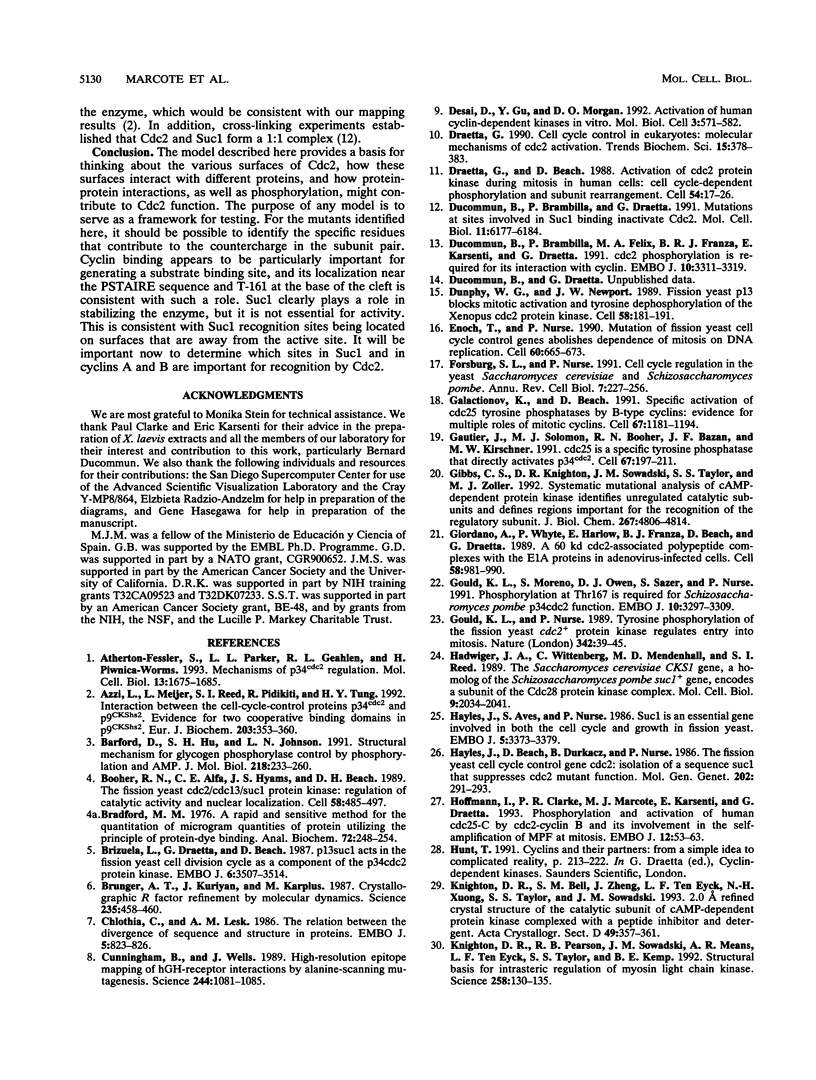
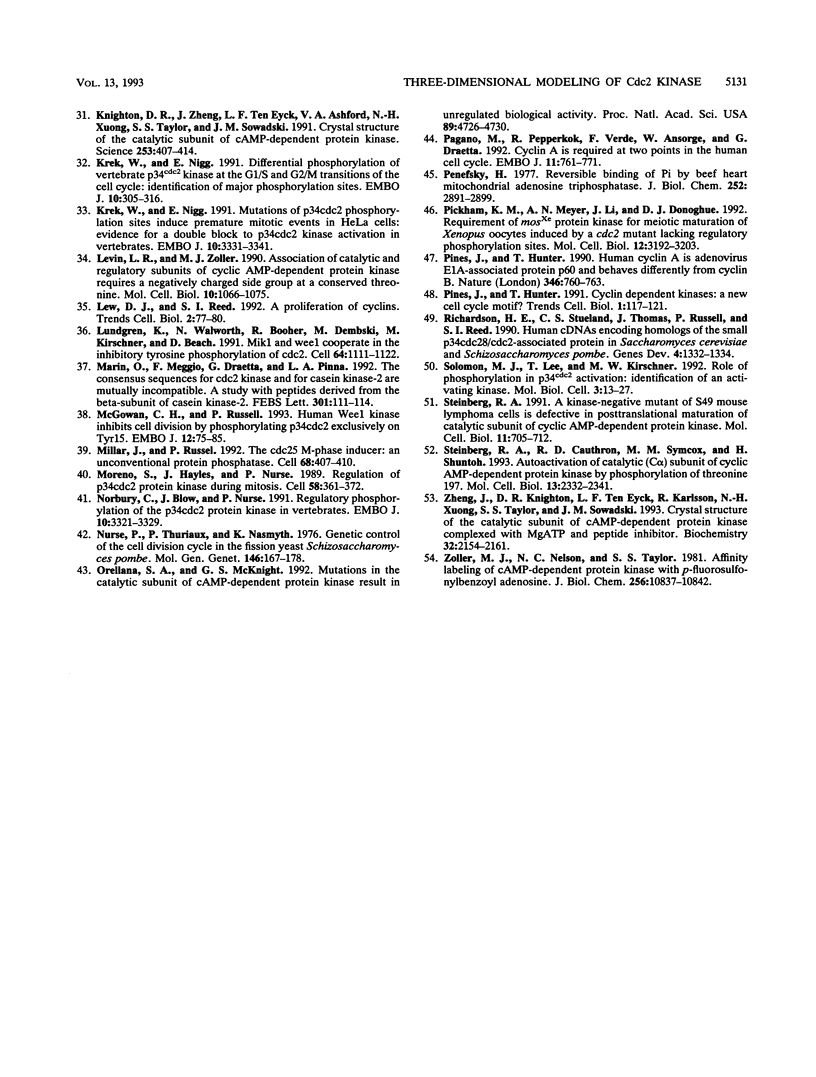
Images in this article
Selected References
These references are in PubMed. This may not be the complete list of references from this article.
- Atherton-Fessler S., Parker L. L., Geahlen R. L., Piwnica-Worms H. Mechanisms of p34cdc2 regulation. Mol Cell Biol. 1993 Mar;13(3):1675–1685. doi: 10.1128/mcb.13.3.1675. [DOI] [PMC free article] [PubMed] [Google Scholar]
- Azzi L., Meijer L., Reed S. I., Pidikiti R., Tung H. Y. Interaction between the cell-cycle-control proteins p34cdc2 and p9CKShs2. Evidence for two cooperative binding domains in p9CKShs2. Eur J Biochem. 1992 Feb 1;203(3):353–360. doi: 10.1111/j.1432-1033.1992.tb16557.x. [DOI] [PubMed] [Google Scholar]
- Barford D., Hu S. H., Johnson L. N. Structural mechanism for glycogen phosphorylase control by phosphorylation and AMP. J Mol Biol. 1991 Mar 5;218(1):233–260. doi: 10.1016/0022-2836(91)90887-c. [DOI] [PubMed] [Google Scholar]
- Booher R. N., Alfa C. E., Hyams J. S., Beach D. H. The fission yeast cdc2/cdc13/suc1 protein kinase: regulation of catalytic activity and nuclear localization. Cell. 1989 Aug 11;58(3):485–497. doi: 10.1016/0092-8674(89)90429-7. [DOI] [PubMed] [Google Scholar]
- Bradford M. M. A rapid and sensitive method for the quantitation of microgram quantities of protein utilizing the principle of protein-dye binding. Anal Biochem. 1976 May 7;72:248–254. doi: 10.1016/0003-2697(76)90527-3. [DOI] [PubMed] [Google Scholar]
- Brizuela L., Draetta G., Beach D. p13suc1 acts in the fission yeast cell division cycle as a component of the p34cdc2 protein kinase. EMBO J. 1987 Nov;6(11):3507–3514. doi: 10.1002/j.1460-2075.1987.tb02676.x. [DOI] [PMC free article] [PubMed] [Google Scholar]
- Brünger A. T., Kuriyan J., Karplus M. Crystallographic R factor refinement by molecular dynamics. Science. 1987 Jan 23;235(4787):458–460. doi: 10.1126/science.235.4787.458. [DOI] [PubMed] [Google Scholar]
- Chothia C., Lesk A. M. The relation between the divergence of sequence and structure in proteins. EMBO J. 1986 Apr;5(4):823–826. doi: 10.1002/j.1460-2075.1986.tb04288.x. [DOI] [PMC free article] [PubMed] [Google Scholar]
- Cunningham B. C., Wells J. A. High-resolution epitope mapping of hGH-receptor interactions by alanine-scanning mutagenesis. Science. 1989 Jun 2;244(4908):1081–1085. doi: 10.1126/science.2471267. [DOI] [PubMed] [Google Scholar]
- Desai D., Gu Y., Morgan D. O. Activation of human cyclin-dependent kinases in vitro. Mol Biol Cell. 1992 May;3(5):571–582. doi: 10.1091/mbc.3.5.571. [DOI] [PMC free article] [PubMed] [Google Scholar]
- Draetta G., Beach D. Activation of cdc2 protein kinase during mitosis in human cells: cell cycle-dependent phosphorylation and subunit rearrangement. Cell. 1988 Jul 1;54(1):17–26. doi: 10.1016/0092-8674(88)90175-4. [DOI] [PubMed] [Google Scholar]
- Draetta G. Cell cycle control in eukaryotes: molecular mechanisms of cdc2 activation. Trends Biochem Sci. 1990 Oct;15(10):378–383. doi: 10.1016/0968-0004(90)90235-4. [DOI] [PubMed] [Google Scholar]
- Ducommun B., Brambilla P., Draetta G. Mutations at sites involved in Suc1 binding inactivate Cdc2. Mol Cell Biol. 1991 Dec;11(12):6177–6184. doi: 10.1128/mcb.11.12.6177. [DOI] [PMC free article] [PubMed] [Google Scholar]
- Ducommun B., Brambilla P., Félix M. A., Franza B. R., Jr, Karsenti E., Draetta G. cdc2 phosphorylation is required for its interaction with cyclin. EMBO J. 1991 Nov;10(11):3311–3319. doi: 10.1002/j.1460-2075.1991.tb04895.x. [DOI] [PMC free article] [PubMed] [Google Scholar]
- Dunphy W. G., Newport J. W. Fission yeast p13 blocks mitotic activation and tyrosine dephosphorylation of the Xenopus cdc2 protein kinase. Cell. 1989 Jul 14;58(1):181–191. doi: 10.1016/0092-8674(89)90414-5. [DOI] [PubMed] [Google Scholar]
- Enoch T., Nurse P. Mutation of fission yeast cell cycle control genes abolishes dependence of mitosis on DNA replication. Cell. 1990 Feb 23;60(4):665–673. doi: 10.1016/0092-8674(90)90669-6. [DOI] [PubMed] [Google Scholar]
- Forsburg S. L., Nurse P. Cell cycle regulation in the yeasts Saccharomyces cerevisiae and Schizosaccharomyces pombe. Annu Rev Cell Biol. 1991;7:227–256. doi: 10.1146/annurev.cb.07.110191.001303. [DOI] [PubMed] [Google Scholar]
- Galaktionov K., Beach D. Specific activation of cdc25 tyrosine phosphatases by B-type cyclins: evidence for multiple roles of mitotic cyclins. Cell. 1991 Dec 20;67(6):1181–1194. doi: 10.1016/0092-8674(91)90294-9. [DOI] [PubMed] [Google Scholar]
- Gautier J., Solomon M. J., Booher R. N., Bazan J. F., Kirschner M. W. cdc25 is a specific tyrosine phosphatase that directly activates p34cdc2. Cell. 1991 Oct 4;67(1):197–211. doi: 10.1016/0092-8674(91)90583-k. [DOI] [PubMed] [Google Scholar]
- Gibbs C. S., Knighton D. R., Sowadski J. M., Taylor S. S., Zoller M. J. Systematic mutational analysis of cAMP-dependent protein kinase identifies unregulated catalytic subunits and defines regions important for the recognition of the regulatory subunit. J Biol Chem. 1992 Mar 5;267(7):4806–4814. [PubMed] [Google Scholar]
- Giordano A., Whyte P., Harlow E., Franza B. R., Jr, Beach D., Draetta G. A 60 kd cdc2-associated polypeptide complexes with the E1A proteins in adenovirus-infected cells. Cell. 1989 Sep 8;58(5):981–990. doi: 10.1016/0092-8674(89)90949-5. [DOI] [PubMed] [Google Scholar]
- Gould K. L., Moreno S., Owen D. J., Sazer S., Nurse P. Phosphorylation at Thr167 is required for Schizosaccharomyces pombe p34cdc2 function. EMBO J. 1991 Nov;10(11):3297–3309. doi: 10.1002/j.1460-2075.1991.tb04894.x. [DOI] [PMC free article] [PubMed] [Google Scholar]
- Gould K. L., Nurse P. Tyrosine phosphorylation of the fission yeast cdc2+ protein kinase regulates entry into mitosis. Nature. 1989 Nov 2;342(6245):39–45. doi: 10.1038/342039a0. [DOI] [PubMed] [Google Scholar]
- Hadwiger J. A., Wittenberg C., Mendenhall M. D., Reed S. I. The Saccharomyces cerevisiae CKS1 gene, a homolog of the Schizosaccharomyces pombe suc1+ gene, encodes a subunit of the Cdc28 protein kinase complex. Mol Cell Biol. 1989 May;9(5):2034–2041. doi: 10.1128/mcb.9.5.2034. [DOI] [PMC free article] [PubMed] [Google Scholar]
- Hayles J., Aves S., Nurse P. suc1 is an essential gene involved in both the cell cycle and growth in fission yeast. EMBO J. 1986 Dec 1;5(12):3373–3379. doi: 10.1002/j.1460-2075.1986.tb04653.x. [DOI] [PMC free article] [PubMed] [Google Scholar]
- Hayles J., Beach D., Durkacz B., Nurse P. The fission yeast cell cycle control gene cdc2: isolation of a sequence suc1 that suppresses cdc2 mutant function. Mol Gen Genet. 1986 Feb;202(2):291–293. doi: 10.1007/BF00331653. [DOI] [PubMed] [Google Scholar]
- Hoffmann I., Clarke P. R., Marcote M. J., Karsenti E., Draetta G. Phosphorylation and activation of human cdc25-C by cdc2--cyclin B and its involvement in the self-amplification of MPF at mitosis. EMBO J. 1993 Jan;12(1):53–63. doi: 10.1002/j.1460-2075.1993.tb05631.x. [DOI] [PMC free article] [PubMed] [Google Scholar]
- Hunt T. Cyclins and their partners: from a simple idea to complicated reality. Semin Cell Biol. 1991 Aug;2(4):213–222. [PubMed] [Google Scholar]
- Knighton D. R., Bell S. M., Zheng J., Ten Eyck L. F., Xuong N. H., Taylor S. S., Sowadski J. M. 2.0 A refined crystal structure of the catalytic subunit of cAMP-dependent protein kinase complexed with a peptide inhibitor and detergent. Acta Crystallogr D Biol Crystallogr. 1993 May 1;49(Pt 3):357–361. doi: 10.1107/S0907444993000502. [DOI] [PubMed] [Google Scholar]
- Knighton D. R., Pearson R. B., Sowadski J. M., Means A. R., Ten Eyck L. F., Taylor S. S., Kemp B. E. Structural basis of the intrasteric regulation of myosin light chain kinases. Science. 1992 Oct 2;258(5079):130–135. doi: 10.1126/science.1439761. [DOI] [PubMed] [Google Scholar]
- Knighton D. R., Zheng J. H., Ten Eyck L. F., Ashford V. A., Xuong N. H., Taylor S. S., Sowadski J. M. Crystal structure of the catalytic subunit of cyclic adenosine monophosphate-dependent protein kinase. Science. 1991 Jul 26;253(5018):407–414. doi: 10.1126/science.1862342. [DOI] [PubMed] [Google Scholar]
- Krek W., Nigg E. A. Differential phosphorylation of vertebrate p34cdc2 kinase at the G1/S and G2/M transitions of the cell cycle: identification of major phosphorylation sites. EMBO J. 1991 Feb;10(2):305–316. doi: 10.1002/j.1460-2075.1991.tb07951.x. [DOI] [PMC free article] [PubMed] [Google Scholar]
- Krek W., Nigg E. A. Mutations of p34cdc2 phosphorylation sites induce premature mitotic events in HeLa cells: evidence for a double block to p34cdc2 kinase activation in vertebrates. EMBO J. 1991 Nov;10(11):3331–3341. doi: 10.1002/j.1460-2075.1991.tb04897.x. [DOI] [PMC free article] [PubMed] [Google Scholar]
- Levin L. R., Zoller M. J. Association of catalytic and regulatory subunits of cyclic AMP-dependent protein kinase requires a negatively charged side group at a conserved threonine. Mol Cell Biol. 1990 Mar;10(3):1066–1075. doi: 10.1128/mcb.10.3.1066. [DOI] [PMC free article] [PubMed] [Google Scholar]
- Lew D. J., I Reed S. A proliferation of cyclins. Trends Cell Biol. 1992 Mar;2(3):77–81. doi: 10.1016/0962-8924(92)90076-y. [DOI] [PubMed] [Google Scholar]
- Lundgren K., Walworth N., Booher R., Dembski M., Kirschner M., Beach D. mik1 and wee1 cooperate in the inhibitory tyrosine phosphorylation of cdc2. Cell. 1991 Mar 22;64(6):1111–1122. doi: 10.1016/0092-8674(91)90266-2. [DOI] [PubMed] [Google Scholar]
- Marin O., Meggio F., Draetta G., Pinna L. A. The consensus sequences for cdc2 kinase and for casein kinase-2 are mutually incompatible. A study with peptides derived from the beta-subunit of casein kinase-2. FEBS Lett. 1992 Apr 13;301(1):111–114. doi: 10.1016/0014-5793(92)80221-2. [DOI] [PubMed] [Google Scholar]
- McGowan C. H., Russell P. Human Wee1 kinase inhibits cell division by phosphorylating p34cdc2 exclusively on Tyr15. EMBO J. 1993 Jan;12(1):75–85. doi: 10.1002/j.1460-2075.1993.tb05633.x. [DOI] [PMC free article] [PubMed] [Google Scholar]
- Millar J. B., Russell P. The cdc25 M-phase inducer: an unconventional protein phosphatase. Cell. 1992 Feb 7;68(3):407–410. doi: 10.1016/0092-8674(92)90177-e. [DOI] [PubMed] [Google Scholar]
- Moreno S., Hayles J., Nurse P. Regulation of p34cdc2 protein kinase during mitosis. Cell. 1989 Jul 28;58(2):361–372. doi: 10.1016/0092-8674(89)90850-7. [DOI] [PubMed] [Google Scholar]
- Norbury C., Blow J., Nurse P. Regulatory phosphorylation of the p34cdc2 protein kinase in vertebrates. EMBO J. 1991 Nov;10(11):3321–3329. doi: 10.1002/j.1460-2075.1991.tb04896.x. [DOI] [PMC free article] [PubMed] [Google Scholar]
- Nurse P., Thuriaux P., Nasmyth K. Genetic control of the cell division cycle in the fission yeast Schizosaccharomyces pombe. Mol Gen Genet. 1976 Jul 23;146(2):167–178. doi: 10.1007/BF00268085. [DOI] [PubMed] [Google Scholar]
- Orellana S. A., McKnight G. S. Mutations in the catalytic subunit of cAMP-dependent protein kinase result in unregulated biological activity. Proc Natl Acad Sci U S A. 1992 May 15;89(10):4726–4730. doi: 10.1073/pnas.89.10.4726. [DOI] [PMC free article] [PubMed] [Google Scholar]
- Penefsky H. S. Reversible binding of Pi by beef heart mitochondrial adenosine triphosphatase. J Biol Chem. 1977 May 10;252(9):2891–2899. [PubMed] [Google Scholar]
- Pickham K. M., Meyer A. N., Li J., Donoghue D. J. Requirement of mosXe protein kinase for meiotic maturation of Xenopus oocytes induced by a cdc2 mutant lacking regulatory phosphorylation sites. Mol Cell Biol. 1992 Jul;12(7):3192–3203. doi: 10.1128/mcb.12.7.3192. [DOI] [PMC free article] [PubMed] [Google Scholar]
- Pines J., Hunter T. Cyclin-dependent kinases: a new cell cycle motif? Trends Cell Biol. 1991 Nov;1(5):117–121. doi: 10.1016/0962-8924(91)90116-q. [DOI] [PubMed] [Google Scholar]
- Pines J., Hunter T. Human cyclin A is adenovirus E1A-associated protein p60 and behaves differently from cyclin B. Nature. 1990 Aug 23;346(6286):760–763. doi: 10.1038/346760a0. [DOI] [PubMed] [Google Scholar]
- Richardson H. E., Stueland C. S., Thomas J., Russell P., Reed S. I. Human cDNAs encoding homologs of the small p34Cdc28/Cdc2-associated protein of Saccharomyces cerevisiae and Schizosaccharomyces pombe. Genes Dev. 1990 Aug;4(8):1332–1344. doi: 10.1101/gad.4.8.1332. [DOI] [PubMed] [Google Scholar]
- Solomon M. J., Lee T., Kirschner M. W. Role of phosphorylation in p34cdc2 activation: identification of an activating kinase. Mol Biol Cell. 1992 Jan;3(1):13–27. doi: 10.1091/mbc.3.1.13. [DOI] [PMC free article] [PubMed] [Google Scholar]
- Steinberg R. A. A kinase-negative mutant of S49 mouse lymphoma cells is defective in posttranslational maturation of catalytic subunit of cyclic AMP-dependent protein kinase. Mol Cell Biol. 1991 Feb;11(2):705–712. doi: 10.1128/mcb.11.2.705. [DOI] [PMC free article] [PubMed] [Google Scholar]
- Steinberg R. A., Cauthron R. D., Symcox M. M., Shuntoh H. Autoactivation of catalytic (C alpha) subunit of cyclic AMP-dependent protein kinase by phosphorylation of threonine 197. Mol Cell Biol. 1993 Apr;13(4):2332–2341. doi: 10.1128/mcb.13.4.2332. [DOI] [PMC free article] [PubMed] [Google Scholar]
- Temperley S. M., Hay R. T. Recognition of the adenovirus type 2 origin of DNA replication by the virally encoded DNA polymerase and preterminal proteins. EMBO J. 1992 Feb;11(2):761–768. doi: 10.1002/j.1460-2075.1992.tb05109.x. [DOI] [PMC free article] [PubMed] [Google Scholar]
- Zheng J., Knighton D. R., ten Eyck L. F., Karlsson R., Xuong N., Taylor S. S., Sowadski J. M. Crystal structure of the catalytic subunit of cAMP-dependent protein kinase complexed with MgATP and peptide inhibitor. Biochemistry. 1993 Mar 9;32(9):2154–2161. doi: 10.1021/bi00060a005. [DOI] [PubMed] [Google Scholar]
- Zoller M. J., Nelson N. C., Taylor S. S. Affinity labeling of cAMP-dependent protein kinase with p-fluorosulfonylbenzoyl adenosine. Covalent modification of lysine 71. J Biol Chem. 1981 Nov 10;256(21):10837–10842. [PubMed] [Google Scholar]