Abstract
Activities of glucose 6-phosphate, 6-phosphogluconate, and isocitrate dehydrogenases, together with intermediate levels of the glycolytic pathway and the pentose phosphate cycle, were measured throughout a year in the living bark of poplar (Populus gelrica). Shoots, immediately after budding (early May), contained very high levels of the three enzyme activities, which fell gradually by early or mid-July to a level, roughly equivalent to budding (May) or growing (July) 2-year-old twigs. In September, the former two dehydrogenase activities of the new shoots and 2-year-old twigs began to rise, while the latter activity started to decrease. The rise of the two dehydrogenase activities continued until late November (or early December). The high level of the two dehydrogenase activities lasted until early in April of the following year and then the decrease in the activities began prior to the onset of budding, reaching a low, basal level in early May. The profile of changes in the two dehydrogenase activities appeared to coincide with the increase and decrease of soluble proteins.
Normal concentrations of total hexose phosphates in the glycolytic pathway plus 6-phosphogluconate were found to be 288 to 895 μmoles/kilogram dry weight. During the metabolism transition (September and April), a transient and striking increase of 6-phosphogluconate was observed. In September, 6-phosphogluconate reached a level on the order of 10−4m and was 4 times that of fructose 6-phosphate. The increase in 6-phosphogluconate coincided with the increase in the glucose 6-phosphate dehydrogenase activity. Coincidentally, with the change of 6-phosphogluconate level, a large deviation of the in vivo ratio of fructose 6-phosphate to glucose 6-phosphate from the known equilibrium constant was observed, showing the relation of pentose phosphate cycle enzyme activity to the control of glycolysis. The ratio of glucose 6-phosphate to glucose 1-phosphate deviated from that predicted. These ratios fluctuated throughout the year and were affected by the growth phases. The levels of pentose phosphate cycle metabolites, except for 6-phosphogluconate, in the bark were extremely low.
The level of inorganic phosphate in the living bark throughout the year was dependent upon the growth phases, suggesting the presence of a regulatory mechanism to maintain inorganic phosphate at a given level as the growth phase changed.
Sugar phosphate levels of popular twigs or potato tubers (Solanum tuberosum) remained constant after they were stored for 2 weeks at low temperatures, whereas in sweet potato roots (Ipomoea batatas), the level rose to about 9-fold of the control, indicating the presence of a strict regulatory system for the synthesis and catabolism of sugar phosphate in the former two.
Full text
PDF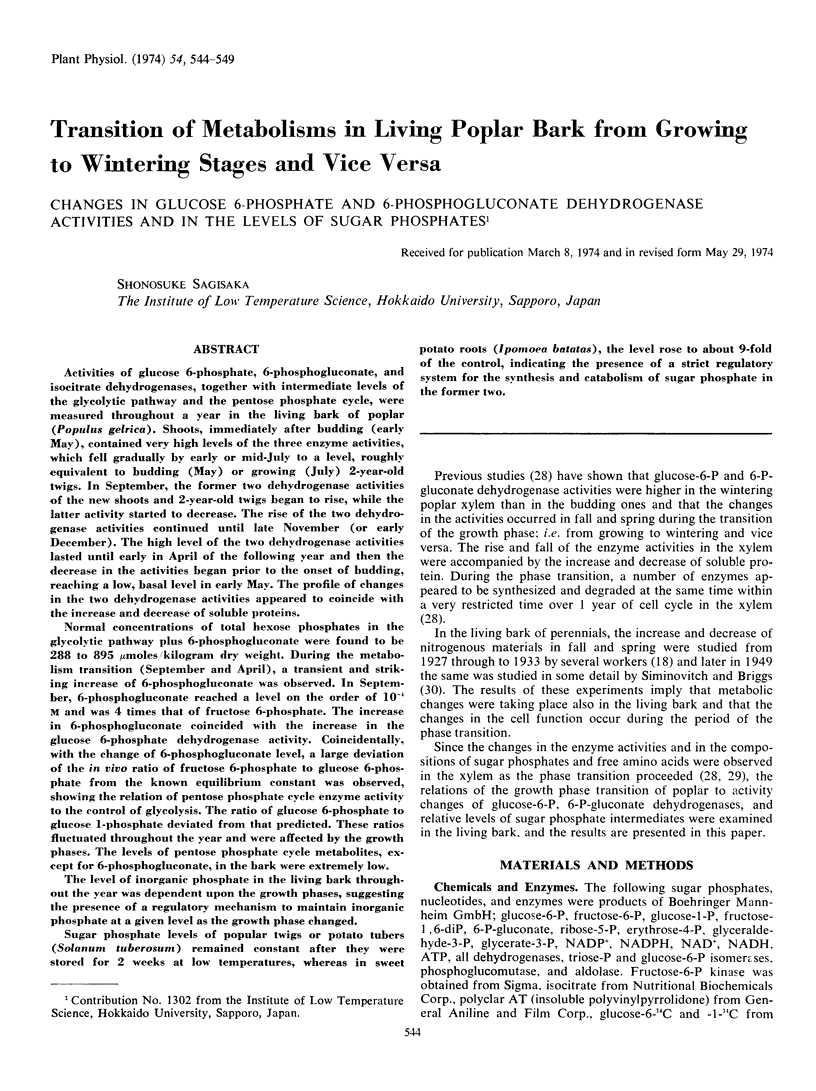
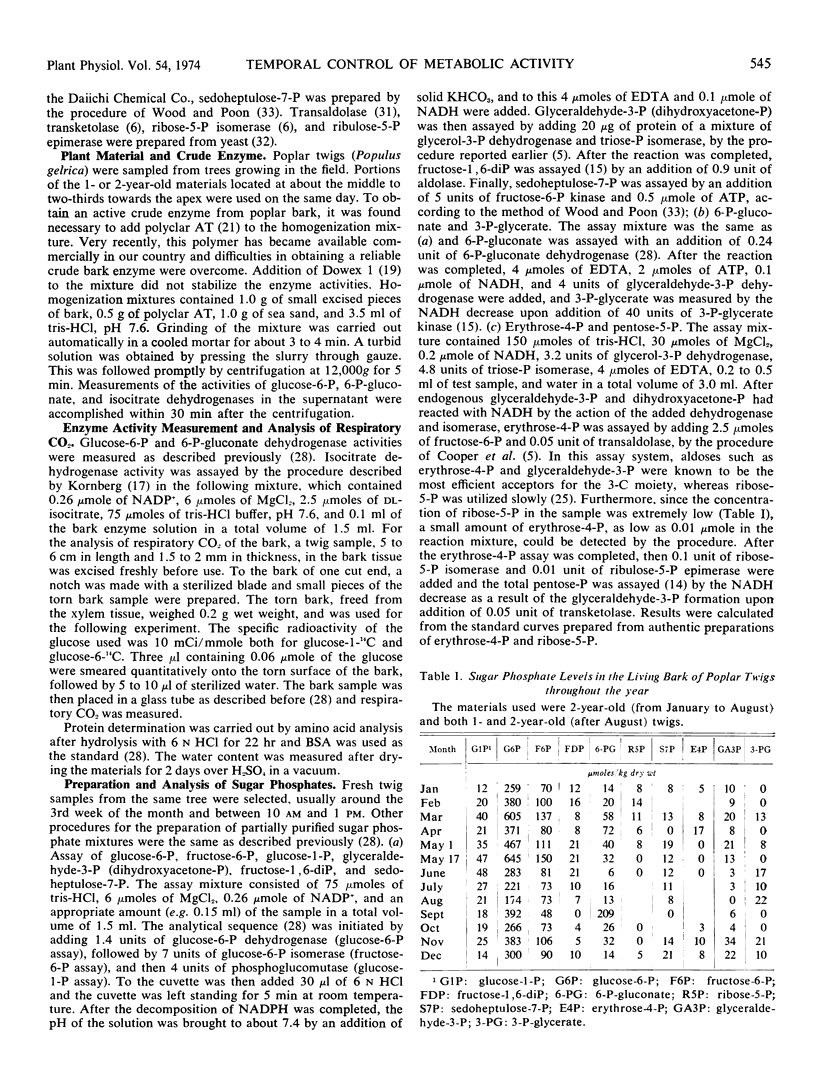
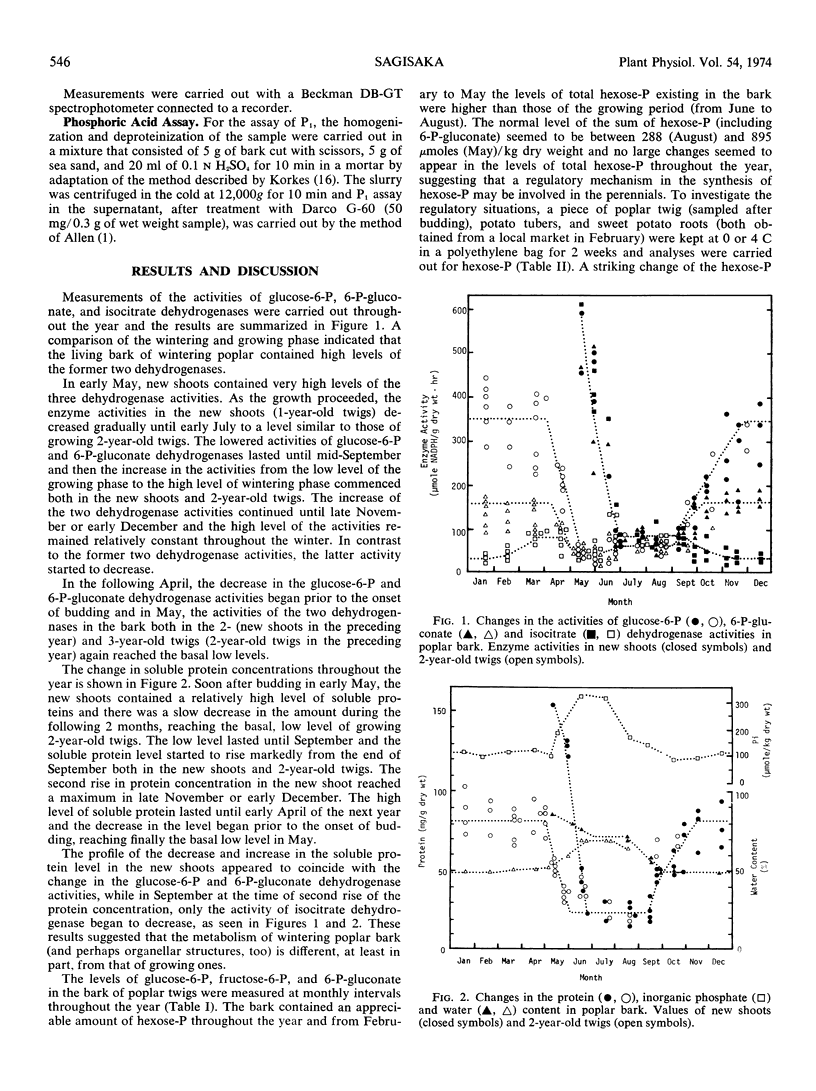
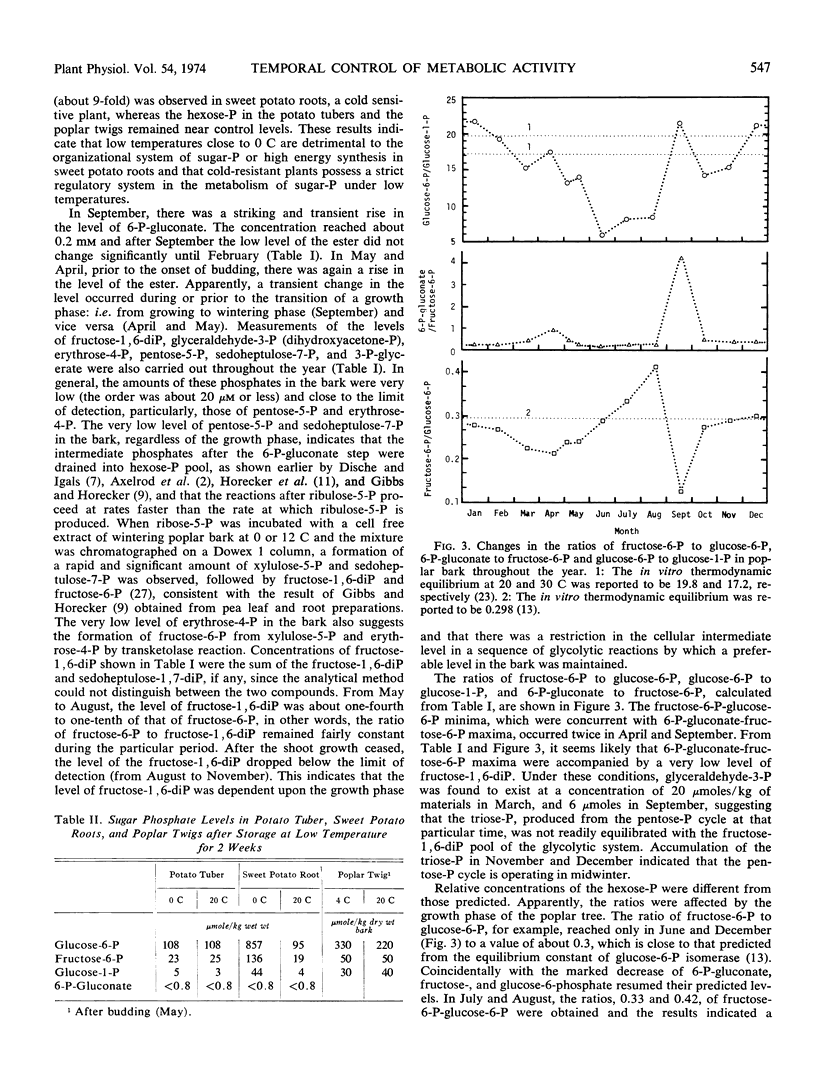
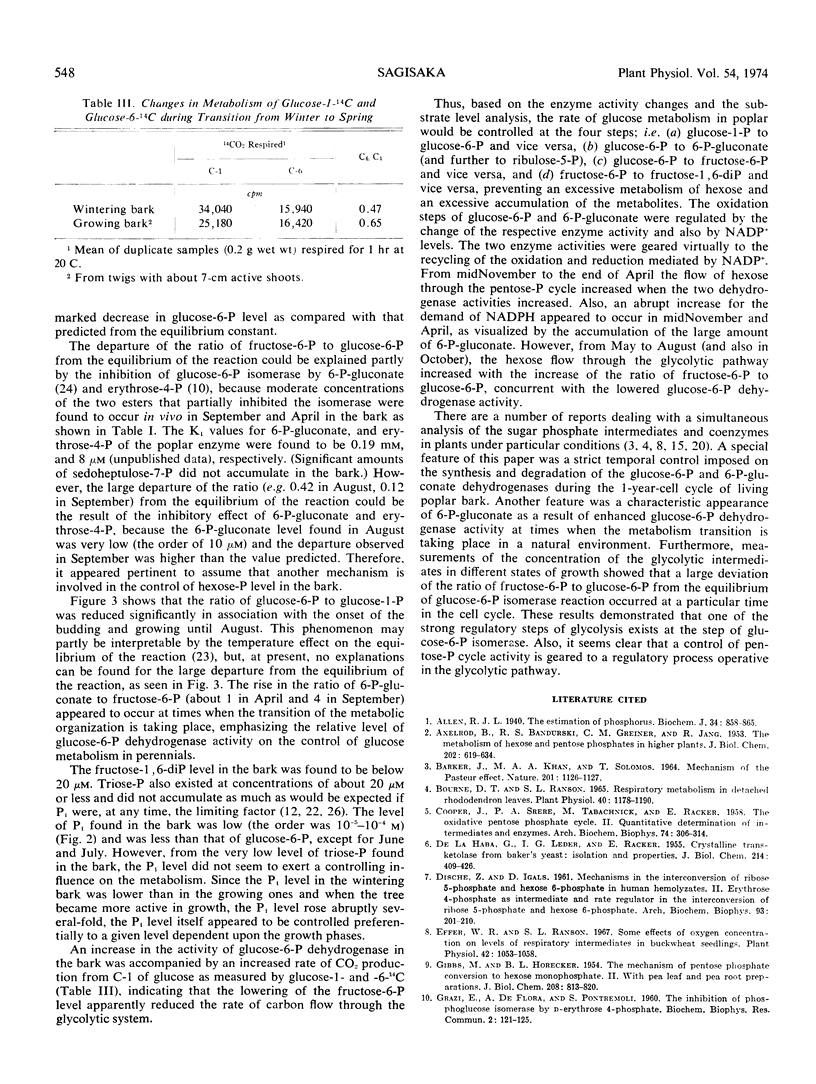
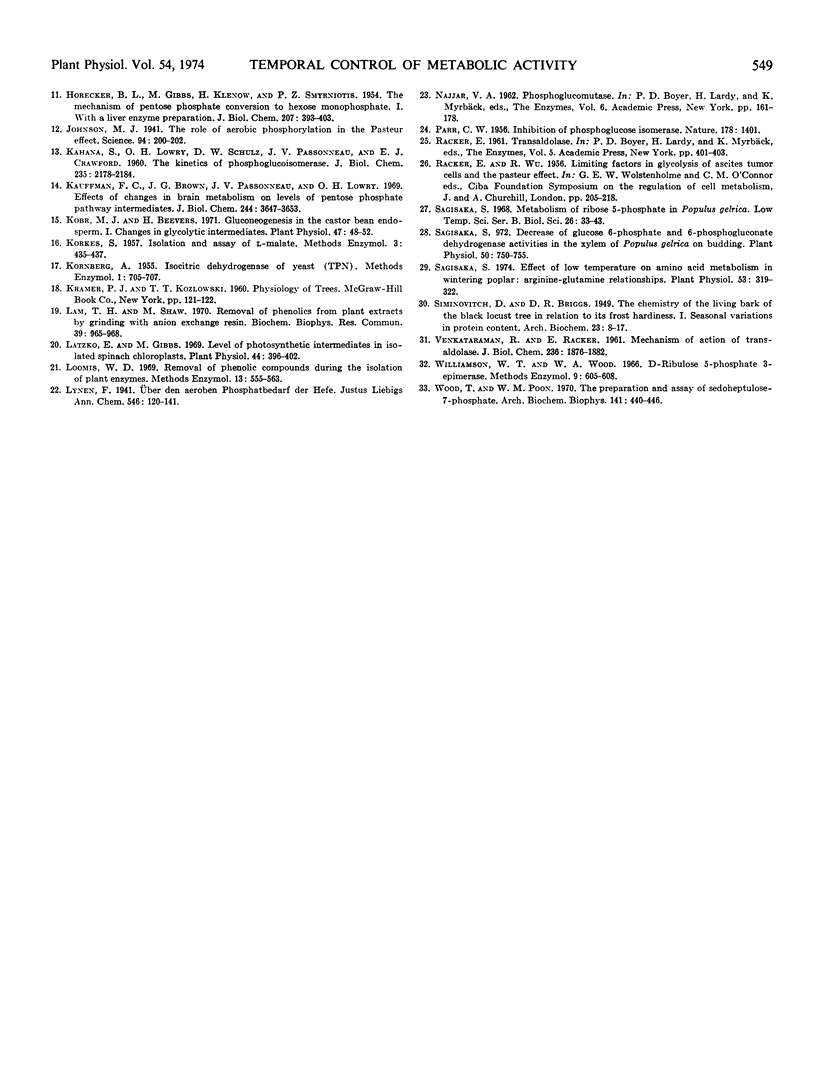
Selected References
These references are in PubMed. This may not be the complete list of references from this article.
- AXELROD B., BANDURSKI R. S., GREINER C. M., JANG R. The metabolism of hexose and pentose phosphates in higher plants. J Biol Chem. 1953 Jun;202(2):619–634. [PubMed] [Google Scholar]
- Allen R. J. The estimation of phosphorus. Biochem J. 1940 Jun;34(6):858–865. doi: 10.1042/bj0340858. [DOI] [PMC free article] [PubMed] [Google Scholar]
- BARKER J., KHAN M. A., SOLOMOS T. MECHANISM OF THE PASTEUR EFFECT. Nature. 1964 Mar 14;201:1126–1127. doi: 10.1038/2011126a0. [DOI] [PubMed] [Google Scholar]
- Bourne D. T., Ranson S. L. Respiratory Metabolism in Detached Rhododendron Leaves. Plant Physiol. 1965 Nov;40(6):1178–1190. doi: 10.1104/pp.40.6.1178. [DOI] [PMC free article] [PubMed] [Google Scholar]
- COOPER J., SRERE P. A., TABACHNICK M., RACKER E. The oxidative pentose phosphate cycle. II. Quantitative determination of intermediates and enzymes. Arch Biochem Biophys. 1958 Apr;74(2):306–314. doi: 10.1016/0003-9861(58)90002-x. [DOI] [PubMed] [Google Scholar]
- DE LA HABA G., LEDER I. G., RACKER E. Crystalline transketolase from bakers' yeast: isolation and properties. J Biol Chem. 1955 May;214(1):409–426. [PubMed] [Google Scholar]
- DISCHE Z., IGALS D. Mechanisms in the interconversion of ribose 5-phosphate and hexose 6-phosphate in human hemolyzates. II. Erythrose 4-phosphate as intermediate and rate regulator in the interconversion of ribose 5-phosphate and hexose 6-phosphate. Arch Biochem Biophys. 1961 May;93:201–210. doi: 10.1016/0003-9861(61)90250-8. [DOI] [PubMed] [Google Scholar]
- Effer W. R., Ranson S. L. Some effects of oxygen concentration on levels of respiratory intermediates in buckwheat seedlings. Plant Physiol. 1967 Aug;42(8):1053–1058. doi: 10.1104/pp.42.8.1053. [DOI] [PMC free article] [PubMed] [Google Scholar]
- GIBBS M., HORECKER B. L. The mechanism of pentose phosphate conversion to hexose monophosphate. II. With pea leaf and pea root preparations. J Biol Chem. 1954 Jun;208(2):813–820. [PubMed] [Google Scholar]
- HORECKER B. L., GIBBS M., KLENOW H., SMYRNIOTIS P. Z. The mechanism of pentose phosphate conversion to hexose monophosphate. I. With a liver enzyme preparation. J Biol Chem. 1954 Mar;207(1):393–403. [PubMed] [Google Scholar]
- Johnson M. J. THE ROLE OF AEROBIC PHOSPHORYLATION IN THE PASTEUR EFFECT. Science. 1941 Aug 29;94(2435):200–202. doi: 10.1126/science.94.2435.200. [DOI] [PubMed] [Google Scholar]
- KAHANA S. E., LOWRY O. H., SCHULZ D. W., PASSONNEAU J. V., CRAWFORD E. J. The kinetics of phosphoglucoisomerase. J Biol Chem. 1960 Aug;235:2178–2184. [PubMed] [Google Scholar]
- Kauffman F. C., Brown J. G., Passonneau J. V., Lowry O. H. Effects of changes in brain metabolism on levels of pentose phosphate pathway intermediates. J Biol Chem. 1969 Jul 10;244(13):3647–3653. [PubMed] [Google Scholar]
- Kobr M. J., Beevers H. Gluconeogenesis in the castor bean endosperm: I. Changes in glycolytic intermediates. Plant Physiol. 1971 Jan;47(1):48–52. doi: 10.1104/pp.47.1.48. [DOI] [PMC free article] [PubMed] [Google Scholar]
- Lam T. H., Shaw M. Removal of phenolics from plant extracts by grinding with anion exchange resin. Biochem Biophys Res Commun. 1970 Jun 5;39(5):965–968. doi: 10.1016/0006-291x(70)90418-3. [DOI] [PubMed] [Google Scholar]
- Latzko E., Gibbs M. Level of photosynthetic intermediates in isolated spinach chloroplasts. Plant Physiol. 1969 Mar;44(3):396–402. doi: 10.1104/pp.44.3.396. [DOI] [PMC free article] [PubMed] [Google Scholar]
- PARR C. W. Inhibition of phosphoglucose isomerase. Nature. 1956 Dec 22;178(4547):1401–1401. doi: 10.1038/1781401a0. [DOI] [PubMed] [Google Scholar]
- Sagisaka S. Effect of low temperature on amino Acid metabolism in wintering poplar: arginine-glutamine relationships. Plant Physiol. 1974 Feb;53(2):319–322. doi: 10.1104/pp.53.2.319. [DOI] [PMC free article] [PubMed] [Google Scholar]
- VENKATARAMAN R., RACKER E. Mechanism of action of transaldolase. I. Crystalization and properties of yeast enzyme. J Biol Chem. 1961 Jul;236:1876–1882. [PubMed] [Google Scholar]
- Wood T., Poon W. M. The preparation and assay of sedoheptulose-7-phosphate. Arch Biochem Biophys. 1970 Dec;141(2):440–446. doi: 10.1016/0003-9861(70)90160-8. [DOI] [PubMed] [Google Scholar]