Abstract
The full-length retroviral transcript serves as genomic RNA for progeny virions, as an mRNA for structural proteins and enzymes, and as a pre-mRNA substrate for splicing that yields subgenomic mRNAs that encode other essential proteins. Thus, RNA splicing to form subgenomic mRNAs must be incomplete or regulated in order to preserve some of the full-length transcripts. We have used the avian sarcoma virus system to delineate the viral functions that are required in the regulation of the splicing event that forms the envelope glycoprotein (env) subgenomic mRNA. We observed previously that a specific insertion mutation just 5' of the env splice acceptor site resulted in nearly complete splicing to form env mRNA and a concomitant replication defect which is presumably due to a deficit of the full-length transcript. Replication-competent pseudorevertants contained second-site mutations that restored splicing control, and these mapped either just upstream or downstream of the env splice acceptor site. In this report, we show that splicing control at this site does not require expression of any known viral replication protein(s), nor does it appear to require the viral splice donor site. From these results and analysis of additional splicing mutations obtained by in vivo selection, we conclude that splicing is controlled through the maintenance of suboptimal cis-acting signals in the viral RNA that alter the efficiency of recognition by the cellular splicing machinery.
Full text
PDF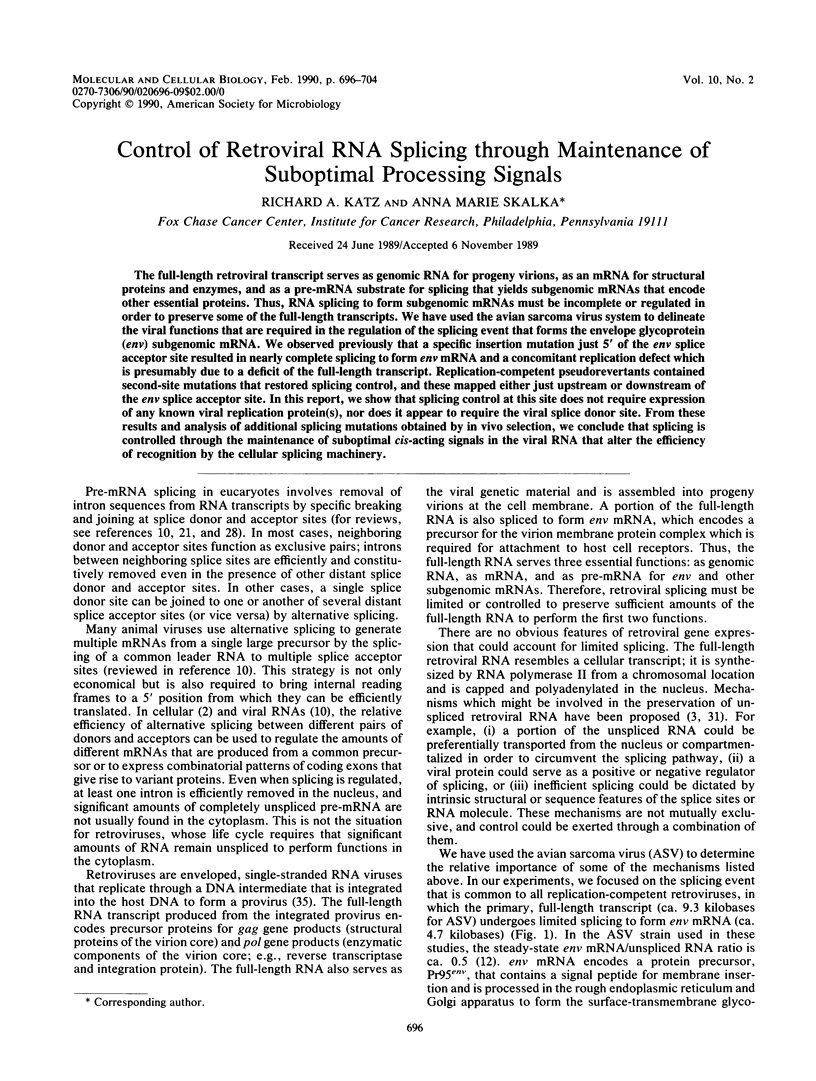
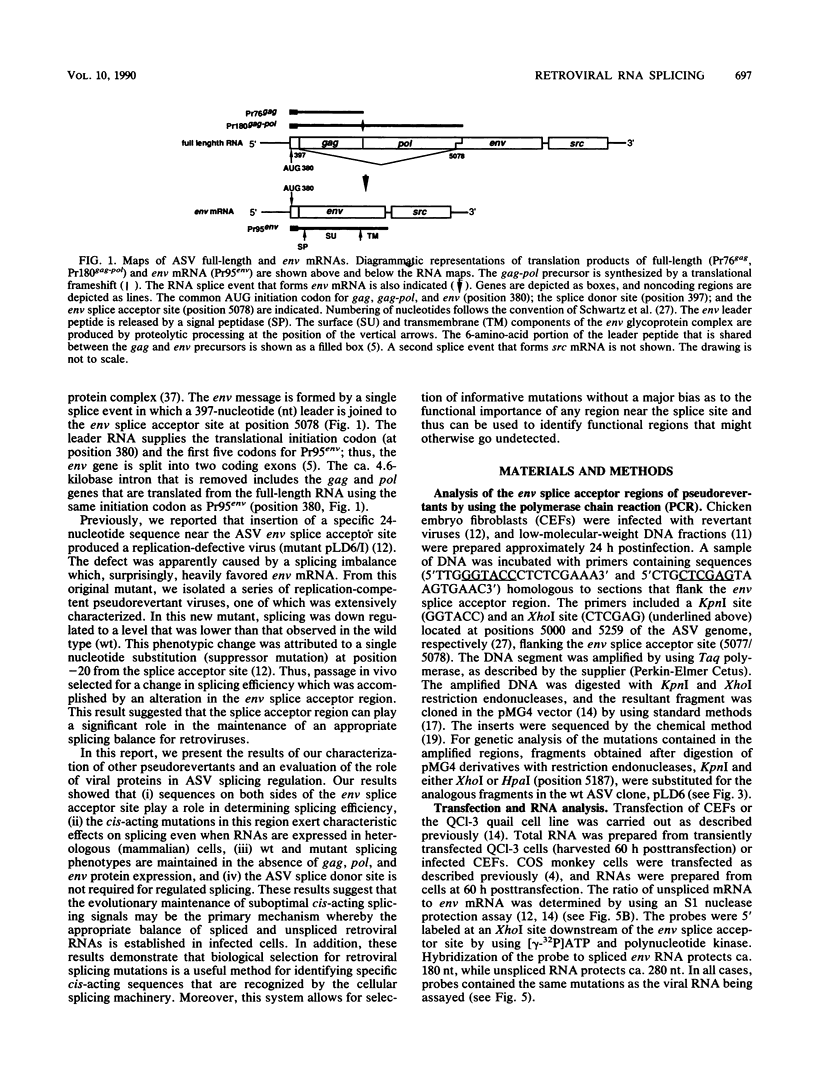
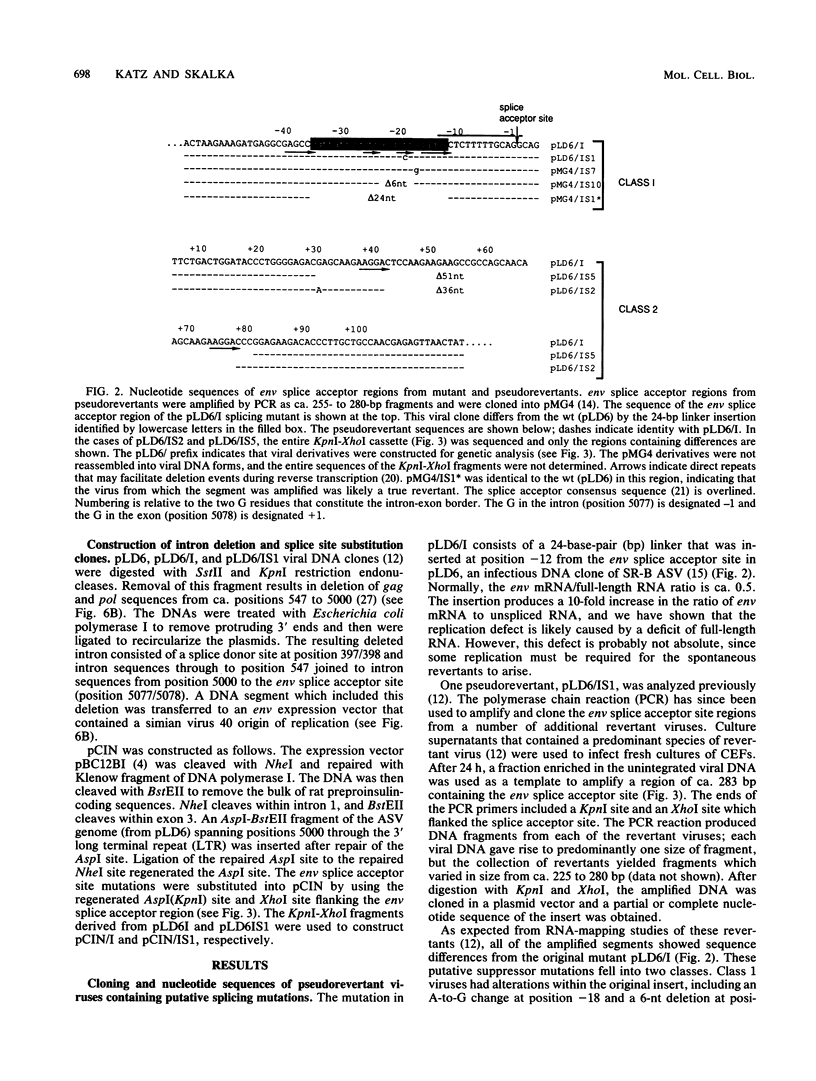
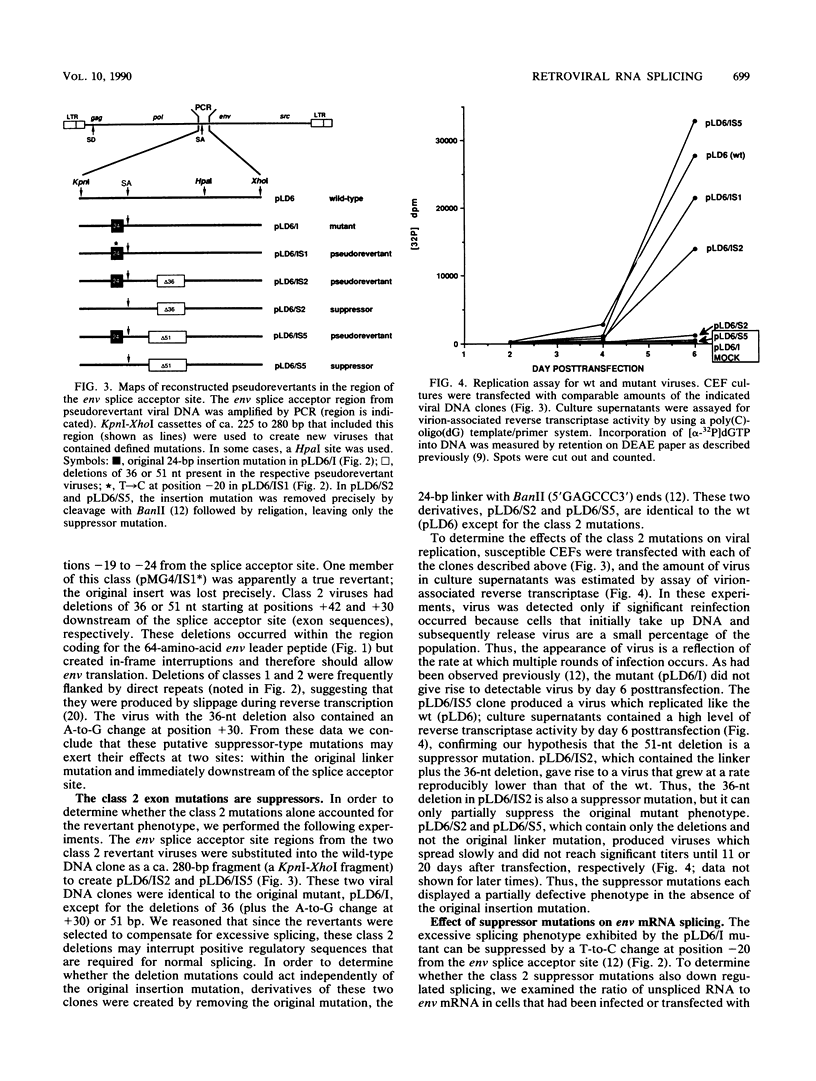
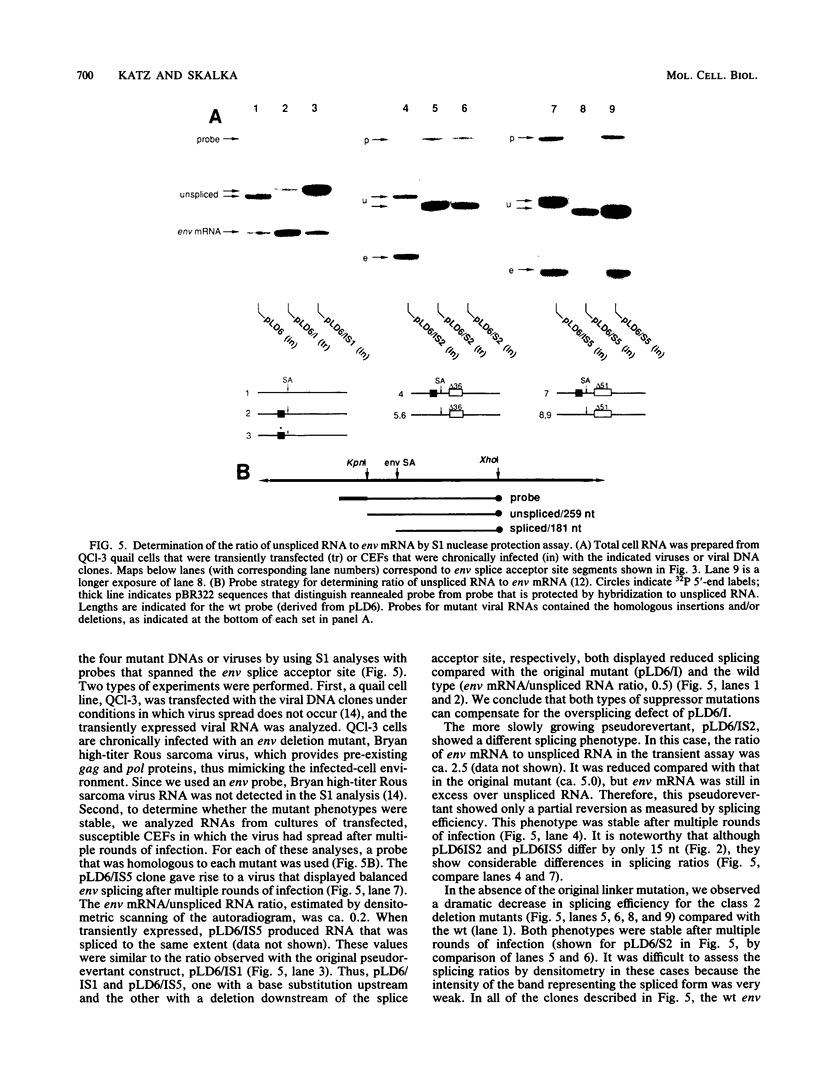
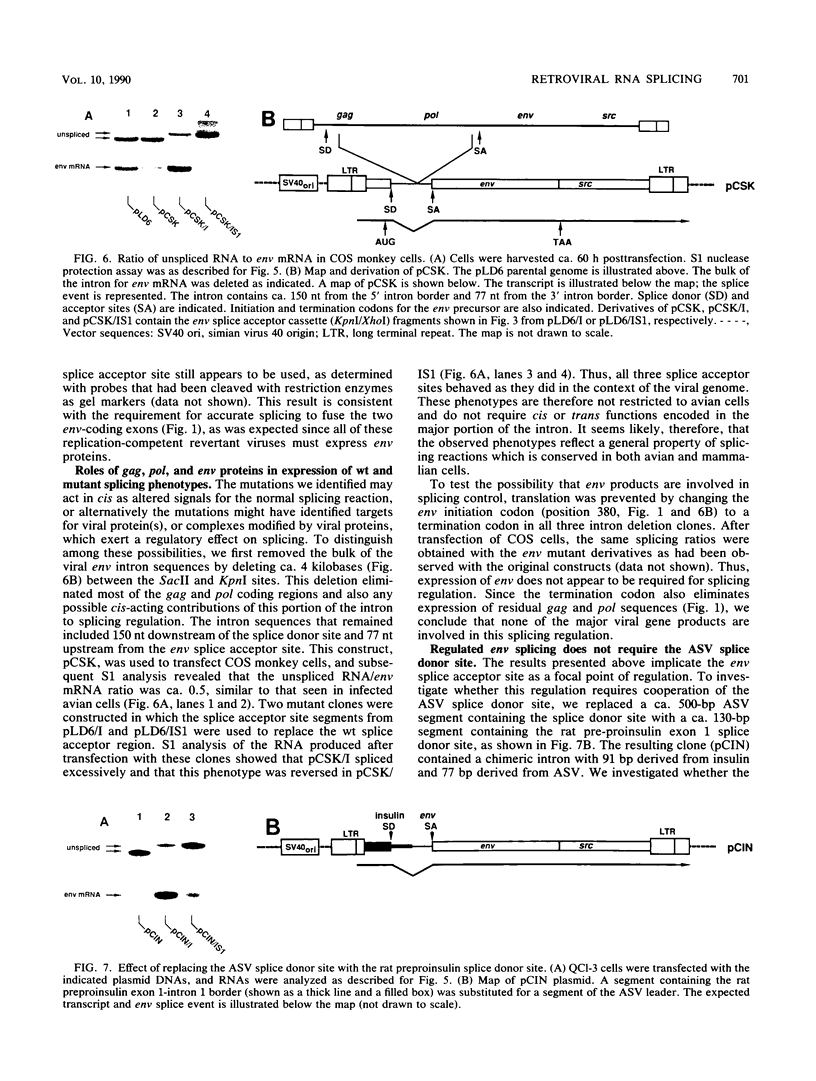
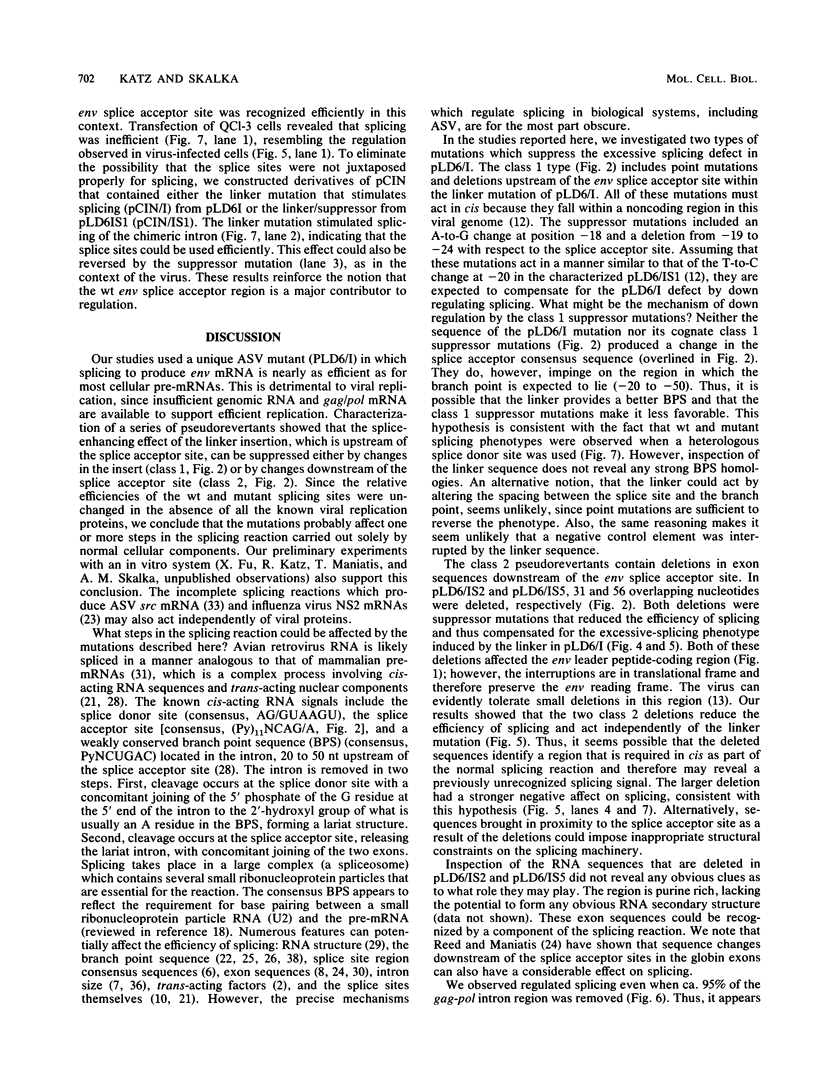
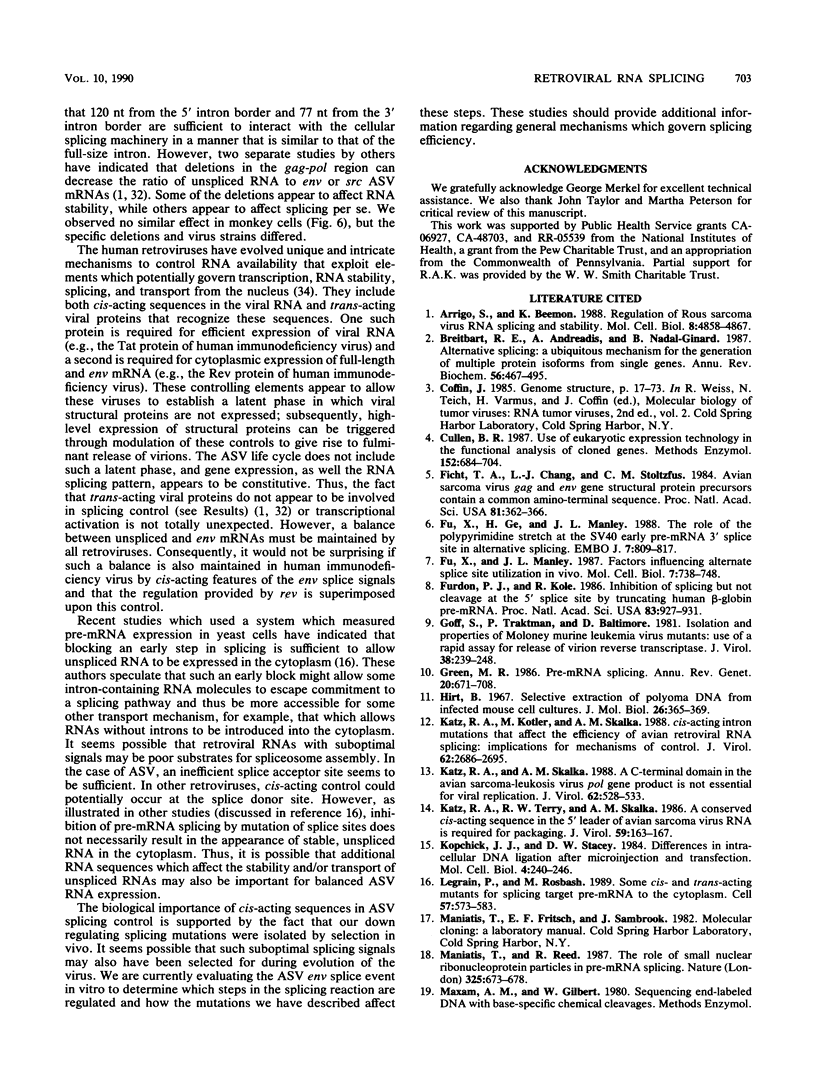
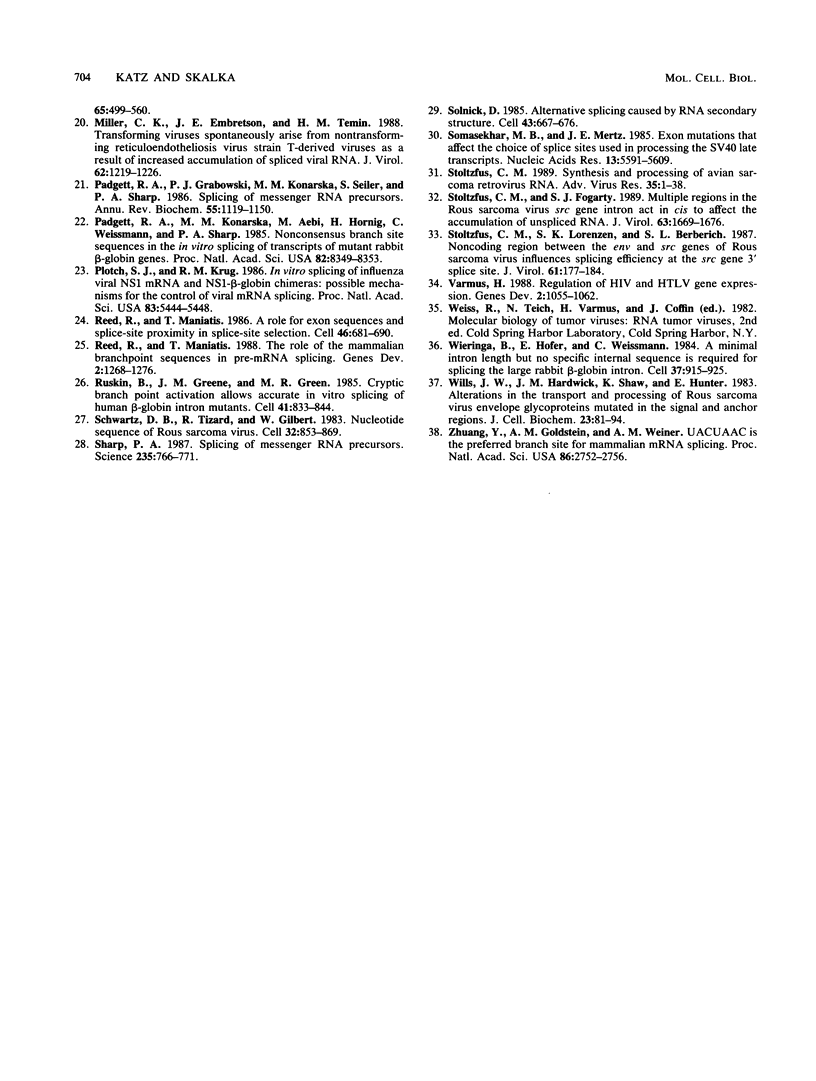
Images in this article
Selected References
These references are in PubMed. This may not be the complete list of references from this article.
- Arrigo S., Beemon K. Regulation of Rous sarcoma virus RNA splicing and stability. Mol Cell Biol. 1988 Nov;8(11):4858–4867. doi: 10.1128/mcb.8.11.4858. [DOI] [PMC free article] [PubMed] [Google Scholar]
- Breitbart R. E., Andreadis A., Nadal-Ginard B. Alternative splicing: a ubiquitous mechanism for the generation of multiple protein isoforms from single genes. Annu Rev Biochem. 1987;56:467–495. doi: 10.1146/annurev.bi.56.070187.002343. [DOI] [PubMed] [Google Scholar]
- Cullen B. R. Use of eukaryotic expression technology in the functional analysis of cloned genes. Methods Enzymol. 1987;152:684–704. doi: 10.1016/0076-6879(87)52074-2. [DOI] [PubMed] [Google Scholar]
- Ficht T. A., Chang L. J., Stoltzfus C. M. Avian sarcoma virus gag and env gene structural protein precursors contain a common amino-terminal sequence. Proc Natl Acad Sci U S A. 1984 Jan;81(2):362–366. doi: 10.1073/pnas.81.2.362. [DOI] [PMC free article] [PubMed] [Google Scholar]
- Fu X. Y., Ge H., Manley J. L. The role of the polypyrimidine stretch at the SV40 early pre-mRNA 3' splice site in alternative splicing. EMBO J. 1988 Mar;7(3):809–817. doi: 10.1002/j.1460-2075.1988.tb02879.x. [DOI] [PMC free article] [PubMed] [Google Scholar]
- Fu X. Y., Manley J. L. Factors influencing alternative splice site utilization in vivo. Mol Cell Biol. 1987 Feb;7(2):738–748. doi: 10.1128/mcb.7.2.738. [DOI] [PMC free article] [PubMed] [Google Scholar]
- Furdon P. J., Kole R. Inhibition of splicing but not cleavage at the 5' splice site by truncating human beta-globin pre-mRNA. Proc Natl Acad Sci U S A. 1986 Feb;83(4):927–931. doi: 10.1073/pnas.83.4.927. [DOI] [PMC free article] [PubMed] [Google Scholar]
- Goff S., Traktman P., Baltimore D. Isolation and properties of Moloney murine leukemia virus mutants: use of a rapid assay for release of virion reverse transcriptase. J Virol. 1981 Apr;38(1):239–248. doi: 10.1128/jvi.38.1.239-248.1981. [DOI] [PMC free article] [PubMed] [Google Scholar]
- Green M. R. Pre-mRNA splicing. Annu Rev Genet. 1986;20:671–708. doi: 10.1146/annurev.ge.20.120186.003323. [DOI] [PubMed] [Google Scholar]
- Hirt B. Selective extraction of polyoma DNA from infected mouse cell cultures. J Mol Biol. 1967 Jun 14;26(2):365–369. doi: 10.1016/0022-2836(67)90307-5. [DOI] [PubMed] [Google Scholar]
- Katz R. A., Kotler M., Skalka A. M. cis-acting intron mutations that affect the efficiency of avian retroviral RNA splicing: implication for mechanisms of control. J Virol. 1988 Aug;62(8):2686–2695. doi: 10.1128/jvi.62.8.2686-2695.1988. [DOI] [PMC free article] [PubMed] [Google Scholar]
- Katz R. A., Skalka A. M. A C-terminal domain in the avian sarcoma-leukosis virus pol gene product is not essential for viral replication. J Virol. 1988 Feb;62(2):528–533. doi: 10.1128/jvi.62.2.528-533.1988. [DOI] [PMC free article] [PubMed] [Google Scholar]
- Katz R. A., Terry R. W., Skalka A. M. A conserved cis-acting sequence in the 5' leader of avian sarcoma virus RNA is required for packaging. J Virol. 1986 Jul;59(1):163–167. doi: 10.1128/jvi.59.1.163-167.1986. [DOI] [PMC free article] [PubMed] [Google Scholar]
- Kopchick J. J., Stacey D. W. Differences in intracellular DNA ligation after microinjection and transfection. Mol Cell Biol. 1984 Feb;4(2):240–246. doi: 10.1128/mcb.4.2.240. [DOI] [PMC free article] [PubMed] [Google Scholar]
- Legrain P., Rosbash M. Some cis- and trans-acting mutants for splicing target pre-mRNA to the cytoplasm. Cell. 1989 May 19;57(4):573–583. doi: 10.1016/0092-8674(89)90127-x. [DOI] [PubMed] [Google Scholar]
- Maniatis T., Reed R. The role of small nuclear ribonucleoprotein particles in pre-mRNA splicing. Nature. 1987 Feb 19;325(6106):673–678. doi: 10.1038/325673a0. [DOI] [PubMed] [Google Scholar]
- Miller C. K., Embretson J. E., Temin H. M. Transforming viruses spontaneously arise from nontransforming reticuloendotheliosis virus strain T-derived viruses as a result of increased accumulation of spliced viral RNA. J Virol. 1988 Apr;62(4):1219–1226. doi: 10.1128/jvi.62.4.1219-1226.1988. [DOI] [PMC free article] [PubMed] [Google Scholar]
- Padgett R. A., Grabowski P. J., Konarska M. M., Seiler S., Sharp P. A. Splicing of messenger RNA precursors. Annu Rev Biochem. 1986;55:1119–1150. doi: 10.1146/annurev.bi.55.070186.005351. [DOI] [PubMed] [Google Scholar]
- Padgett R. A., Konarska M. M., Aebi M., Hornig H., Weissmann C., Sharp P. A. Nonconsensus branch-site sequences in the in vitro splicing of transcripts of mutant rabbit beta-globin genes. Proc Natl Acad Sci U S A. 1985 Dec;82(24):8349–8353. doi: 10.1073/pnas.82.24.8349. [DOI] [PMC free article] [PubMed] [Google Scholar]
- Plotch S. J., Krug R. M. In vitro splicing of influenza viral NS1 mRNA and NS1-beta-globin chimeras: possible mechanisms for the control of viral mRNA splicing. Proc Natl Acad Sci U S A. 1986 Aug;83(15):5444–5448. doi: 10.1073/pnas.83.15.5444. [DOI] [PMC free article] [PubMed] [Google Scholar]
- Reed R., Maniatis T. A role for exon sequences and splice-site proximity in splice-site selection. Cell. 1986 Aug 29;46(5):681–690. doi: 10.1016/0092-8674(86)90343-0. [DOI] [PubMed] [Google Scholar]
- Reed R., Maniatis T. The role of the mammalian branchpoint sequence in pre-mRNA splicing. Genes Dev. 1988 Oct;2(10):1268–1276. doi: 10.1101/gad.2.10.1268. [DOI] [PubMed] [Google Scholar]
- Ruskin B., Greene J. M., Green M. R. Cryptic branch point activation allows accurate in vitro splicing of human beta-globin intron mutants. Cell. 1985 Jul;41(3):833–844. doi: 10.1016/s0092-8674(85)80064-7. [DOI] [PubMed] [Google Scholar]
- Schwartz D. E., Tizard R., Gilbert W. Nucleotide sequence of Rous sarcoma virus. Cell. 1983 Mar;32(3):853–869. doi: 10.1016/0092-8674(83)90071-5. [DOI] [PubMed] [Google Scholar]
- Sharp P. A. Splicing of messenger RNA precursors. Science. 1987 Feb 13;235(4790):766–771. doi: 10.1126/science.3544217. [DOI] [PubMed] [Google Scholar]
- Solnick D. Alternative splicing caused by RNA secondary structure. Cell. 1985 Dec;43(3 Pt 2):667–676. doi: 10.1016/0092-8674(85)90239-9. [DOI] [PubMed] [Google Scholar]
- Somasekhar M. B., Mertz J. E. Exon mutations that affect the choice of splice sites used in processing the SV40 late transcripts. Nucleic Acids Res. 1985 Aug 12;13(15):5591–5609. doi: 10.1093/nar/13.15.5591. [DOI] [PMC free article] [PubMed] [Google Scholar]
- Stoltzfus C. M., Fogarty S. J. Multiple regions in the Rous sarcoma virus src gene intron act in cis to affect the accumulation of unspliced RNA. J Virol. 1989 Apr;63(4):1669–1676. doi: 10.1128/jvi.63.4.1669-1676.1989. [DOI] [PMC free article] [PubMed] [Google Scholar]
- Stoltzfus C. M., Lorenzen S. K., Berberich S. L. Noncoding region between the env and src genes of Rous sarcoma virus influences splicing efficiency at the src gene 3' splice site. J Virol. 1987 Jan;61(1):177–184. doi: 10.1128/jvi.61.1.177-184.1987. [DOI] [PMC free article] [PubMed] [Google Scholar]
- Stoltzfus C. M. Synthesis and processing of avian sarcoma retrovirus RNA. Adv Virus Res. 1988;35:1–38. doi: 10.1016/s0065-3527(08)60707-1. [DOI] [PubMed] [Google Scholar]
- Varmus H. Regulation of HIV and HTLV gene expression. Genes Dev. 1988 Sep;2(9):1055–1062. doi: 10.1101/gad.2.9.1055. [DOI] [PubMed] [Google Scholar]
- Wieringa B., Hofer E., Weissmann C. A minimal intron length but no specific internal sequence is required for splicing the large rabbit beta-globin intron. Cell. 1984 Jul;37(3):915–925. doi: 10.1016/0092-8674(84)90426-4. [DOI] [PubMed] [Google Scholar]
- Wills J. W., Hardwick J. M., Shaw K., Hunter E. Alterations in the transport and processing of Rous sarcoma virus envelope glycoproteins mutated in the signal and anchor regions. J Cell Biochem. 1983;23(1-4):81–94. doi: 10.1002/jcb.240230109. [DOI] [PubMed] [Google Scholar]