Abstract
Brush border membrane vesicles were isolated from rabbit renal cortex by Mg++-precipitation and differential centrifugation. 36Cl− and [3H]glucose uptakes were simultaneously determined by a rapid filtration technique. Lysis of the vesicles with distilled water abolished 90-95% of the radioactivity on the filters, suggesting that nearly all of the 36Cl− and [3H]glucose counts represented uptake into an osmotically reactive intravesicular space. Inwardly directed K+ gradients plus valinomycin stimulated 36Cl− uptake, demonstrating a conductive pathway for chloride uptake into brush-border membrane vesicles. 36Cl− uptake could also be stimulated by inwardly directed proton gradients (pHoutside < pHinside). This effect was seen in the absence of sodium, as well as in the presence of valinomycin when the vesicles had equal K+ concentrations inside and out. An “overshoot” phenomenon was observed when external 36Cl− was 2 mM and the external pH was lowered from 7.5 to 6.0 or to 4.5. The effect of the proton gradient was presumed to be different from the conductive mechanism because (a) the stimulation of 36Cl− uptake by inwardly directed K+ diffusion potentials was additive to the proton gradient effect, and (b) competition studies revealed statistically significant effects of thiocyanate on the conductive pathway, but not on the proton-driven pathway.
HCl cotransport or anion exchange are electrically neutral mechanisms which could couple 36Cl− uptake to inwardly-directed proton gradients in a brush border membrane vesicle. If both electrically neutral and conductive path ways for chloride transport are present in the luminal membrane of the proximal tubule, then the mechanism as well as the direction of net chloride transport will be influenced by the nature of the accompanying cation transport process.
Full text
PDF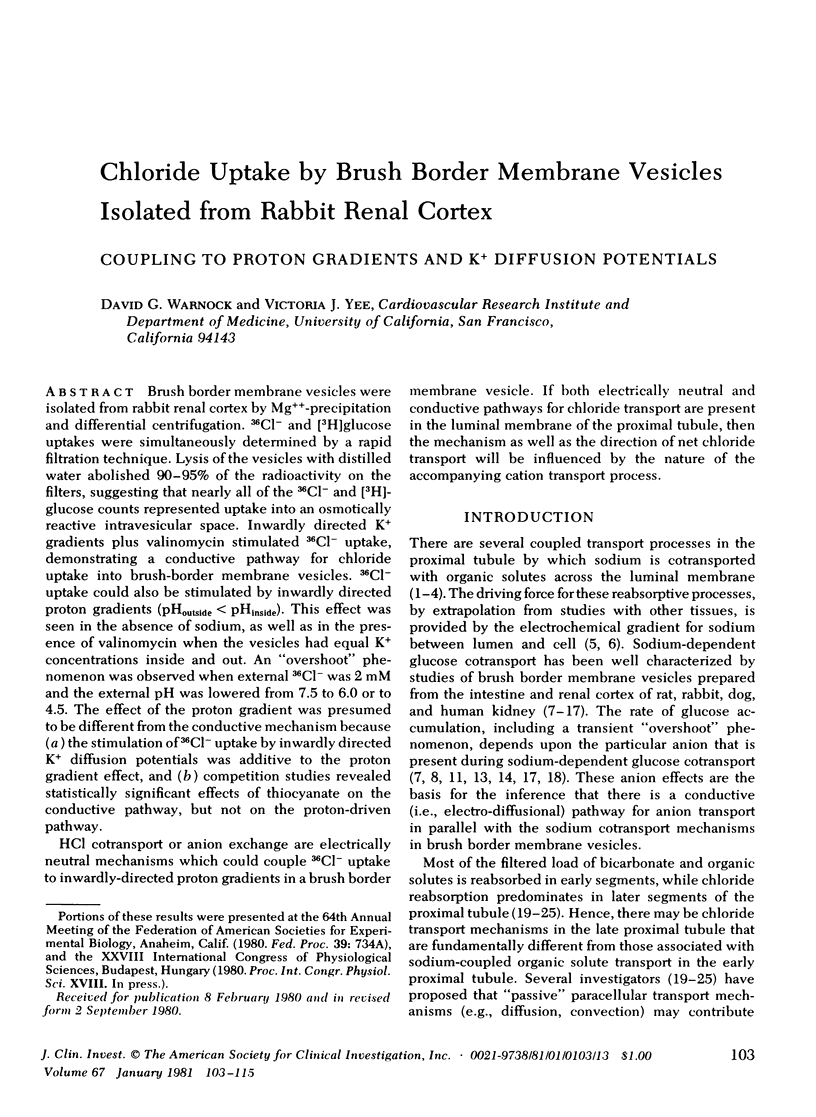
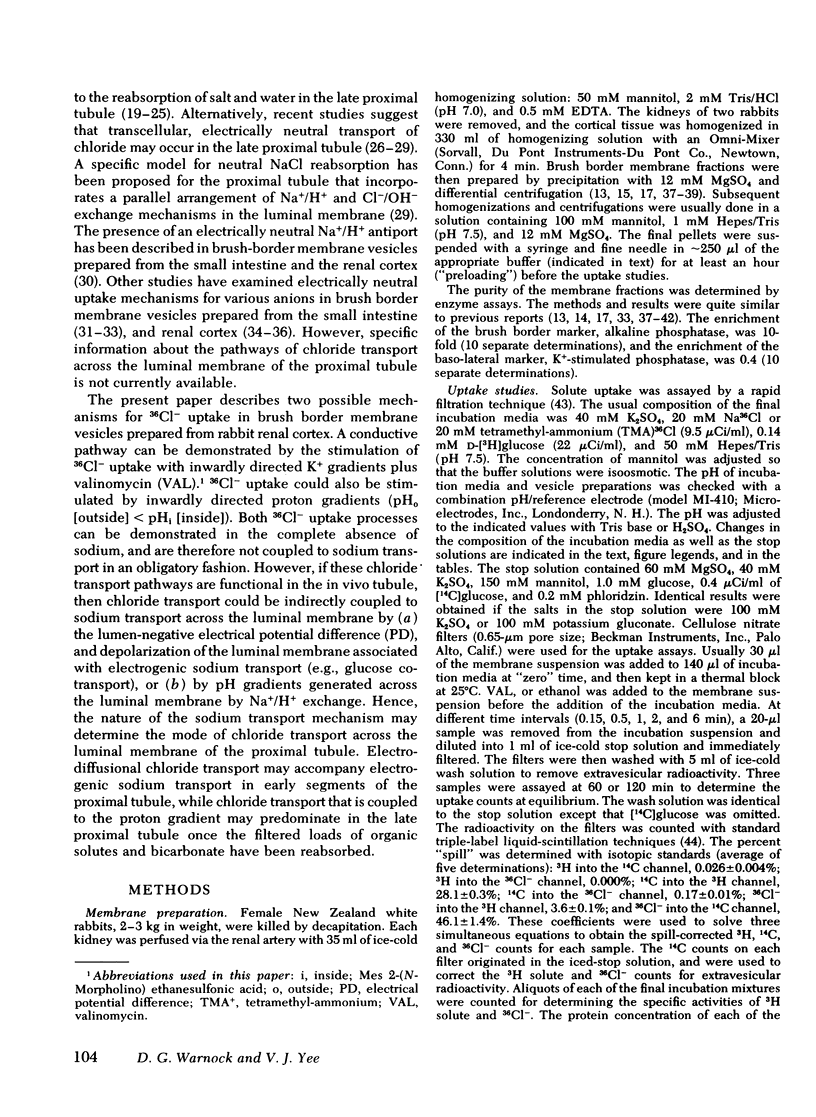
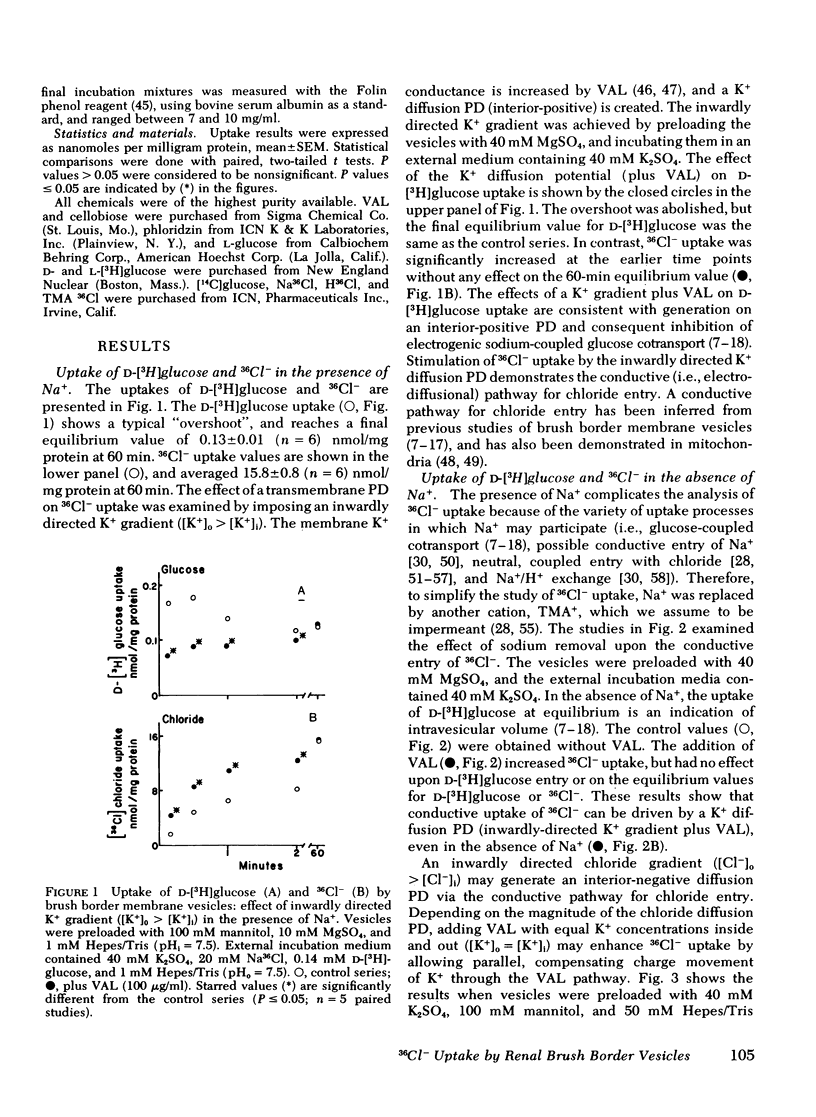
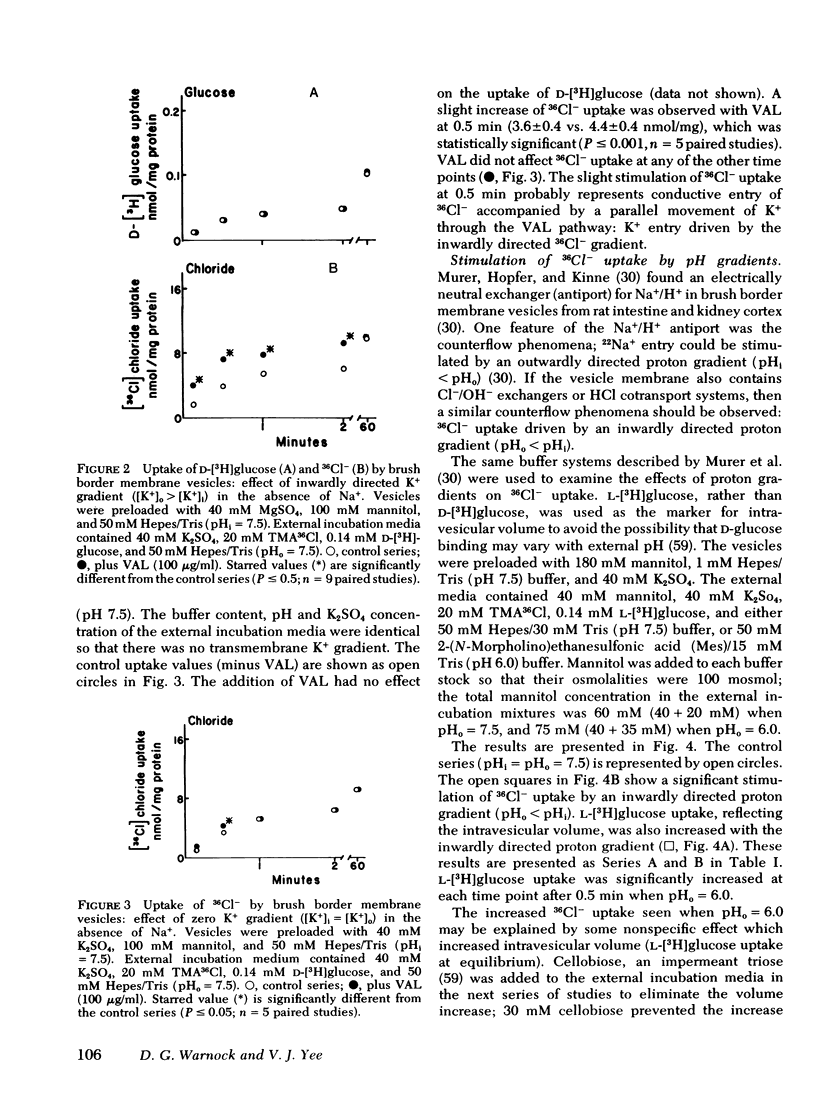
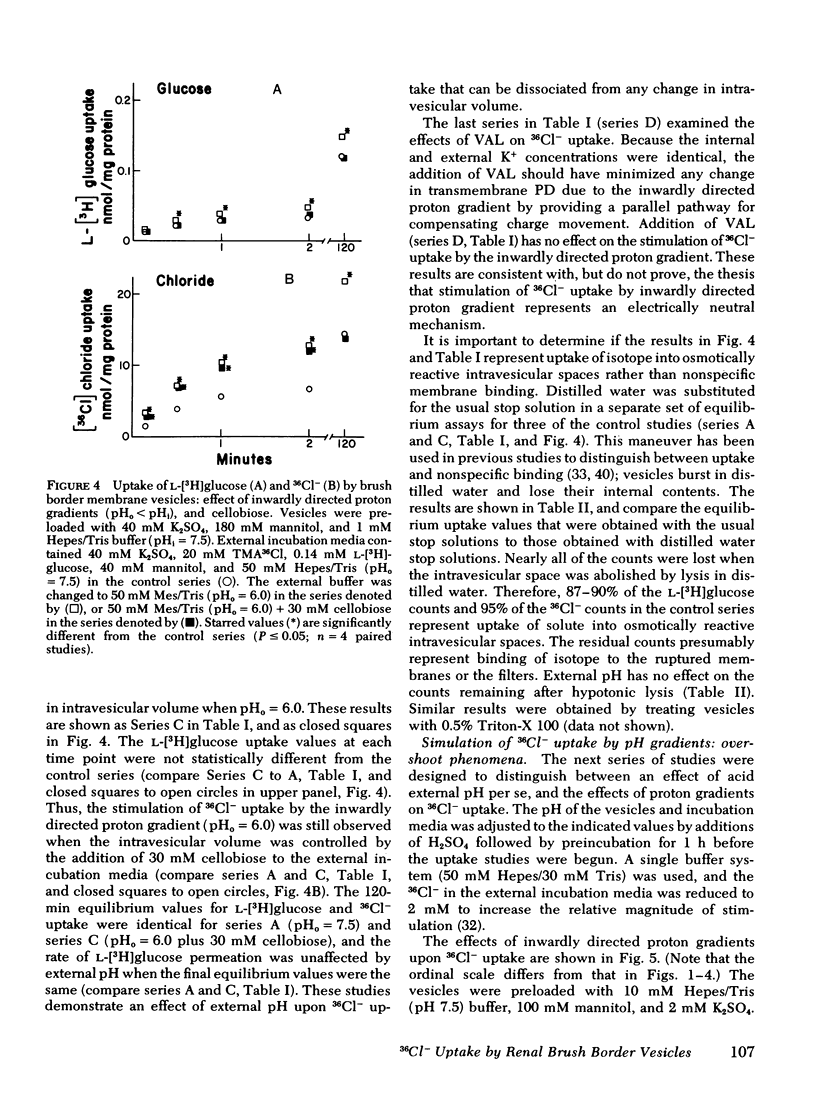
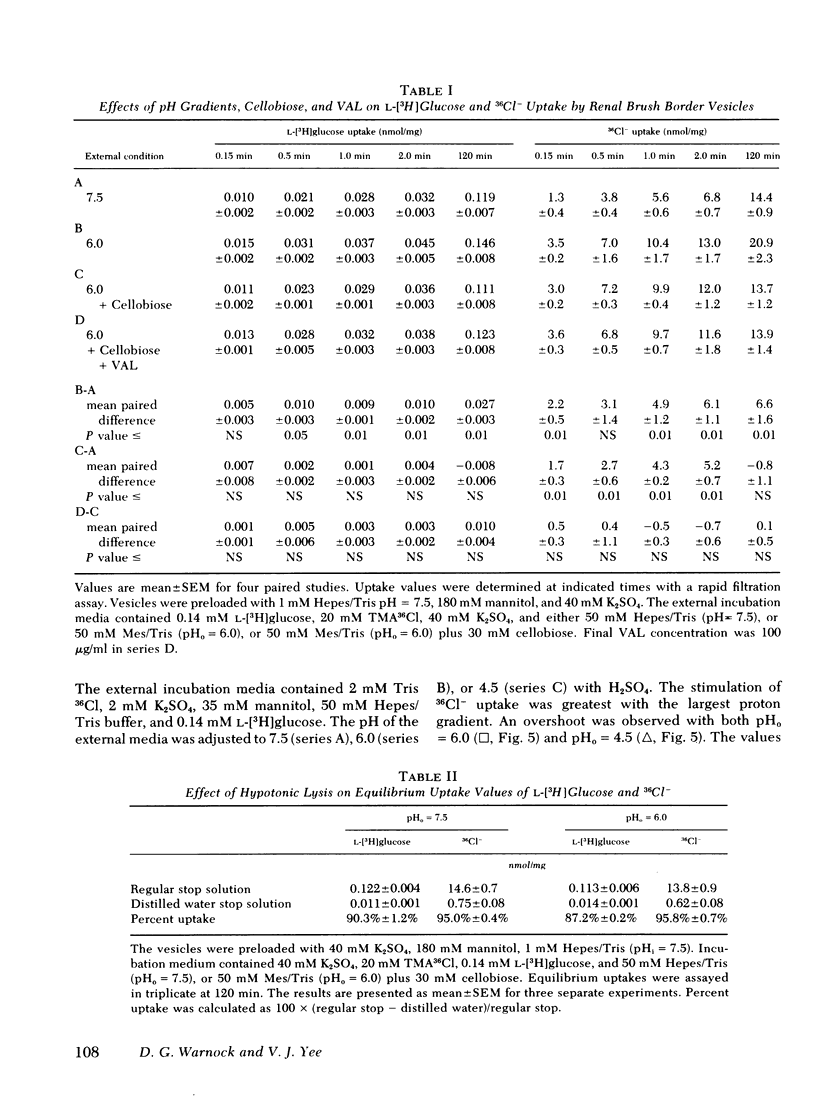
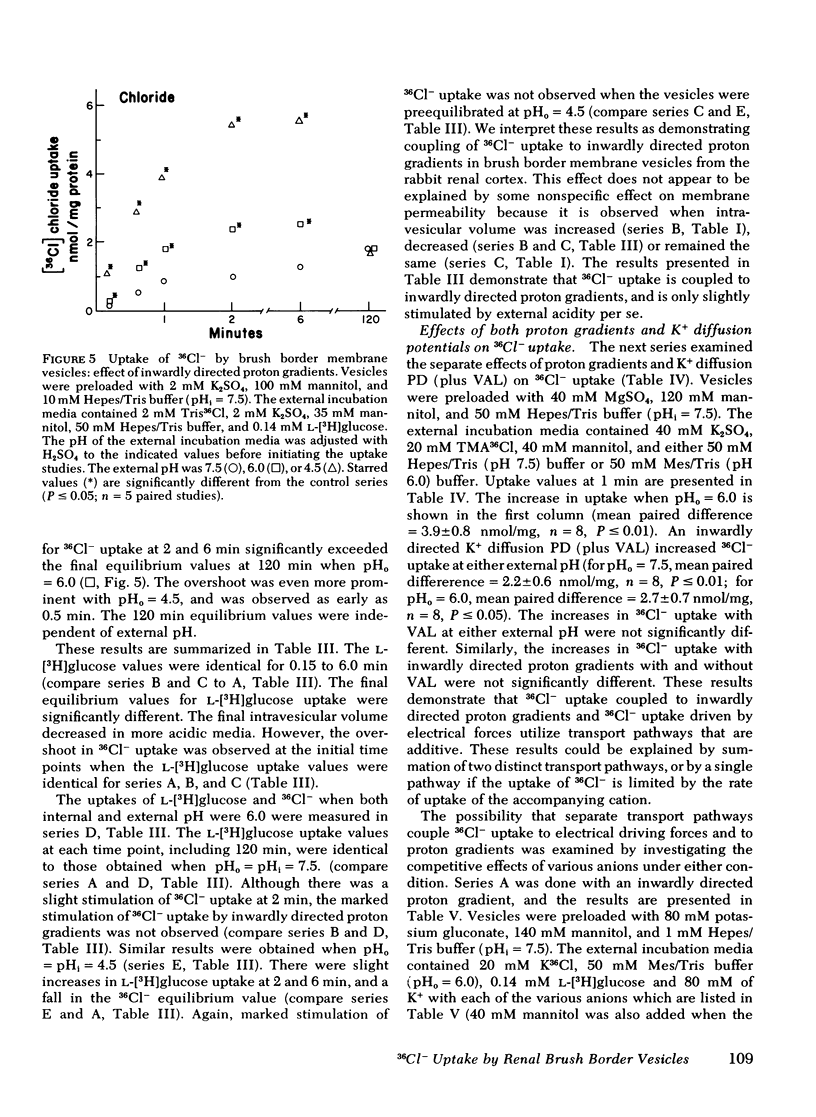
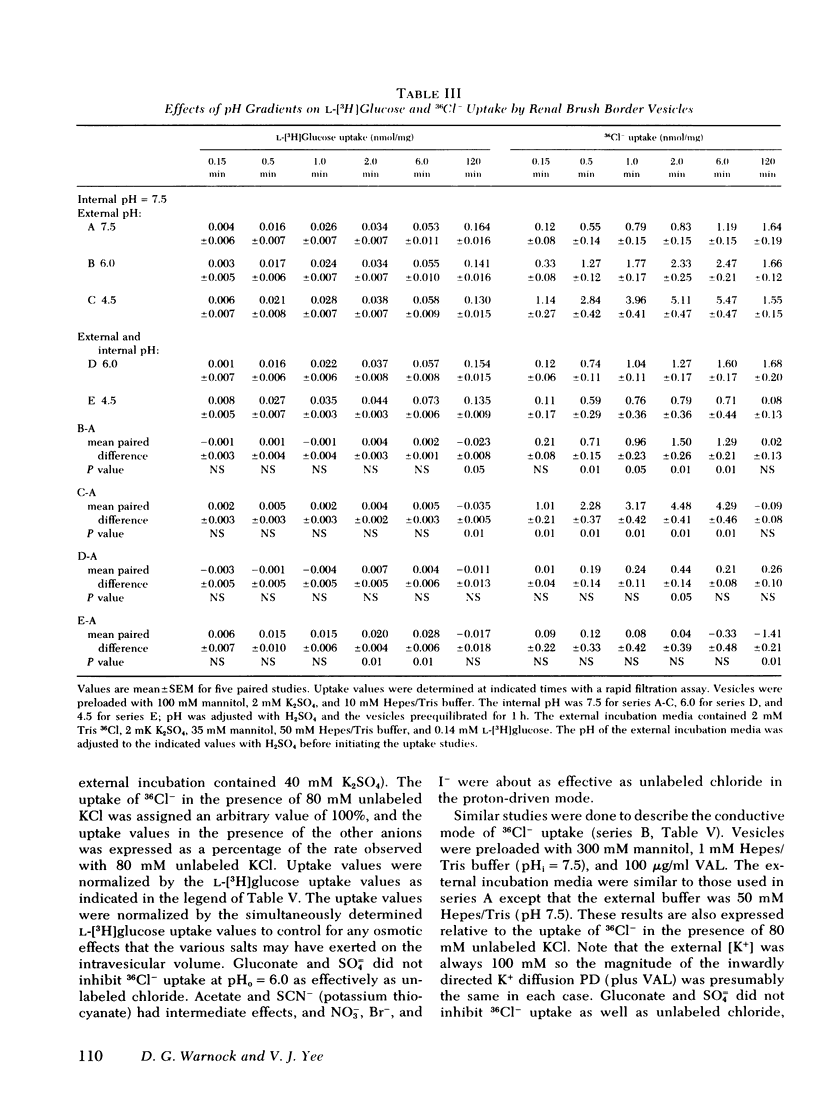
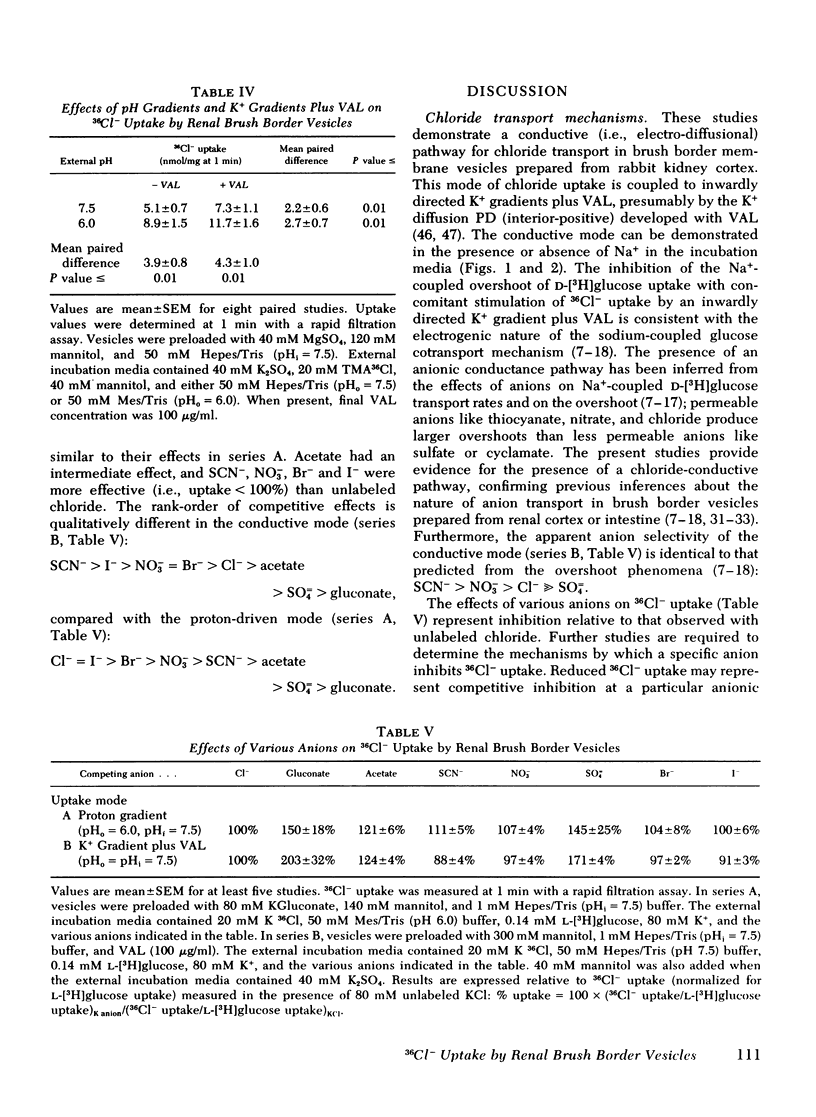
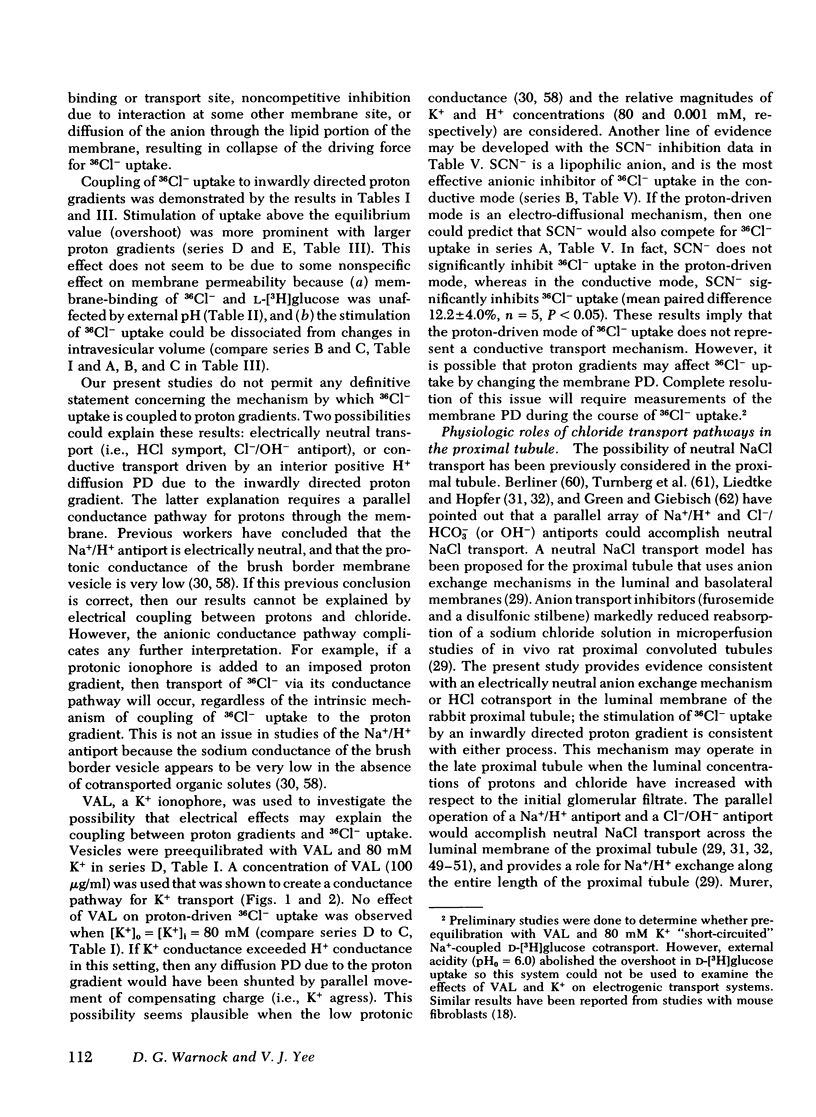
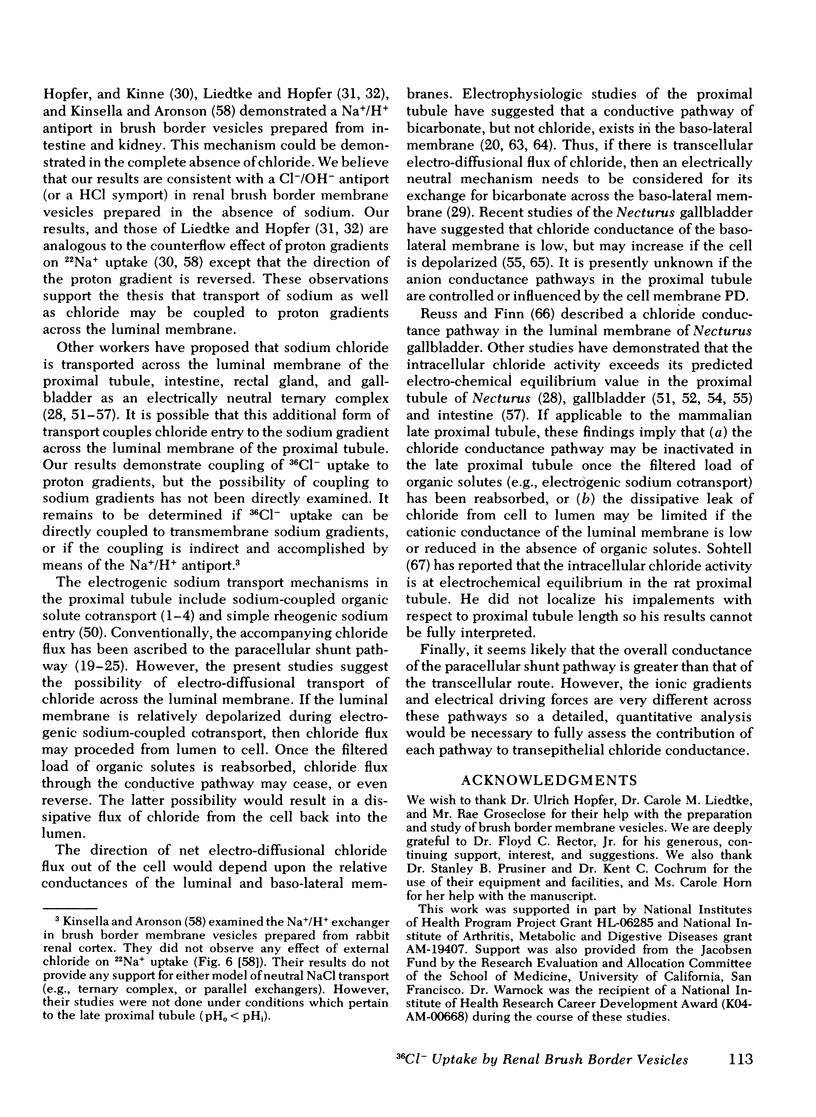
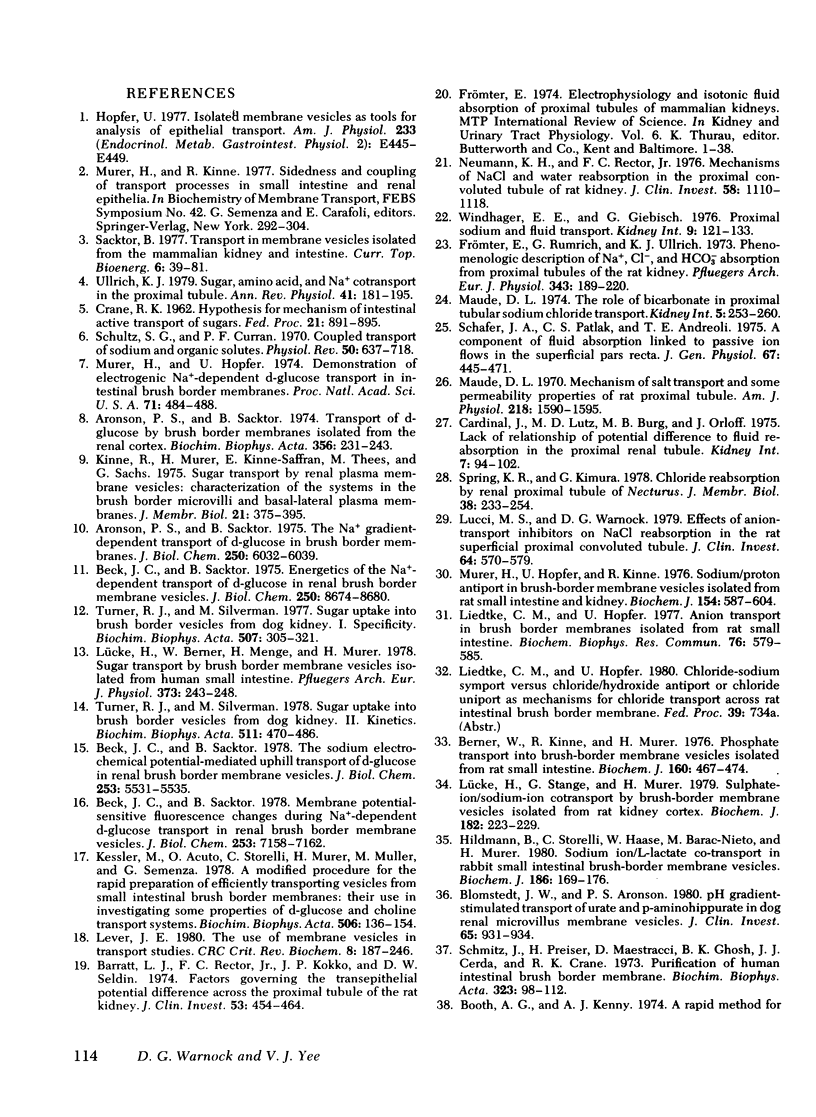
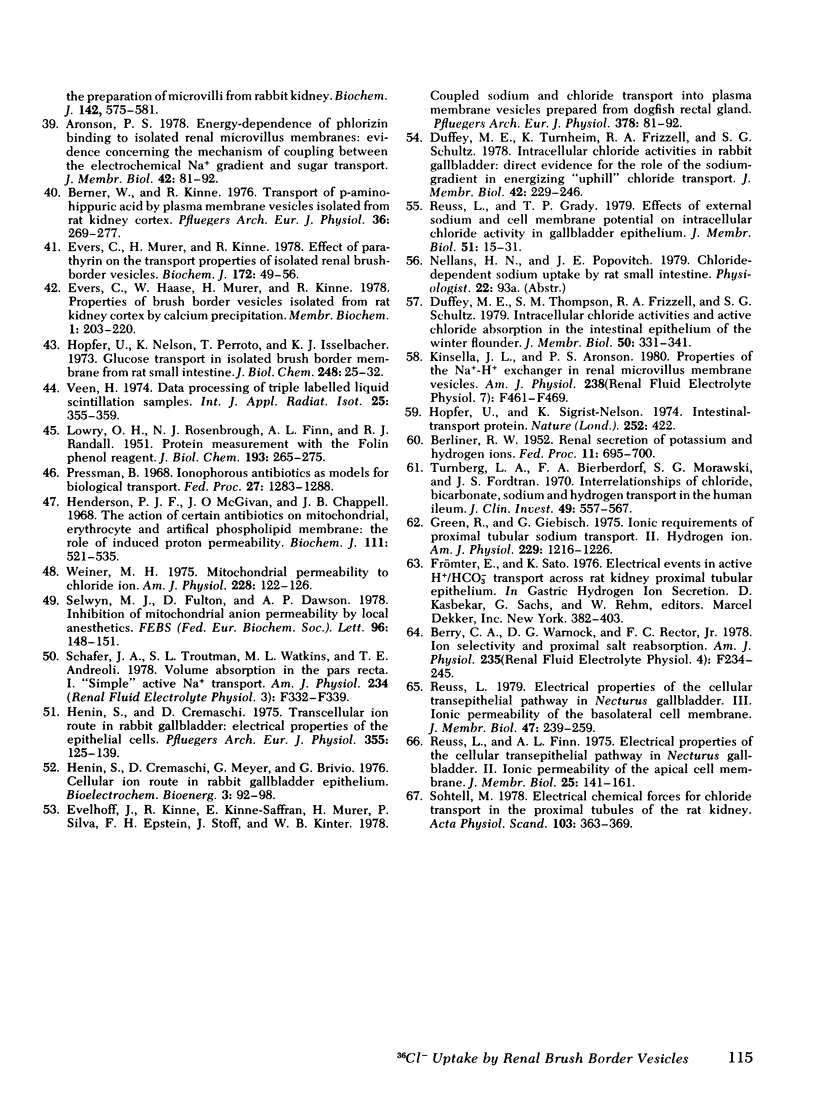
Selected References
These references are in PubMed. This may not be the complete list of references from this article.
- Aronson P. S. Energy-dependence of phlorizin binding to isolated renal microvillus membranes. Evidence concerning the mechanism of coupling between the electrochemical Na+ gradient the sugar transport. J Membr Biol. 1978 Jul 21;42(1):81–98. doi: 10.1007/BF01870395. [DOI] [PubMed] [Google Scholar]
- Aronson P. S., Sacktor B. The Na+ gradient-dependent transport of D-glucose in renal brush border membranes. J Biol Chem. 1975 Aug 10;250(15):6032–6039. [PubMed] [Google Scholar]
- Aronson P. S., Sacktor B. Transport of D-glucose by brush border membranes isolated from the renal cortex. Biochim Biophys Acta. 1974 Jul 31;356(2):231–243. doi: 10.1016/0005-2736(74)90286-7. [DOI] [PubMed] [Google Scholar]
- BERLINER R. W. Renal secretion of potassium and hydrogen ions. Fed Proc. 1952 Sep;11(3):695–700. [PubMed] [Google Scholar]
- Barratt L. J., Rector F. C., Jr, Kokko J. P., Seldin D. W. Factors governing the transepithelial potential difference across the proximal tubule of the rat kidney. J Clin Invest. 1974 Feb;53(2):454–464. doi: 10.1172/JCI107579. [DOI] [PMC free article] [PubMed] [Google Scholar]
- Beck J. C., Sacktor B. Energetics of the Na+-dependent transport of D-glucose in renal brush border membrane vesicles. J Biol Chem. 1975 Nov 25;250(22):8674–8680. [PubMed] [Google Scholar]
- Beck J. C., Sacktor B. Membrane potential-sensitive fluorescence changes during Na+-dependent D-glucose transport in renal brush border membrane vesicles. J Biol Chem. 1978 Oct 25;253(20):7158–7162. [PubMed] [Google Scholar]
- Beck J. C., Sacktor B. The sodium electrochemical potential-mediated uphill transport of D-glucose in renal brush border membrane vesicles. J Biol Chem. 1978 Aug 10;253(15):5531–5535. [PubMed] [Google Scholar]
- Berner W., Kinne R., Murer H. Phosphate transport into brush-border membrane vesicles isolated from rat small intestine. Biochem J. 1976 Dec 15;160(3):467–474. doi: 10.1042/bj1600467. [DOI] [PMC free article] [PubMed] [Google Scholar]
- Berner W., Kinne R. Transport of p-aminohippuric acid by plasma membrane vesicles isolated from rat kidney cortex. Pflugers Arch. 1976 Feb 24;361(3):269–277. doi: 10.1007/BF00587292. [DOI] [PubMed] [Google Scholar]
- Berry C. A., Warnock D. G., Rector F. C., Jr Ion selectivity and proximal salt reabsorption. Am J Physiol. 1978 Sep;235(3):F234–F245. doi: 10.1152/ajprenal.1978.235.3.F234. [DOI] [PubMed] [Google Scholar]
- Blomstedt J. W., Aronson P. S. pH gradient-stimulated transport of urate and p-aminohippurate in dog renal microvillus membrane vesicles. J Clin Invest. 1980 Apr;65(4):931–934. doi: 10.1172/JCI109748. [DOI] [PMC free article] [PubMed] [Google Scholar]
- CRANE R. K. Hypothesis for mechanism of intestinal active transport of sugars. Fed Proc. 1962 Nov-Dec;21:891–895. [PubMed] [Google Scholar]
- Cardinal J., Lutz M. D., Burg M. B., Orloff J. Lack of relationship of potential difference to fluid absorption in the proximal renal tubule. Kidney Int. 1975 Feb;7(2):94–102. doi: 10.1038/ki.1975.14. [DOI] [PubMed] [Google Scholar]
- Duffey M. E., Thompson S. M., Frizzell R. A., Schultz S. G. Intracellular chloride activities and active chloride absorption in the intestinal epithelium of the winter flounder. J Membr Biol. 1979 Nov 30;50(3-4):331–341. doi: 10.1007/BF01868896. [DOI] [PubMed] [Google Scholar]
- Duffey M. E., Turnheim K., Frizzell R. A., Schultz S. G. Intracellular chloride activities in rabbit gallbladder: direct evidence for the role of the sodium-gradient in energizing "uphill" chloride transport. J Membr Biol. 1978 Sep 19;42(3):229–245. doi: 10.1007/BF01870360. [DOI] [PubMed] [Google Scholar]
- Eveloff J., Kinne R., Kinne-Saffran E., Murer H., Silva P., Epstein F. H., Stoff J., Kinter W. B. Coupled sodium and chloride transport into plasma membrane vesicles prepared from dogfish rectal gland. Pflugers Arch. 1978 Dec 28;378(2):87–92. doi: 10.1007/BF00584439. [DOI] [PubMed] [Google Scholar]
- Evers C., Haase W., Murer H., Kinne R. Properties of brush border vesicles isolated from rat kidney cortex by calcium precipitation. Membr Biochem. 1978;1(3-4):203–219. doi: 10.3109/09687687809063848. [DOI] [PubMed] [Google Scholar]
- Evers C., Murer H., Kinne R. Effect of parathyrin on the transport properties of isolated renal brush-border vesicles. Biochem J. 1978 Apr 15;172(1):49–56. doi: 10.1042/bj1720049. [DOI] [PMC free article] [PubMed] [Google Scholar]
- Frömter E., Rumrich G., Ullrich K. J. Phenomenologic description of Na+, Cl- and HCO-3 absorption from proximal tubules of rat kidney. Pflugers Arch. 1973 Oct 22;343(3):189–220. doi: 10.1007/BF00586045. [DOI] [PubMed] [Google Scholar]
- Green R., Giebisch G. Ionic requirements of proximal tubular sodium transport. II. Hydrogen ion. Am J Physiol. 1975 Nov;229(5):1216–1226. doi: 10.1152/ajplegacy.1975.229.5.1216. [DOI] [PubMed] [Google Scholar]
- Henderson P. J., McGivan J. D., Chappell J. B. The action of certain antibiotics on mitochondrial, erythrocyte and artificial phospholipid membranes. The role of induced proton permeability. Biochem J. 1969 Feb;111(4):521–535. doi: 10.1042/bj1110521. [DOI] [PMC free article] [PubMed] [Google Scholar]
- Hildmann B., Storelli C., Haase W., Barac-Nieto M., Murer H. Sodium ion/L-lactate co-transport in rabbit small-intestinal brush-border-membrane vesicles. Biochem J. 1980 Jan 15;186(1):169–176. doi: 10.1042/bj1860169. [DOI] [PMC free article] [PubMed] [Google Scholar]
- Hopfer U. Isolated membrane vesicles as tools for analysis of epithelial transport. Am J Physiol. 1977 Dec;233(6):E445–E449. doi: 10.1152/ajpendo.1977.233.6.E445. [DOI] [PubMed] [Google Scholar]
- Hopfer U., Nelson K., Perrotto J., Isselbacher K. J. Glucose transport in isolated brush border membrane from rat small intestine. J Biol Chem. 1973 Jan 10;248(1):25–32. [PubMed] [Google Scholar]
- Hopfer U., Sigrist-Nelson K. Letter: Intestinal transport protein. Nature. 1974 Nov 29;252(5482):422–422. doi: 10.1038/252422a0. [DOI] [PubMed] [Google Scholar]
- Hénin S., Cremaschi D. Transcellular ion route in rabbit gallbladder. Electric properties of the epithelial cells. Pflugers Arch. 1975;355(2):125–139. doi: 10.1007/BF00581828. [DOI] [PubMed] [Google Scholar]
- Kessler M., Acuto O., Storelli C., Murer H., Müller M., Semenza G. A modified procedure for the rapid preparation of efficiently transporting vesicles from small intestinal brush border membranes. Their use in investigating some properties of D-glucose and choline transport systems. Biochim Biophys Acta. 1978 Jan 4;506(1):136–154. doi: 10.1016/0005-2736(78)90440-6. [DOI] [PubMed] [Google Scholar]
- Kinsella J. L., Aronson P. S. Properties of the Na+-H+ exchanger in renal microvillus membrane vesicles. Am J Physiol. 1980 Jun;238(6):F461–F469. doi: 10.1152/ajprenal.1980.238.6.F461. [DOI] [PubMed] [Google Scholar]
- LOWRY O. H., ROSEBROUGH N. J., FARR A. L., RANDALL R. J. Protein measurement with the Folin phenol reagent. J Biol Chem. 1951 Nov;193(1):265–275. [PubMed] [Google Scholar]
- Lever J. E. The use of membrane vesicles in transport studies. CRC Crit Rev Biochem. 1980 Jan;7(3):187–246. doi: 10.3109/10409238009105462. [DOI] [PubMed] [Google Scholar]
- Liedtke C. M., Hopfer U. Anion transport in brush border membranes isolated from rat small intestine. Biochem Biophys Res Commun. 1976 May 23;76(2):579–585. doi: 10.1016/0006-291x(77)90763-x. [DOI] [PubMed] [Google Scholar]
- Lucci M. S., Warnock D. G. Effects of anion-transport inhibitors on NaCl reabsorption in the rat superficial proximal convoluted tubule. J Clin Invest. 1979 Aug;64(2):570–579. doi: 10.1172/JCI109495. [DOI] [PMC free article] [PubMed] [Google Scholar]
- Lúcke H., Berner W., Menge H., Murer H. Sugar transport by brush border membrane vesicles isolated from human small intestine. Pflugers Arch. 1978 Mar 20;373(3):243–248. doi: 10.1007/BF00580831. [DOI] [PubMed] [Google Scholar]
- Lücke H., Stange G., Murer H. Sulphate-ion/sodium-ion co-transport by brush-border membrane vesicles isolated from rat kidney cortex. Biochem J. 1979 Jul 15;182(1):223–229. doi: 10.1042/bj1820223. [DOI] [PMC free article] [PubMed] [Google Scholar]
- Maude D. L. Mechanism of salt transport and some permeability properties of rat proximal tubule. Am J Physiol. 1970 Jun;218(6):1590–1595. doi: 10.1152/ajplegacy.1970.218.6.1590. [DOI] [PubMed] [Google Scholar]
- Maude D. L. The role of bicarbonate in proximal tubular sodium chloride transport. Kidney Int. 1974 Apr;5(4):253–260. doi: 10.1038/ki.1974.34. [DOI] [PubMed] [Google Scholar]
- Murer H., Hopfer U. Demonstration of electrogenic Na+-dependent D-glucose transport in intestinal brush border membranes. Proc Natl Acad Sci U S A. 1974 Feb;71(2):484–488. doi: 10.1073/pnas.71.2.484. [DOI] [PMC free article] [PubMed] [Google Scholar]
- Murer H., Hopfer U., Kinne R. Sodium/proton antiport in brush-border-membrane vesicles isolated from rat small intestine and kidney. Biochem J. 1976 Mar 15;154(3):597–604. [PMC free article] [PubMed] [Google Scholar]
- Neumann K. H., Rector F. C., Jr Mechanism of NaCl and water reabsorption in the proximal convoluted tubule of rat kidney. J Clin Invest. 1976 Nov;58(5):1110–1118. doi: 10.1172/JCI108563. [DOI] [PMC free article] [PubMed] [Google Scholar]
- Pressman B. C. Ionophorous antibiotics as models for biological transport. Fed Proc. 1968 Nov-Dec;27(6):1283–1288. [PubMed] [Google Scholar]
- Reuss L. Electrical properties of the cellular transepithelial pathway in Necturus gallbladder: III. Ionic permeability of the basolateral cell membrane. J Membr Biol. 1979 May 25;47(3):239–259. doi: 10.1007/BF01869080. [DOI] [PubMed] [Google Scholar]
- Reuss L., Finn A. L. Electrical properties of the cellular transepithelial pathway in Necturus gallbladder. II. Ionic permeability of the apical cell membrane. J Membr Biol. 1975 Dec 4;25(1-2):141–161. doi: 10.1007/BF01868572. [DOI] [PubMed] [Google Scholar]
- Reuss L., Grady T. P. Effects of external sodium and cell membrane potential on intracellular chloride activity in gallbladder epithelium. J Membr Biol. 1979 Dec 12;51(1):15–31. doi: 10.1007/BF01869341. [DOI] [PubMed] [Google Scholar]
- Schafer J. A., Patlak C. S., Andreoli T. E. A component of fluid absorption linked to passive ion flows in the superficial pars recta. J Gen Physiol. 1975 Oct;66(4):445–471. doi: 10.1085/jgp.66.4.445. [DOI] [PMC free article] [PubMed] [Google Scholar]
- Schafer J. A., Troutman S. L., Watkins M. L., Andreoli T. E. Volume absorption in the pars recta. I. "Simple" active Na+ transport. Am J Physiol. 1978 Apr;234(4):F332–F339. doi: 10.1152/ajprenal.1978.234.4.F332. [DOI] [PubMed] [Google Scholar]
- Schmitz J., Preiser H., Maestracci D., Ghosh B. K., Cerda J. J., Crane R. K. Purification of the human intestinal brush border membrane. Biochim Biophys Acta. 1973 Sep 27;323(1):98–112. doi: 10.1016/0005-2736(73)90434-3. [DOI] [PubMed] [Google Scholar]
- Schultz S. G., Curran P. F. Coupled transport of sodium and organic solutes. Physiol Rev. 1970 Oct;50(4):637–718. doi: 10.1152/physrev.1970.50.4.637. [DOI] [PubMed] [Google Scholar]
- Sohtell M. Electrochemical forces for chloride transport in the proximal tubules of the rat kidney. Acta Physiol Scand. 1978 Aug;103(4):363–369. doi: 10.1111/j.1748-1716.1978.tb06229.x. [DOI] [PubMed] [Google Scholar]
- Spring K. R., Kimura G. Chloride reabsorption by renal proximal tubules of Necturus. J Membr Biol. 1978 Jan 18;38(3):233–254. doi: 10.1007/BF01871924. [DOI] [PubMed] [Google Scholar]
- Turnberg L. A., Bieberdorf F. A., Morawski S. G., Fordtran J. S. Interrelationships of chloride, bicarbonate, sodium, and hydrogen transport in the human ileum. J Clin Invest. 1970 Mar;49(3):557–567. doi: 10.1172/JCI106266. [DOI] [PMC free article] [PubMed] [Google Scholar]
- Turner R. J., Silverman M. Sugar uptake into brush border vesicles from dog kidney. I. Specificity. Biochim Biophys Acta. 1978 Feb 21;507(2):305–321. doi: 10.1016/0005-2736(78)90425-x. [DOI] [PubMed] [Google Scholar]
- Turner R. J., Silverman M. Sugar uptake into brush border vesicles from dog kidney. II. Kinetics. Biochim Biophys Acta. 1978 Aug 17;511(3):470–486. doi: 10.1016/0005-2736(78)90282-1. [DOI] [PubMed] [Google Scholar]
- Ullrich K. J. Sugar, amino acid, and Na+ cotransport in the proximal tubule. Annu Rev Physiol. 1979;41:181–195. doi: 10.1146/annurev.ph.41.030179.001145. [DOI] [PubMed] [Google Scholar]
- Weiner M. W. Mitochondrial permeability to chloride ion. Am J Physiol. 1975 Jan;228(1):122–126. doi: 10.1152/ajplegacy.1975.228.1.122. [DOI] [PubMed] [Google Scholar]
- Windhager E. E., Giebisch G. Proximal sodium and fluid transport. Kidney Int. 1976 Feb;9(2):121–133. doi: 10.1038/ki.1976.16. [DOI] [PubMed] [Google Scholar]