Abstract
In mouse cells, the major inducible heat shock protein is a protein of 68,000 daltons (hsp68). We have previously shown that mouse plasmacytomas do not express hsp68. We have now made use of these natural mutants to assess the contribution of hsp68 to acquired thermotolerance. An endpoint limiting dilution assay was used to quantify cell survival to lethal stresses. Two test plasmacytoma cell lines (C1.18.1 and J558) and an hsp68-positive myeloma, XC1.1/51, used as a control, were examined. All showed recovery when pretreated for 10 min at 44 degrees C 2 h before exposure to otherwise lethal stresses of 1 to 4 h at 43 degrees C. Similar results were obtained with the Friend erythroleukemia line D1B, which we have also shown not to express hsp68. These results indicate that hsp68 is not required for protection against thermal stresses in mouse cells.
Full text
PDF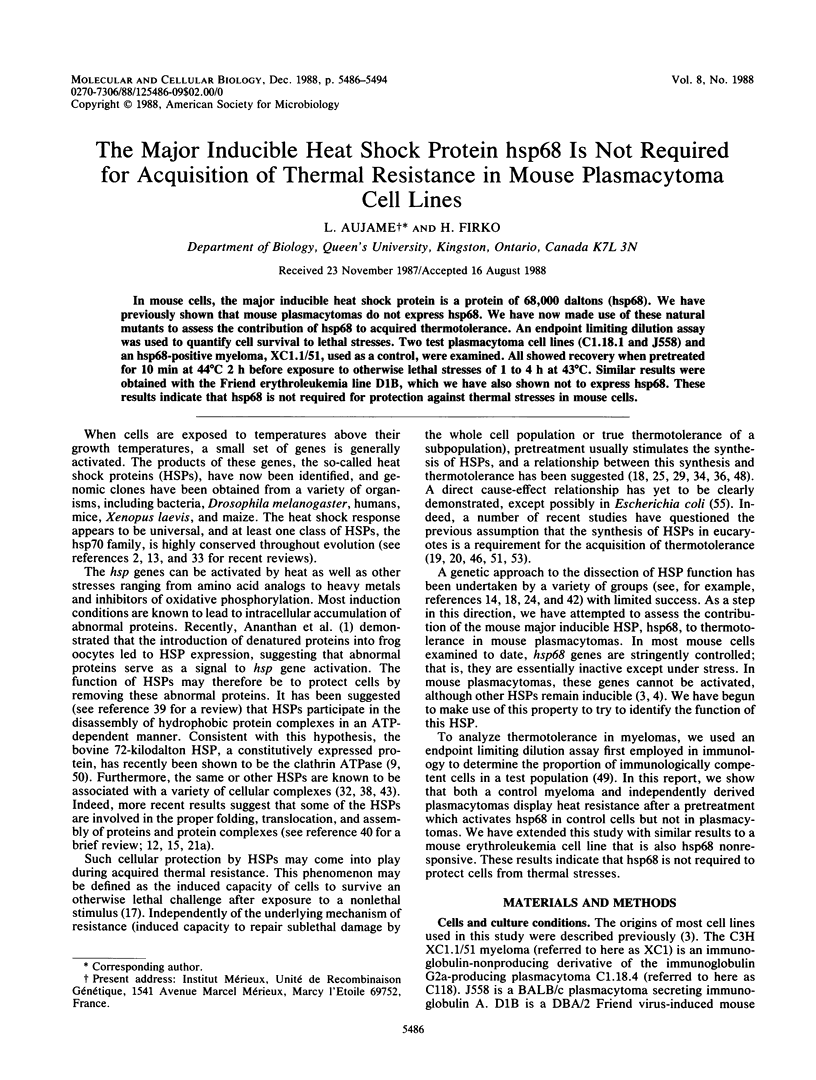
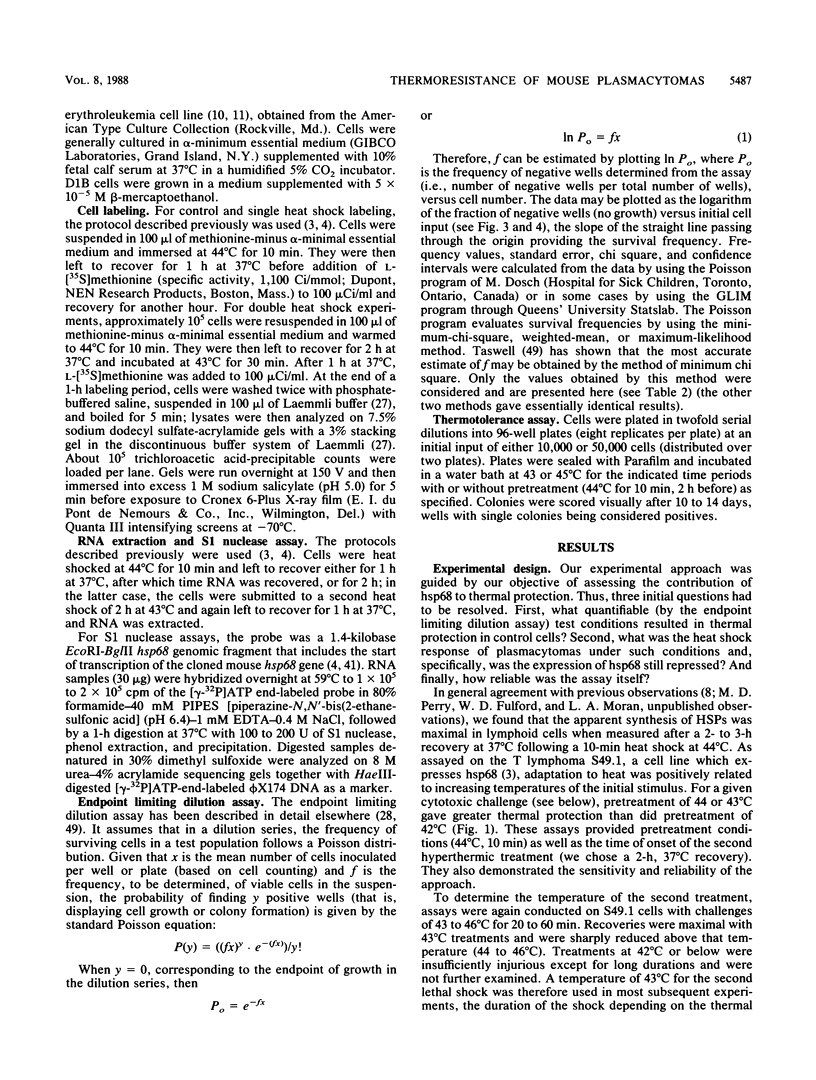
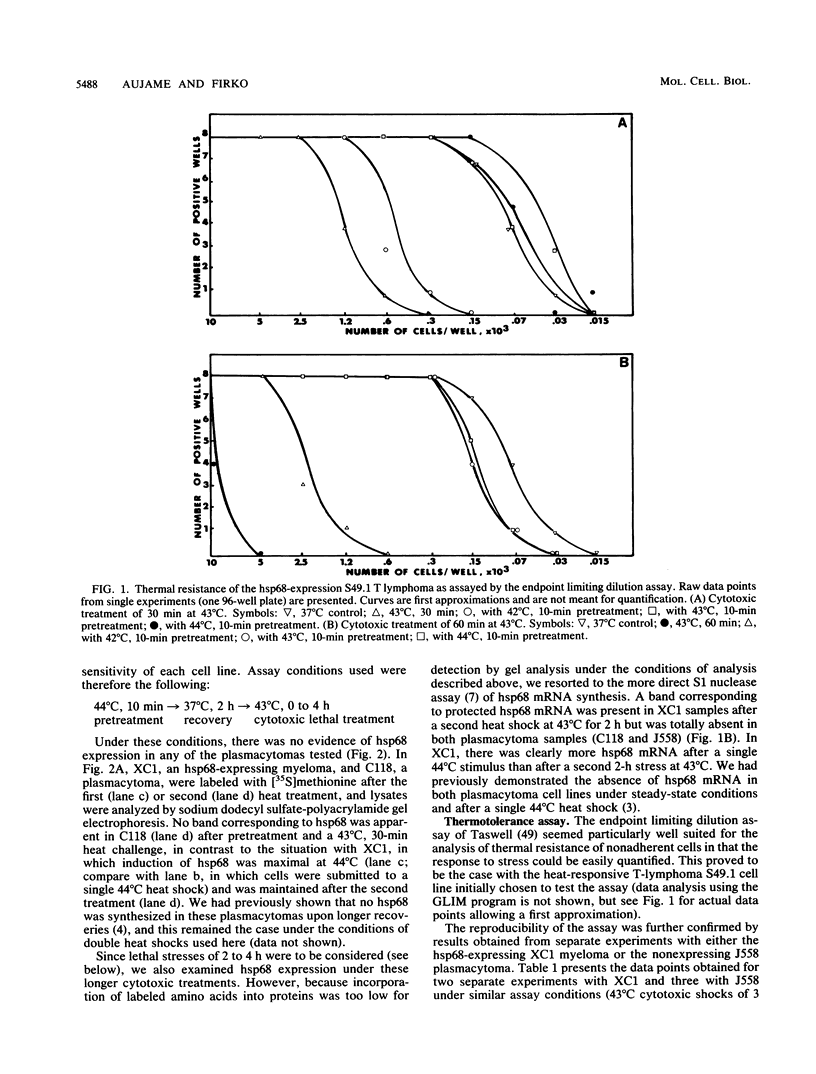
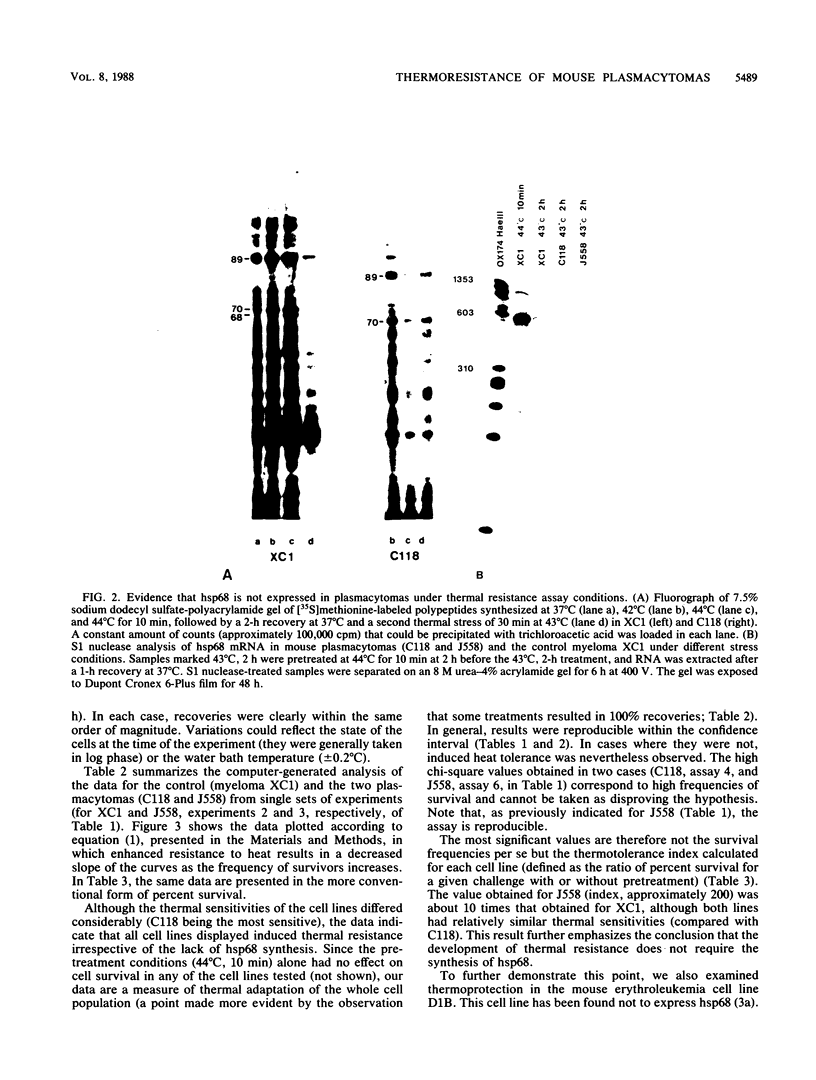
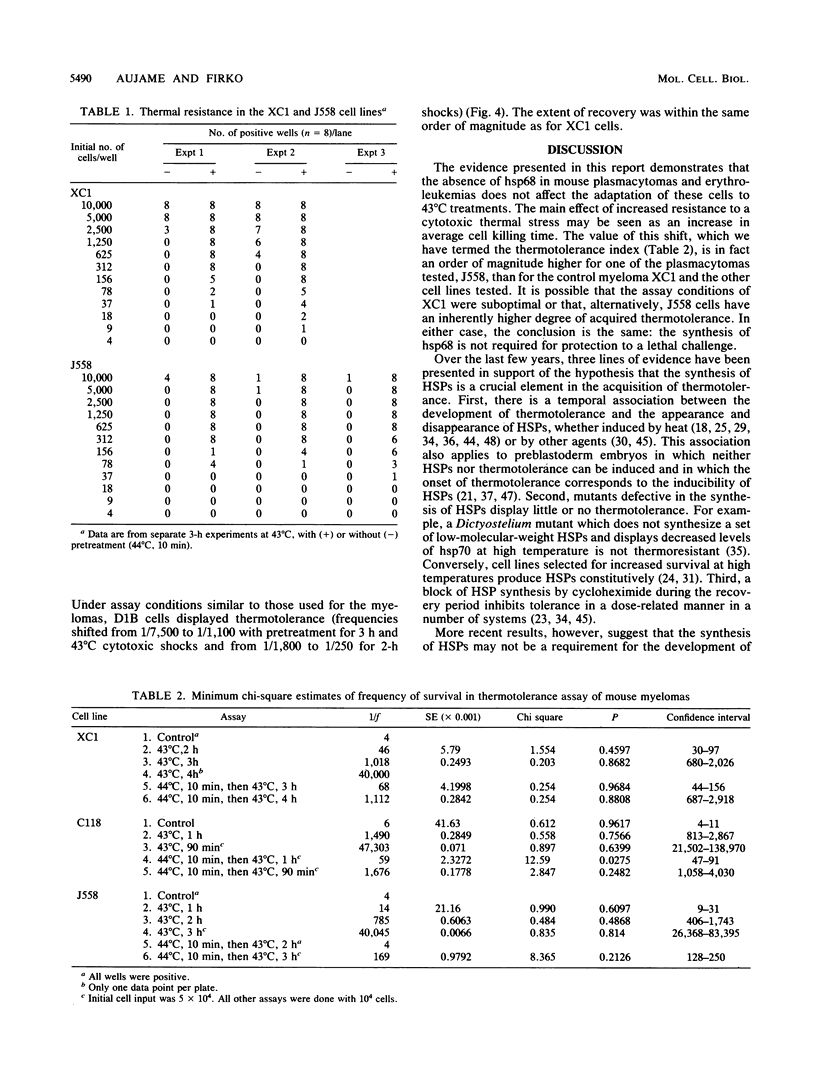
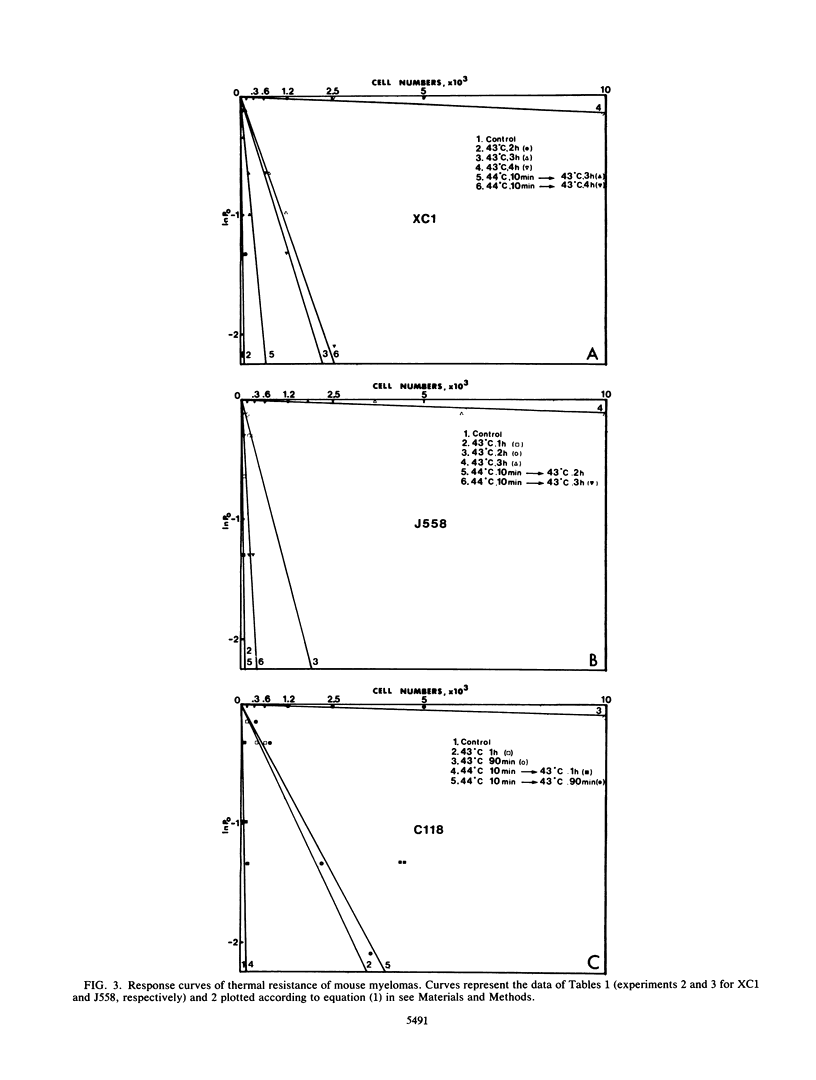
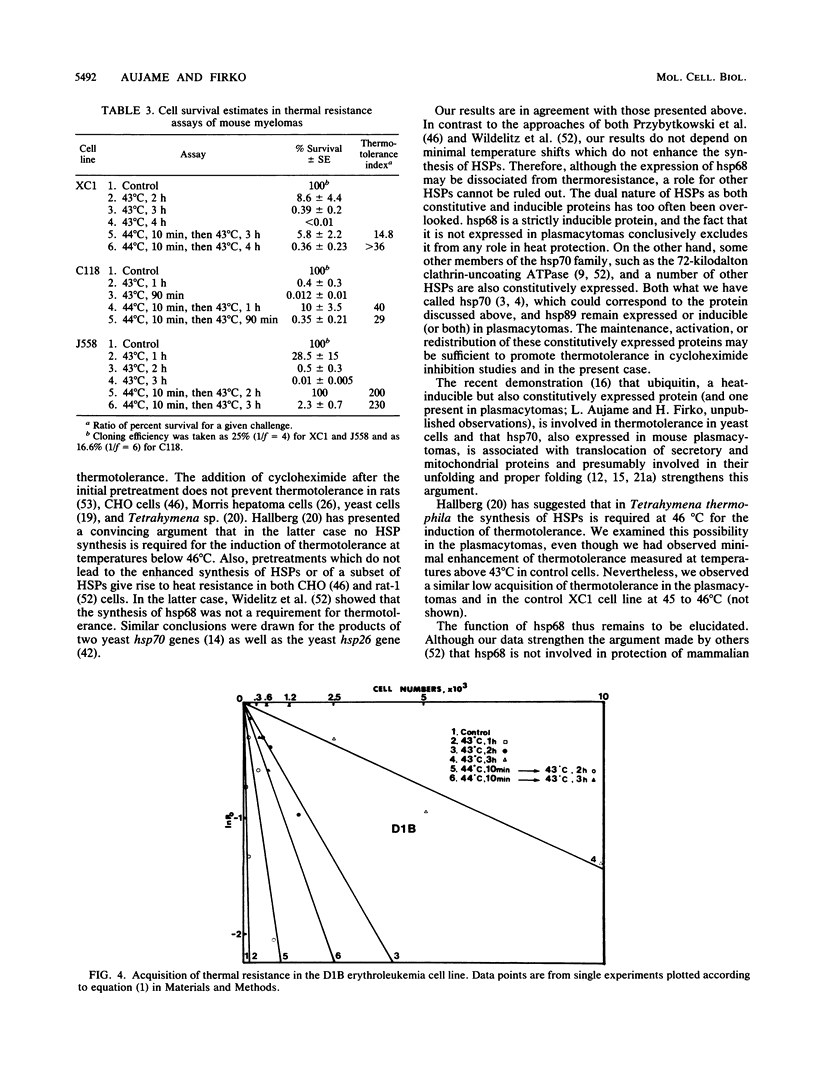
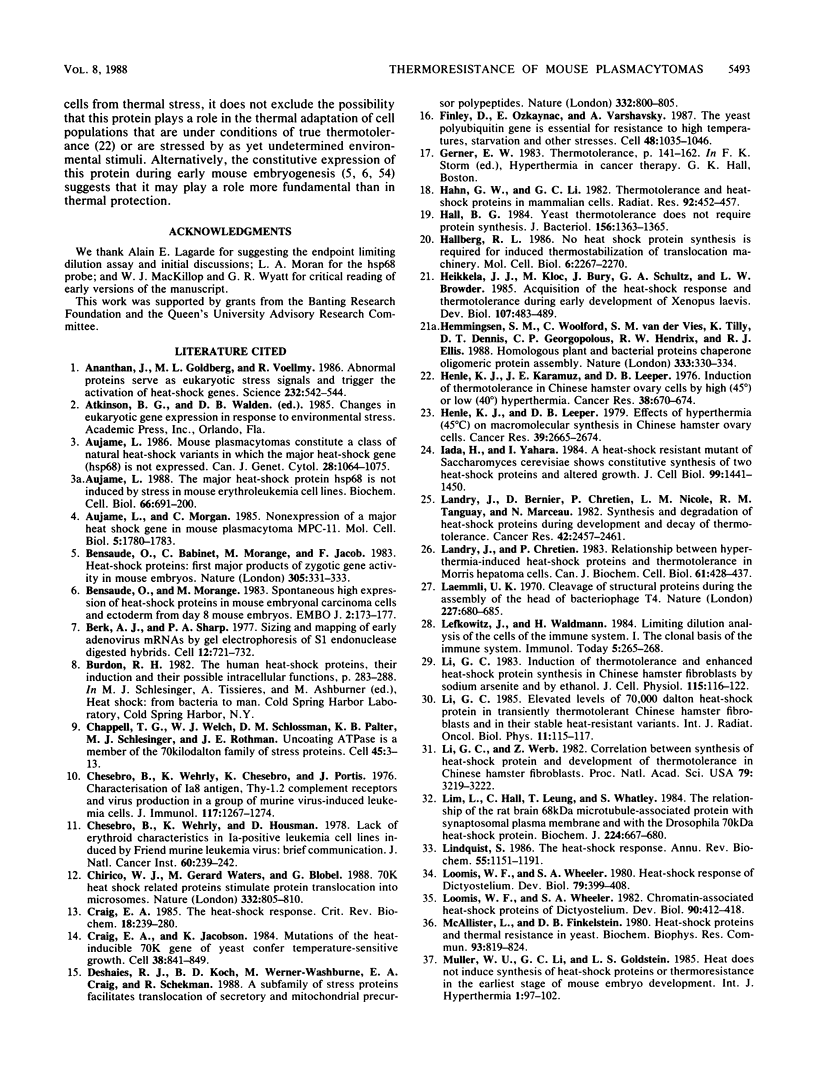
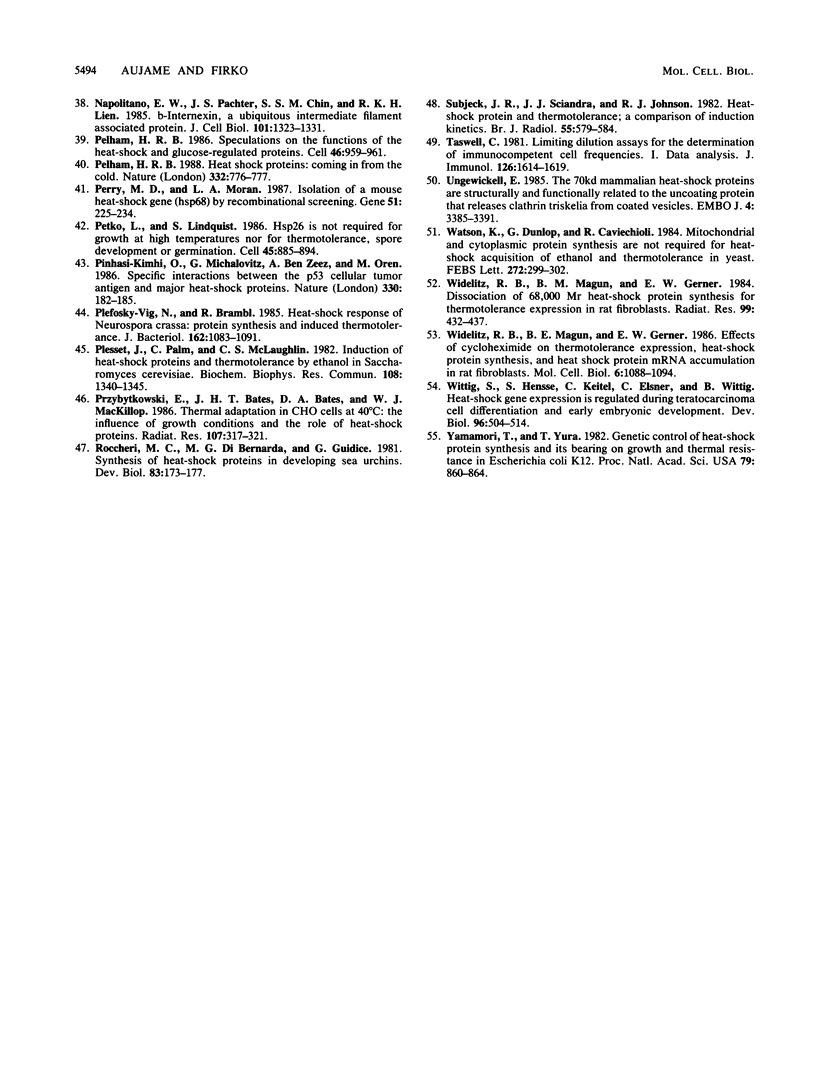
Images in this article
Selected References
These references are in PubMed. This may not be the complete list of references from this article.
- Aujame L., Morgan C. Nonexpression of a major heat shock gene in mouse plasmacytoma MPC-11. Mol Cell Biol. 1985 Jul;5(7):1780–1783. doi: 10.1128/mcb.5.7.1780. [DOI] [PMC free article] [PubMed] [Google Scholar]
- Aujame L. Murine plasmacytomas constitute a class of natural heat-shock variants in which the major inducible hsp-68 gene is not expressed. Can J Genet Cytol. 1986 Dec;28(6):1064–1075. doi: 10.1139/g86-150. [DOI] [PubMed] [Google Scholar]
- Aujame L. The major heat-shock protein hsp 68 is not induced by stress in mouse erythroleukemia cell lines. Biochem Cell Biol. 1988 Jul;66(7):691–701. doi: 10.1139/o88-079. [DOI] [PubMed] [Google Scholar]
- Bensaude O., Babinet C., Morange M., Jacob F. Heat shock proteins, first major products of zygotic gene activity in mouse embryo. Nature. 1983 Sep 22;305(5932):331–333. doi: 10.1038/305331a0. [DOI] [PubMed] [Google Scholar]
- Bensaude O., Morange M. Spontaneous high expression of heat-shock proteins in mouse embryonal carcinoma cells and ectoderm from day 8 mouse embryo. EMBO J. 1983;2(2):173–177. doi: 10.1002/j.1460-2075.1983.tb01401.x. [DOI] [PMC free article] [PubMed] [Google Scholar]
- Berk A. J., Sharp P. A. Sizing and mapping of early adenovirus mRNAs by gel electrophoresis of S1 endonuclease-digested hybrids. Cell. 1977 Nov;12(3):721–732. doi: 10.1016/0092-8674(77)90272-0. [DOI] [PubMed] [Google Scholar]
- Chesebro B., Wehrly K., Chesebro K., Portis J. Characterization of Ia8 antigen, thy-1.2 antigen, complement receptors, and virus production in a group of murine virus-induced leukemia cell lines. J Immunol. 1976 Oct;117(4):1267–1274. [PubMed] [Google Scholar]
- Chesebro B., Wehrly K., Housman D. Lack of erythroid characteristics in Ia-positive leukemia cell lines induced by Friend murine leukemia virus: brief communication. J Natl Cancer Inst. 1978 Jan;60(1):239–242. doi: 10.1093/jnci/60.1.239. [DOI] [PubMed] [Google Scholar]
- Chirico W. J., Waters M. G., Blobel G. 70K heat shock related proteins stimulate protein translocation into microsomes. Nature. 1988 Apr 28;332(6167):805–810. doi: 10.1038/332805a0. [DOI] [PubMed] [Google Scholar]
- Craig E. A., Jacobsen K. Mutations of the heat inducible 70 kilodalton genes of yeast confer temperature sensitive growth. Cell. 1984 Oct;38(3):841–849. doi: 10.1016/0092-8674(84)90279-4. [DOI] [PubMed] [Google Scholar]
- Craig E. A. The heat shock response. CRC Crit Rev Biochem. 1985;18(3):239–280. doi: 10.3109/10409238509085135. [DOI] [PubMed] [Google Scholar]
- Deshaies R. J., Koch B. D., Werner-Washburne M., Craig E. A., Schekman R. A subfamily of stress proteins facilitates translocation of secretory and mitochondrial precursor polypeptides. Nature. 1988 Apr 28;332(6167):800–805. doi: 10.1038/332800a0. [DOI] [PubMed] [Google Scholar]
- Finley D., Ozkaynak E., Varshavsky A. The yeast polyubiquitin gene is essential for resistance to high temperatures, starvation, and other stresses. Cell. 1987 Mar 27;48(6):1035–1046. doi: 10.1016/0092-8674(87)90711-2. [DOI] [PubMed] [Google Scholar]
- Hahn G. M., Li G. C. Thermotolerance and heat shock proteins in mammalian cells. Radiat Res. 1982 Dec;92(3):452–457. [PubMed] [Google Scholar]
- Hall B. G. Yeast thermotolerance does not require protein synthesis. J Bacteriol. 1983 Dec;156(3):1363–1365. doi: 10.1128/jb.156.3.1363-1365.1983. [DOI] [PMC free article] [PubMed] [Google Scholar]
- Hallberg R. L. No heat shock protein synthesis is required for induced thermostabilization of translational machinery. Mol Cell Biol. 1986 Jun;6(6):2267–2270. doi: 10.1128/mcb.6.6.2267. [DOI] [PMC free article] [PubMed] [Google Scholar]
- Heikkila J. J., Kloc M., Bury J., Schultz G. A., Browder L. W. Acquisition of the heat-shock response and thermotolerance during early development of Xenopus laevis. Dev Biol. 1985 Feb;107(2):483–489. doi: 10.1016/0012-1606(85)90329-x. [DOI] [PubMed] [Google Scholar]
- Hemmingsen S. M., Woolford C., van der Vies S. M., Tilly K., Dennis D. T., Georgopoulos C. P., Hendrix R. W., Ellis R. J. Homologous plant and bacterial proteins chaperone oligomeric protein assembly. Nature. 1988 May 26;333(6171):330–334. doi: 10.1038/333330a0. [DOI] [PubMed] [Google Scholar]
- Henle K. J., Leeper D. B. Effects of hyperthermia (45 degrees) on macromolecular synthesis in Chinese hamster ovary cells. Cancer Res. 1979 Jul;39(7 Pt 1):2665–2674. [PubMed] [Google Scholar]
- Iida H., Yahara I. A heat shock-resistant mutant of Saccharomyces cerevisiae shows constitutive synthesis of two heat shock proteins and altered growth. J Cell Biol. 1984 Oct;99(4 Pt 1):1441–1450. doi: 10.1083/jcb.99.4.1441. [DOI] [PMC free article] [PubMed] [Google Scholar]
- Laemmli U. K. Cleavage of structural proteins during the assembly of the head of bacteriophage T4. Nature. 1970 Aug 15;227(5259):680–685. doi: 10.1038/227680a0. [DOI] [PubMed] [Google Scholar]
- Landry J., Bernier D., Chrétien P., Nicole L. M., Tanguay R. M., Marceau N. Synthesis and degradation of heat shock proteins during development and decay of thermotolerance. Cancer Res. 1982 Jun;42(6):2457–2461. [PubMed] [Google Scholar]
- Landry J., Chrétien P. Relationship between hyperthermia-induced heat-shock proteins and thermotolerance in Morris hepatoma cells. Can J Biochem Cell Biol. 1983 Jun;61(6):428–437. doi: 10.1139/o83-058. [DOI] [PubMed] [Google Scholar]
- Li G. C. Induction of thermotolerance and enhanced heat shock protein synthesis in Chinese hamster fibroblasts by sodium arsenite and by ethanol. J Cell Physiol. 1983 May;115(2):116–122. doi: 10.1002/jcp.1041150203. [DOI] [PubMed] [Google Scholar]
- Li G. C., Werb Z. Correlation between synthesis of heat shock proteins and development of thermotolerance in Chinese hamster fibroblasts. Proc Natl Acad Sci U S A. 1982 May;79(10):3218–3222. doi: 10.1073/pnas.79.10.3218. [DOI] [PMC free article] [PubMed] [Google Scholar]
- Lim L., Hall C., Leung T., Whatley S. The relationship of the rat brain 68 kDa microtubule-associated protein with synaptosomal plasma membranes and with the Drosophila 70 kDa heat-shock protein. Biochem J. 1984 Dec 1;224(2):677–680. doi: 10.1042/bj2240677. [DOI] [PMC free article] [PubMed] [Google Scholar]
- Lindquist S. The heat-shock response. Annu Rev Biochem. 1986;55:1151–1191. doi: 10.1146/annurev.bi.55.070186.005443. [DOI] [PubMed] [Google Scholar]
- Loomis W. F., Wheeler S. A. Chromatin-associated heat shock proteins of Dictyostelium. Dev Biol. 1982 Apr;90(2):412–418. doi: 10.1016/0012-1606(82)90390-6. [DOI] [PubMed] [Google Scholar]
- Loomis W. F., Wheeler S. Heat shock response of Dictyostelium. Dev Biol. 1980 Oct;79(2):399–408. doi: 10.1016/0012-1606(80)90125-6. [DOI] [PubMed] [Google Scholar]
- McAlister L., Finkelstein D. B. Heat shock proteins and thermal resistance in yeast. Biochem Biophys Res Commun. 1980 Apr 14;93(3):819–824. doi: 10.1016/0006-291x(80)91150-x. [DOI] [PubMed] [Google Scholar]
- Muller W. U., Li G. C., Goldstein L. S. Heat does not induce synthesis of heat shock proteins or thermotolerance in the earliest stage of mouse embryo development. Int J Hyperthermia. 1985 Jan-Mar;1(1):97–102. doi: 10.3109/02656738509029277. [DOI] [PubMed] [Google Scholar]
- Napolitano E. W., Pachter J. S., Chin S. S., Liem R. K. beta-Internexin, a ubiquitous intermediate filament-associated protein. J Cell Biol. 1985 Oct;101(4):1323–1331. doi: 10.1083/jcb.101.4.1323. [DOI] [PMC free article] [PubMed] [Google Scholar]
- Pelham H. R. Speculations on the functions of the major heat shock and glucose-regulated proteins. Cell. 1986 Sep 26;46(7):959–961. doi: 10.1016/0092-8674(86)90693-8. [DOI] [PubMed] [Google Scholar]
- Pelham H. Heat-shock proteins. Coming in from the cold. Nature. 1988 Apr 28;332(6167):776–777. doi: 10.1038/332776a0. [DOI] [PubMed] [Google Scholar]
- Petko L., Lindquist S. Hsp26 is not required for growth at high temperatures, nor for thermotolerance, spore development, or germination. Cell. 1986 Jun 20;45(6):885–894. doi: 10.1016/0092-8674(86)90563-5. [DOI] [PubMed] [Google Scholar]
- Pinhasi-Kimhi O., Michalovitz D., Ben-Zeev A., Oren M. Specific interaction between the p53 cellular tumour antigen and major heat shock proteins. Nature. 1986 Mar 13;320(6058):182–184. doi: 10.1038/320182a0. [DOI] [PubMed] [Google Scholar]
- Plesofsky-Vig N., Brambl R. Heat shock response of Neurospora crassa: protein synthesis and induced thermotolerance. J Bacteriol. 1985 Jun;162(3):1083–1091. doi: 10.1128/jb.162.3.1083-1091.1985. [DOI] [PMC free article] [PubMed] [Google Scholar]
- Plesset J., Palm C., McLaughlin C. S. Induction of heat shock proteins and thermotolerance by ethanol in Saccharomyces cerevisiae. Biochem Biophys Res Commun. 1982 Oct 15;108(3):1340–1345. doi: 10.1016/0006-291x(82)92147-7. [DOI] [PubMed] [Google Scholar]
- Przybytkowski E., Bates J. H., Bates D. A., Mackillop W. J. Thermal adaptation in CHO cells at 40 degrees C: the influence of growth conditions and the role of heat shock proteins. Radiat Res. 1986 Sep;107(3):317–331. [PubMed] [Google Scholar]
- Roccheri M. C., Di Bernardo M. G., Giudice G. Synthesis of heat-shock proteins in developing sea urchins. Dev Biol. 1981 Apr 15;83(1):173–177. doi: 10.1016/s0012-1606(81)80020-6. [DOI] [PubMed] [Google Scholar]
- Subjeck J. R., Sciandra J. J., Johnson R. J. Heat shock proteins and thermotolerance; a comparison of induction kinetics. Br J Radiol. 1982 Aug;55(656):579–584. doi: 10.1259/0007-1285-55-656-579. [DOI] [PubMed] [Google Scholar]
- Taswell C. Limiting dilution assays for the determination of immunocompetent cell frequencies. I. Data analysis. J Immunol. 1981 Apr;126(4):1614–1619. [PubMed] [Google Scholar]
- Ungewickell E. The 70-kd mammalian heat shock proteins are structurally and functionally related to the uncoating protein that releases clathrin triskelia from coated vesicles. EMBO J. 1985 Dec 16;4(13A):3385–3391. doi: 10.1002/j.1460-2075.1985.tb04094.x. [DOI] [PMC free article] [PubMed] [Google Scholar]
- Watson K., Dunlop G., Cavicchioli R. Mitochondrial and cytoplasmic protein syntheses are not required for heat shock acquisition of ethanol and thermotolerance in yeast. FEBS Lett. 1984 Jul 9;172(2):299–302. doi: 10.1016/0014-5793(84)81145-x. [DOI] [PubMed] [Google Scholar]
- Widelitz R. B., Magun B. E., Gerner E. W. Dissociation of 68,000 Mr heat shock protein synthesis from thermotolerance expression in rat fibroblasts. Radiat Res. 1984 Aug;99(2):433–437. [PubMed] [Google Scholar]
- Widelitz R. B., Magun B. E., Gerner E. W. Effects of cycloheximide on thermotolerance expression, heat shock protein synthesis, and heat shock protein mRNA accumulation in rat fibroblasts. Mol Cell Biol. 1986 Apr;6(4):1088–1094. doi: 10.1128/mcb.6.4.1088. [DOI] [PMC free article] [PubMed] [Google Scholar]
- Yamamori T., Yura T. Genetic control of heat-shock protein synthesis and its bearing on growth and thermal resistance in Escherichia coli K-12. Proc Natl Acad Sci U S A. 1982 Feb;79(3):860–864. doi: 10.1073/pnas.79.3.860. [DOI] [PMC free article] [PubMed] [Google Scholar]