Abstract
It should be possible to predict the rate of growth of Escherichia coli of a given genotype in a specified environment. The idea that the rate of synthesis of ATP determines the rate of growth and that the yield of ATP determines the yield of growth is entrenched in bacterial physiology, yet this idea is inconsistent with experimental results. In minimal media the growth rate and yield vary with the carbon source in a manner independent of the rate of formation and yield of ATP. With acetate as the carbon source, anapleurotic reactions, not ATP synthesis, limit the growth rate. For acetate and other gluconeogenic substrates the limiting step appears to be the formation of triose phosphate. I conclude that the rate of growth is controlled by the rate of formation of a precursor metabolite and, thus, of monomers such as amino acids derived from it. The protein-synthesizing system is regulated according to demand for protein synthesis. I examine the conjecture that the signal for this regulation is the ratio of uncharged tRNA to aminoacyl-tRNA, that this signal controls the concentration of guanosine tetraphosphate, and that the concentration of guanosine tetraphosphate controls transcription of rrn genes. Differential equations describing this system were solved numerically for steady states of growth; the computed values of ribosomes and guanosine tetraphosphate and the maximal growth rate agree with experimental values obtained from the literature of the past 35 years. These equations were also solved for dynamical states corresponding to nutritional shifts up and down.
Full text
PDF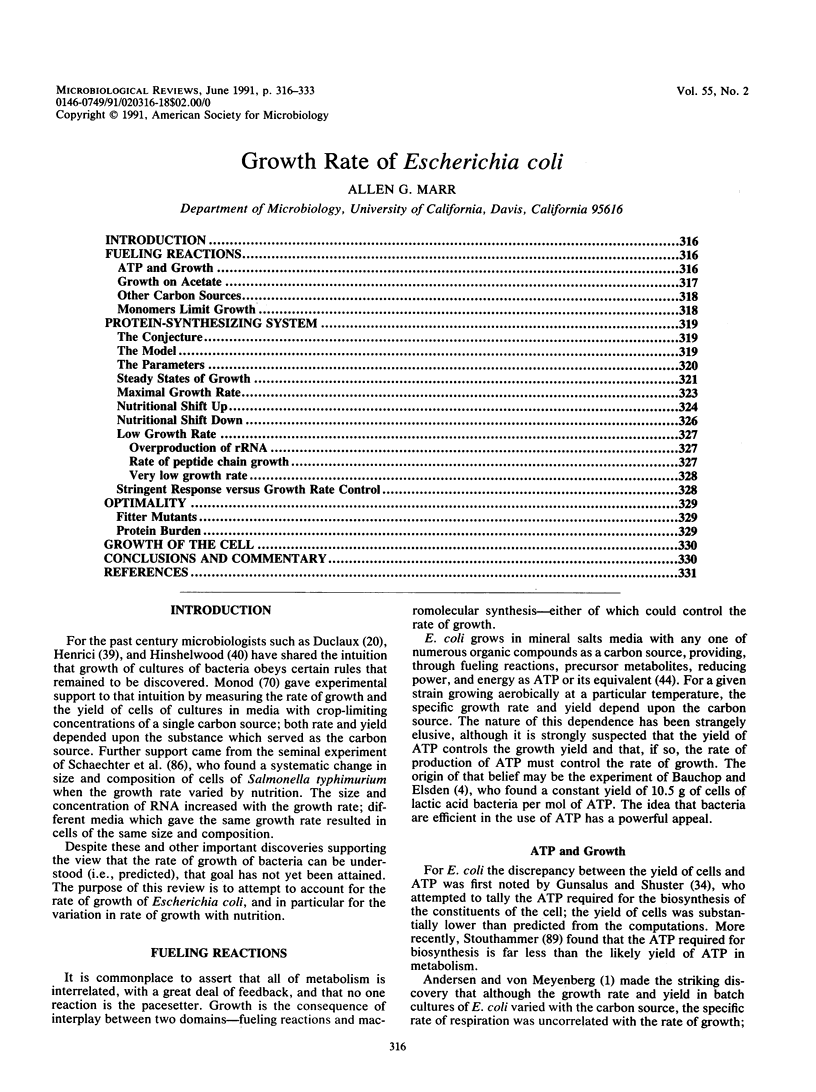
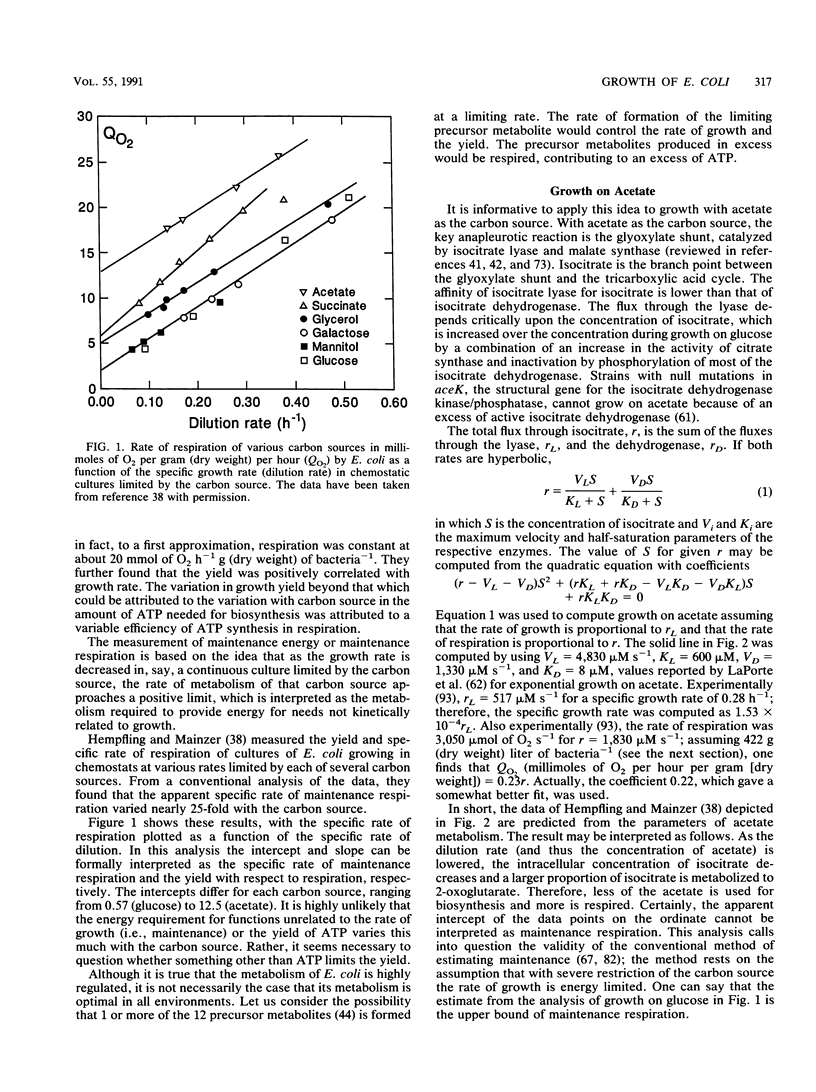
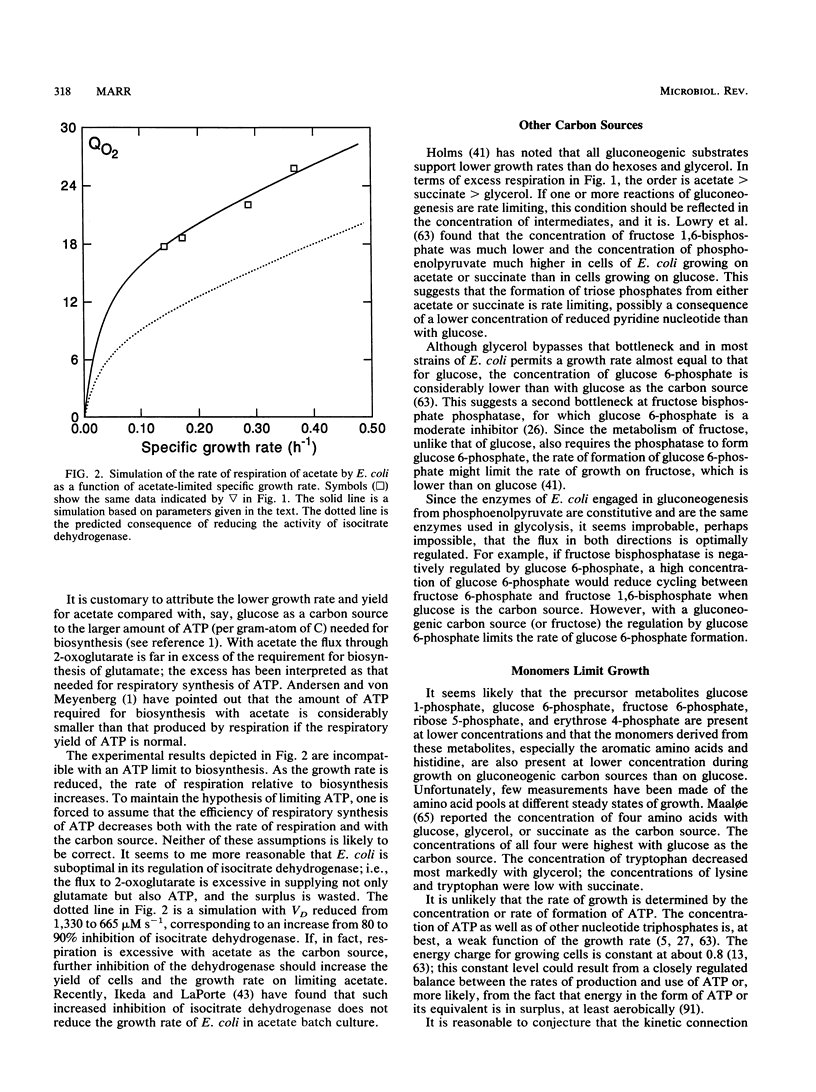
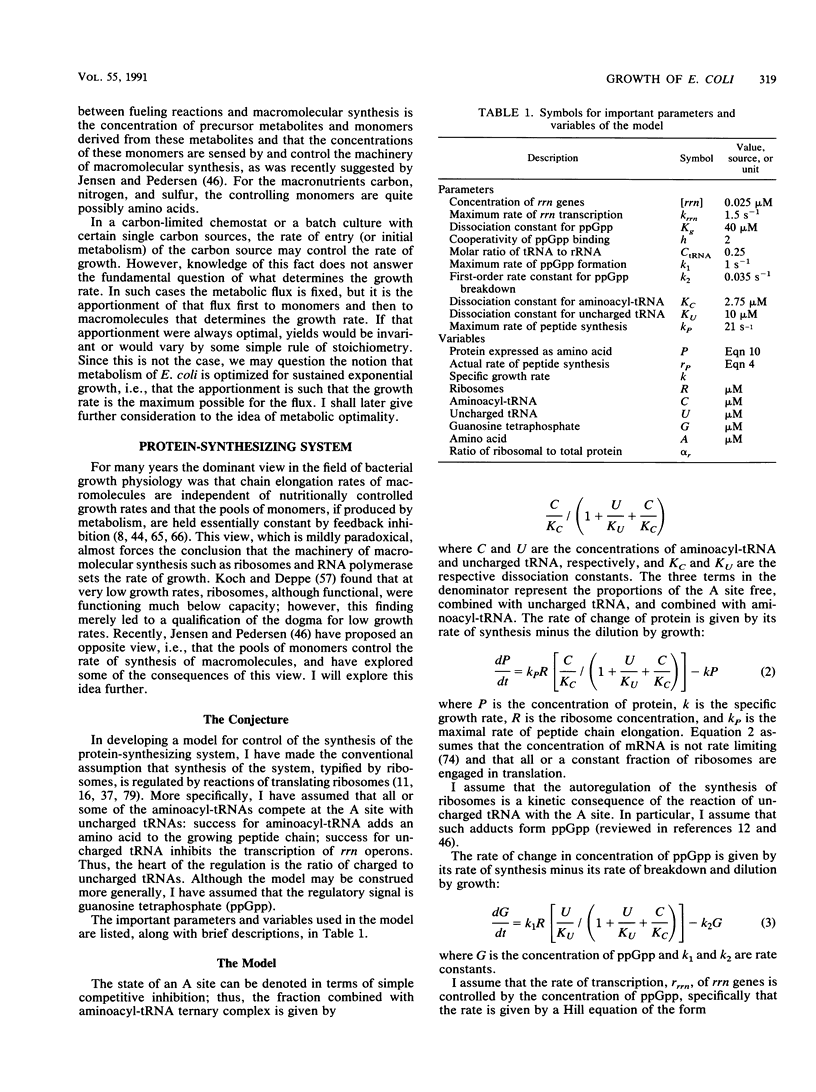
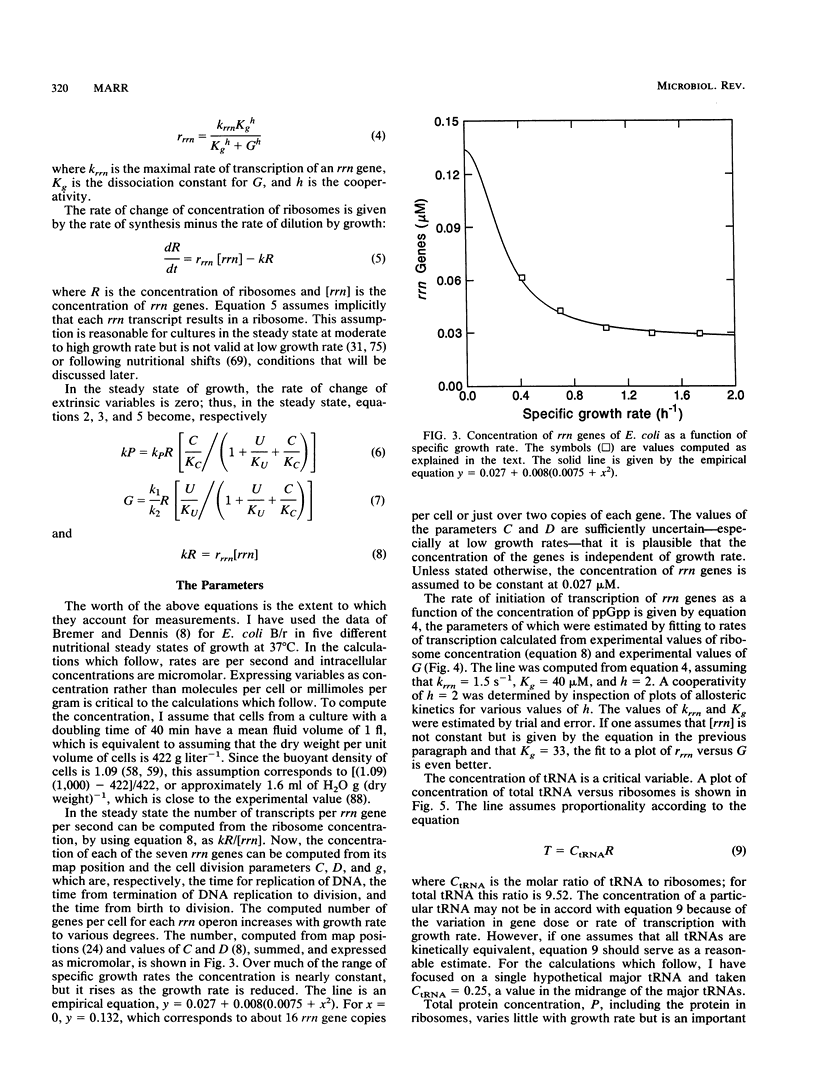
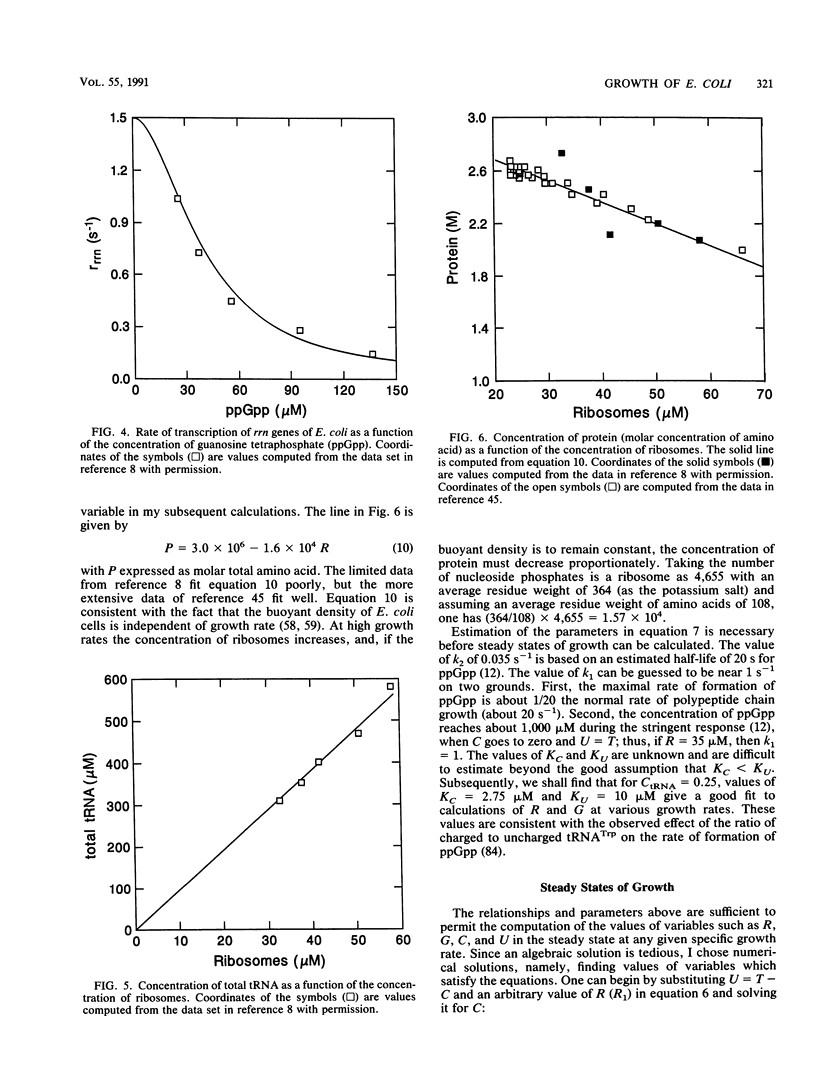
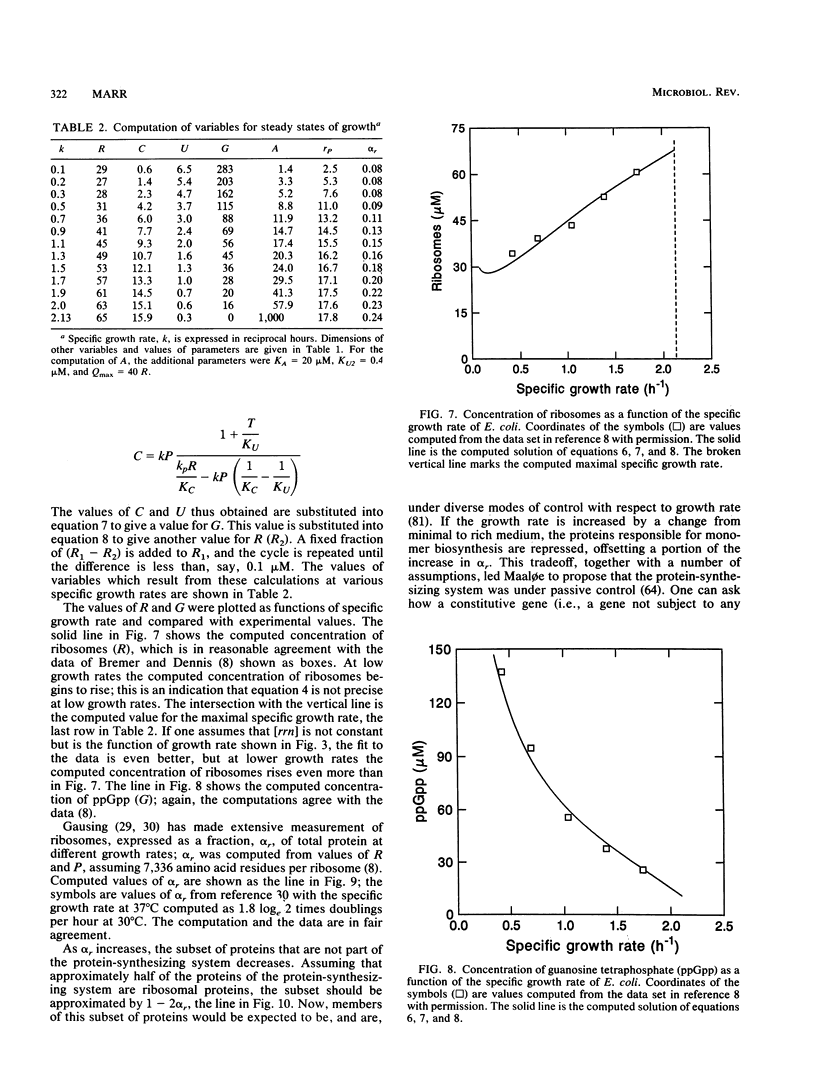
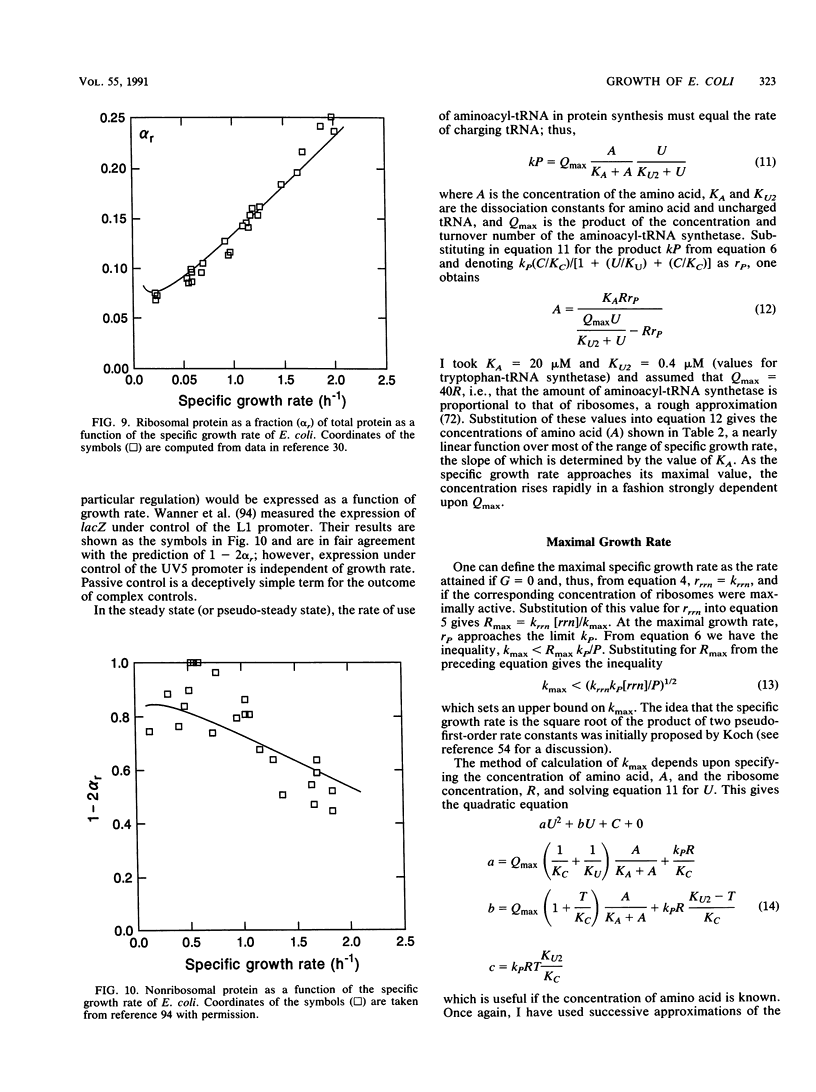
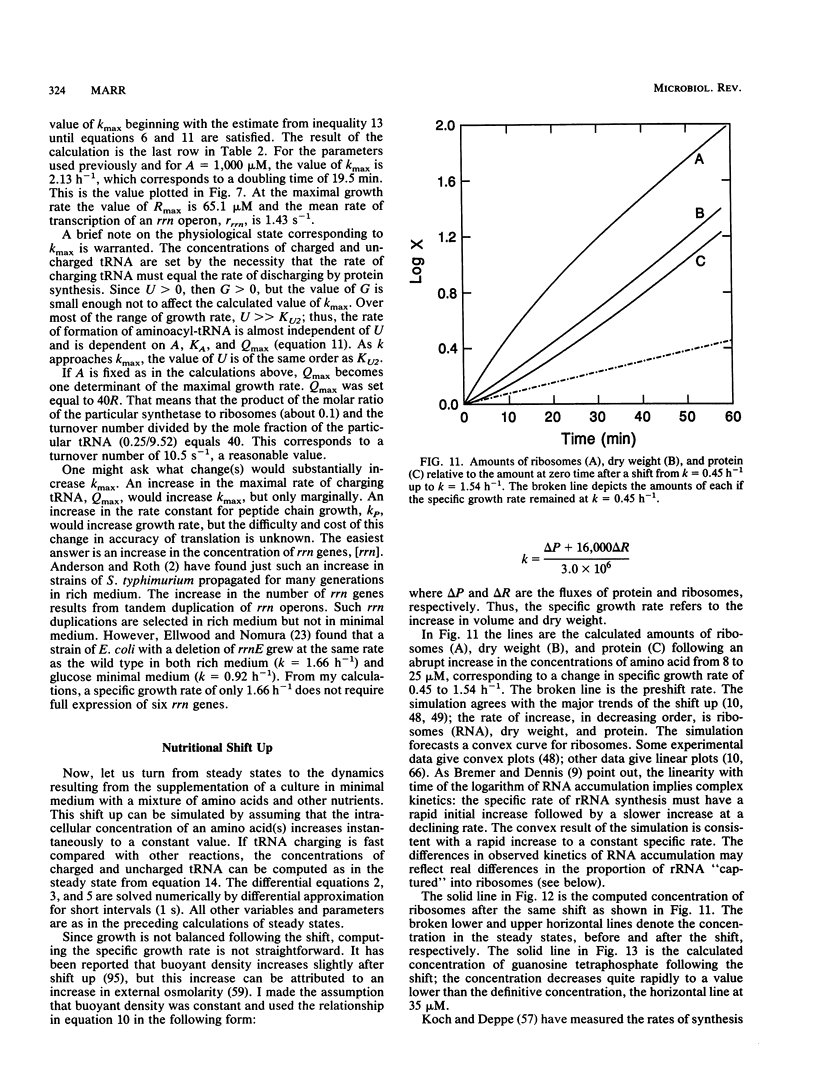
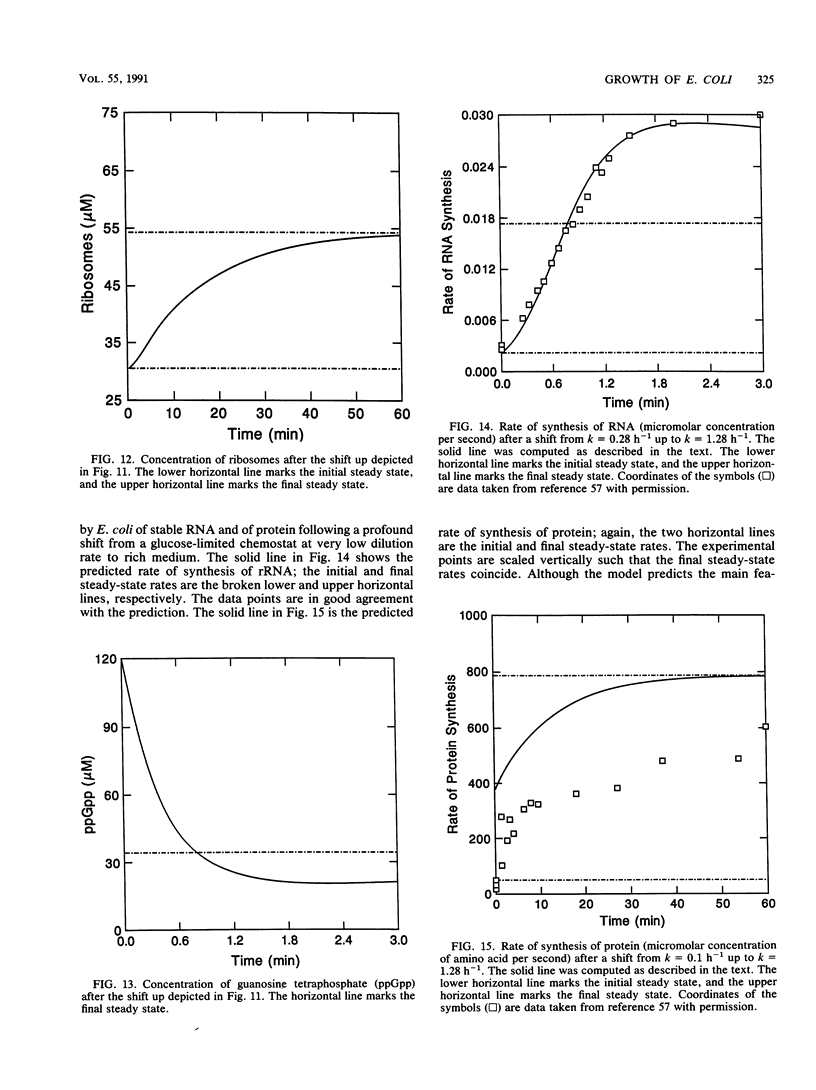
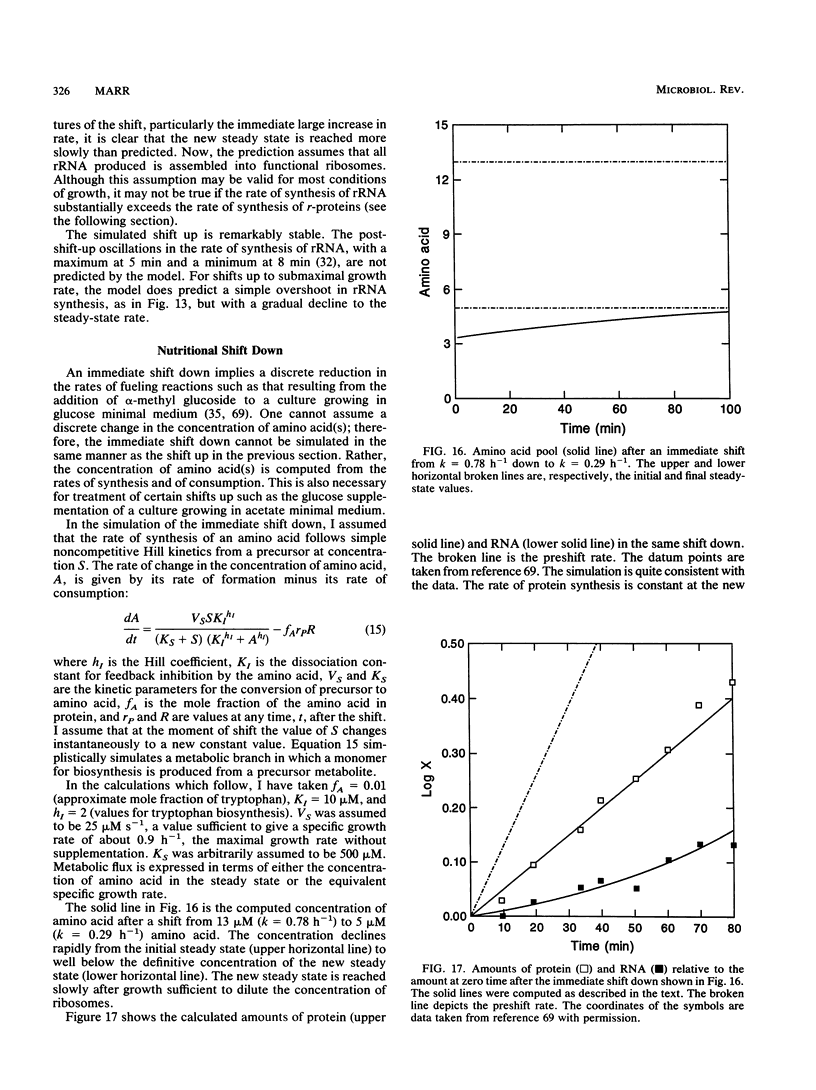
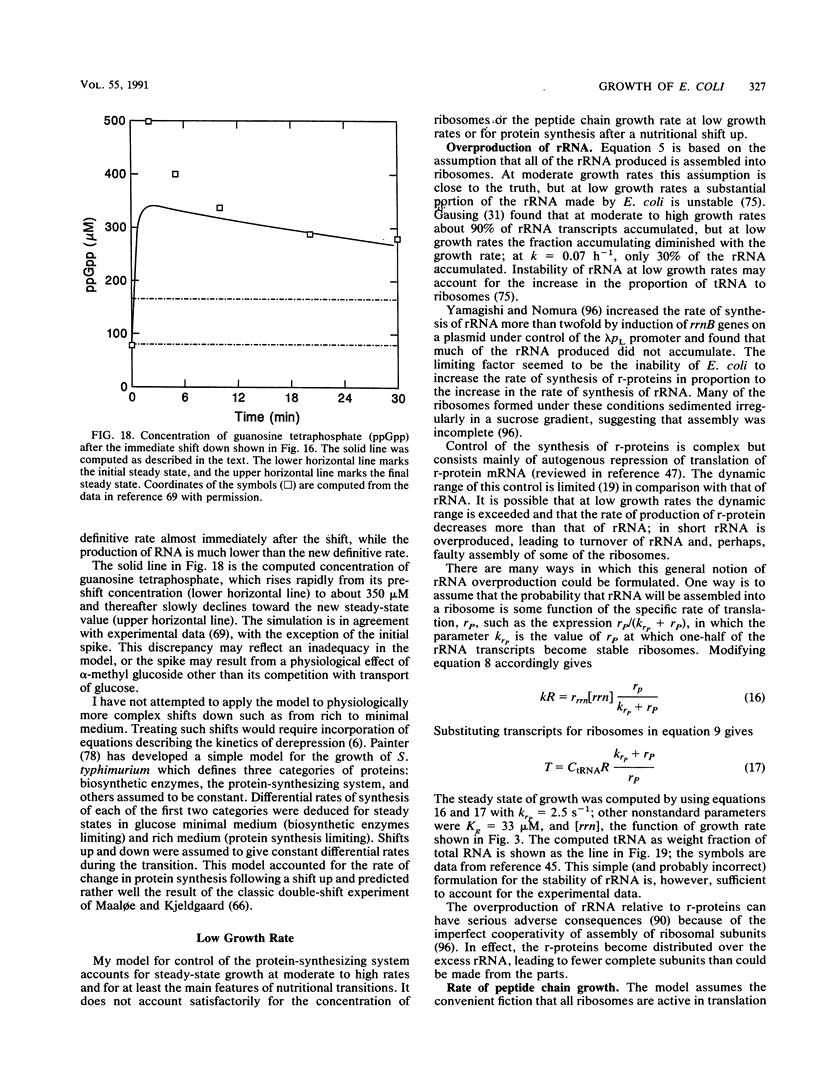
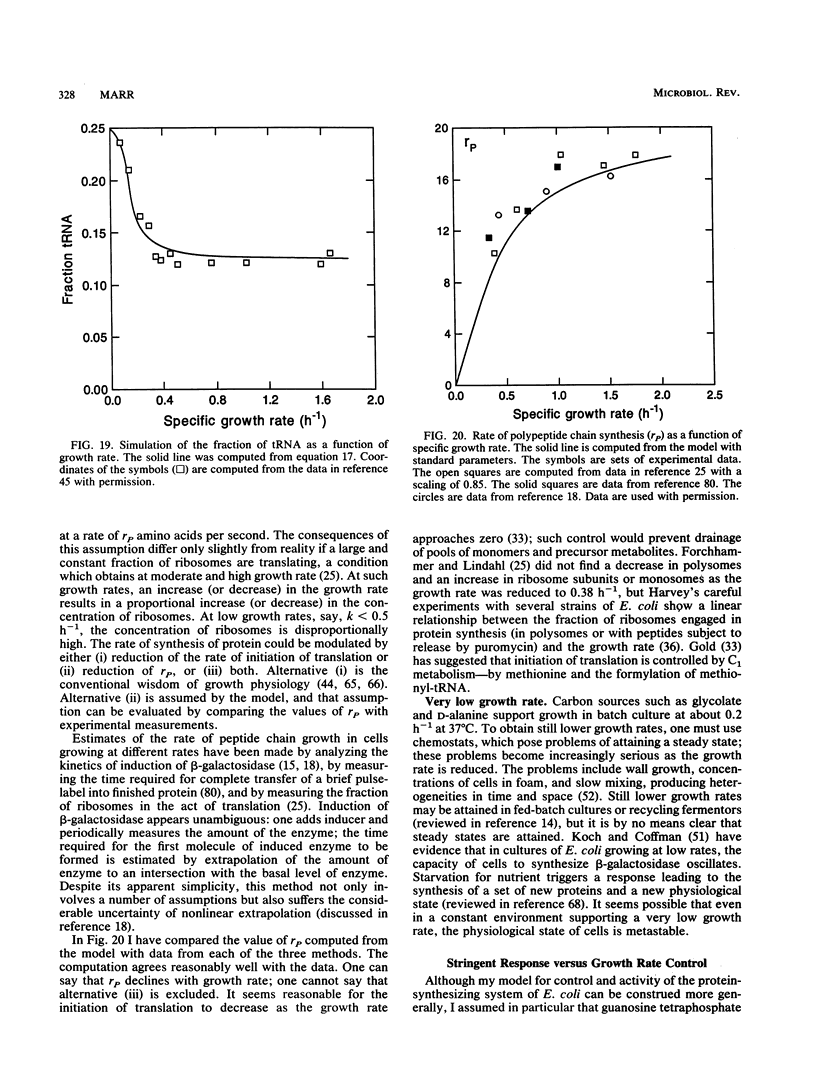
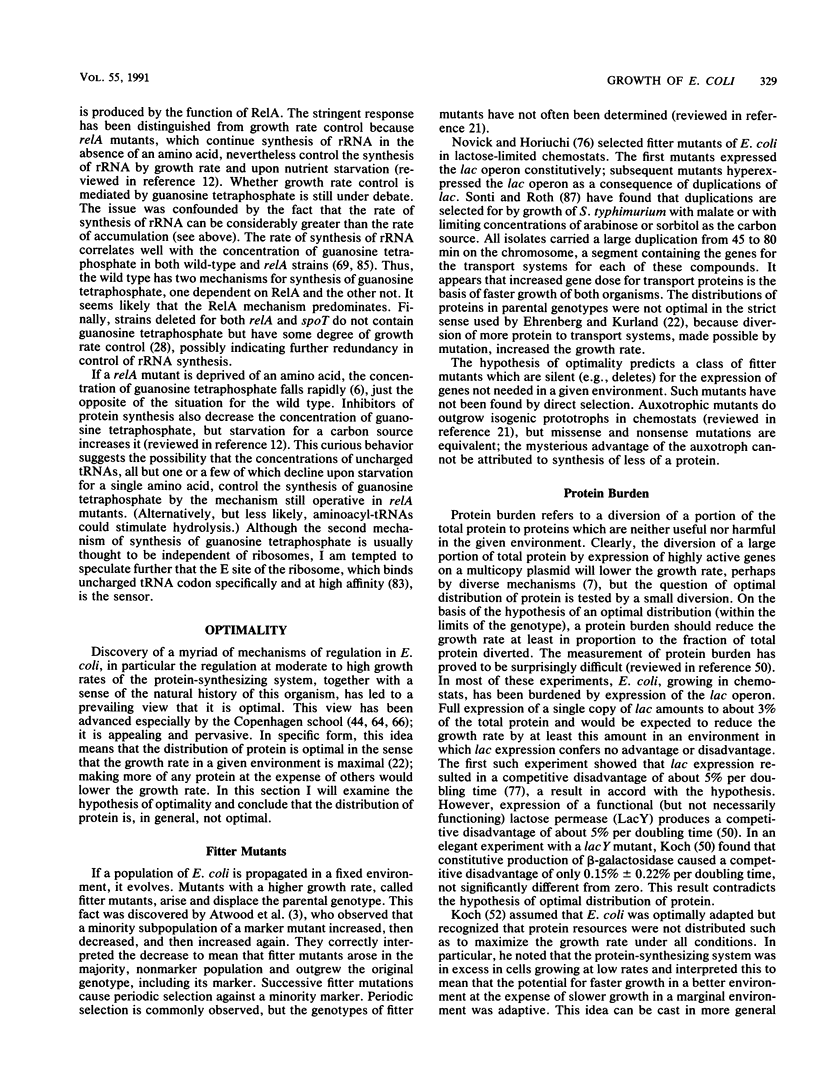
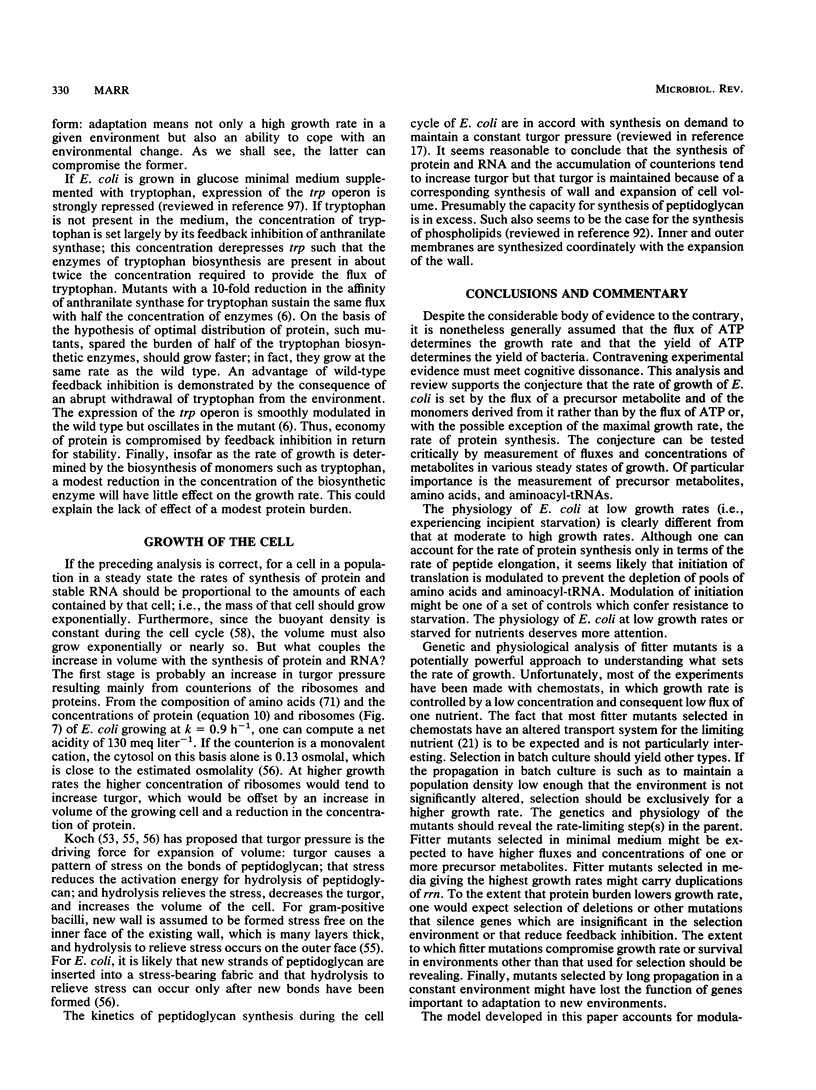
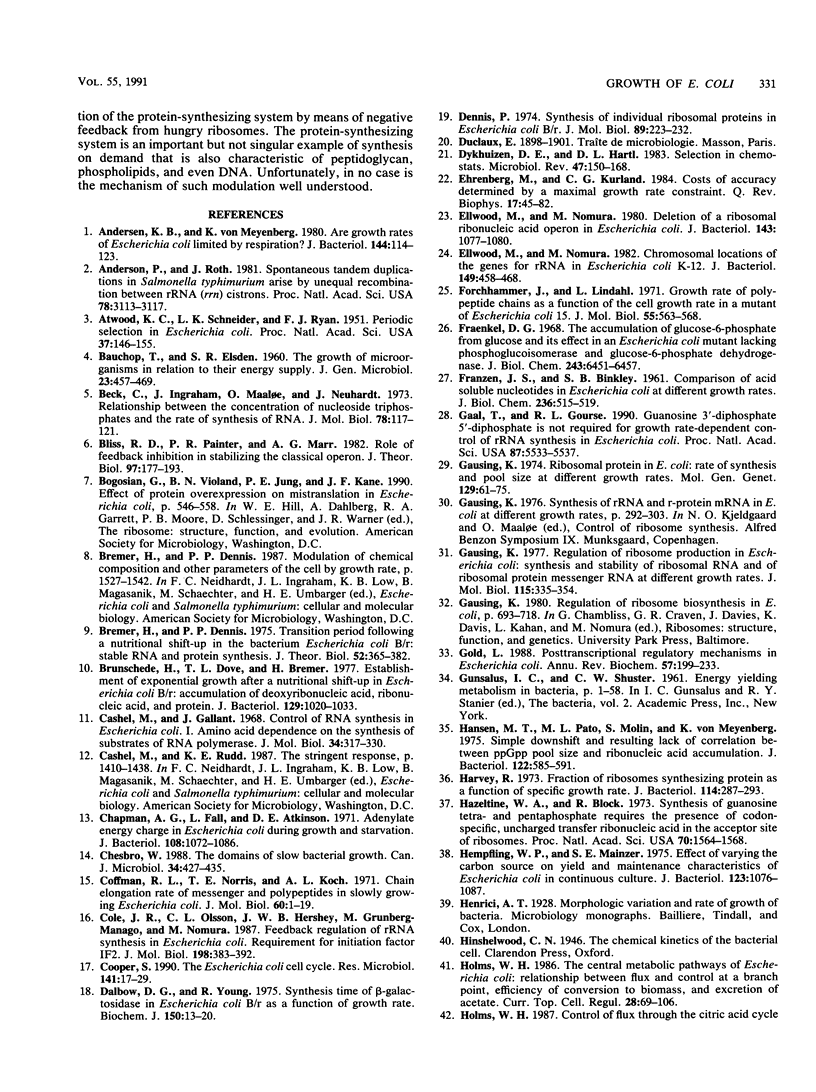
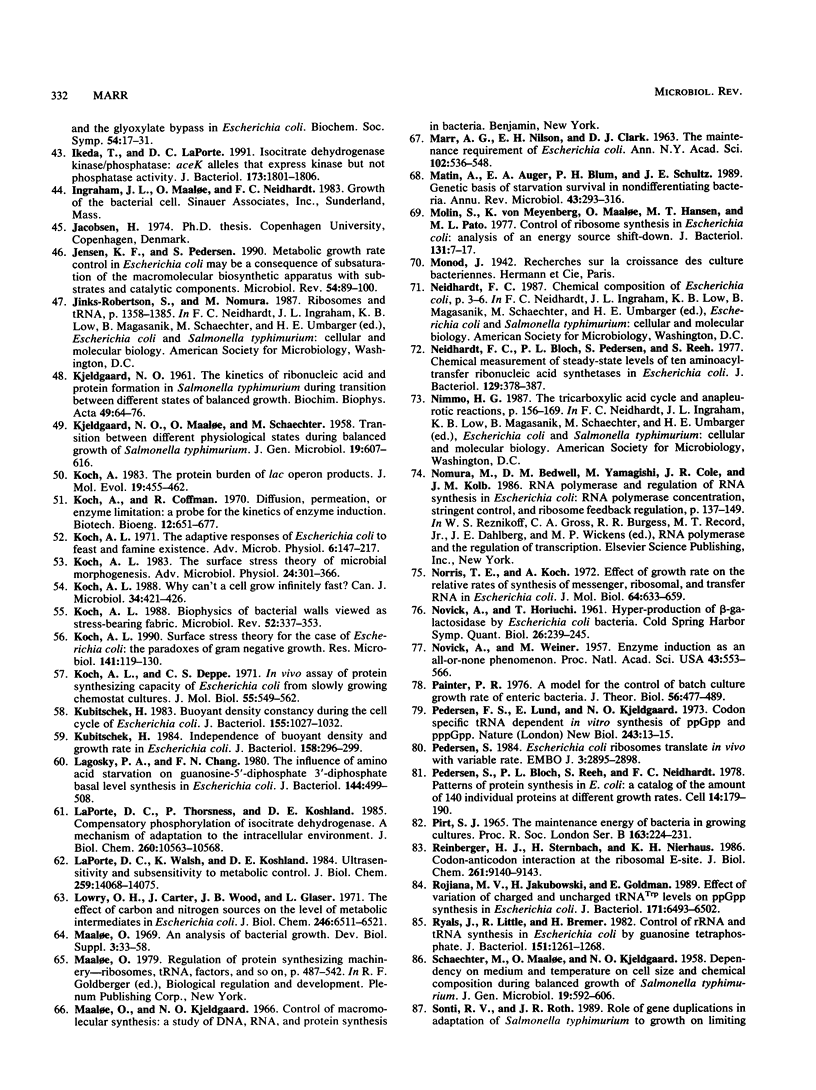
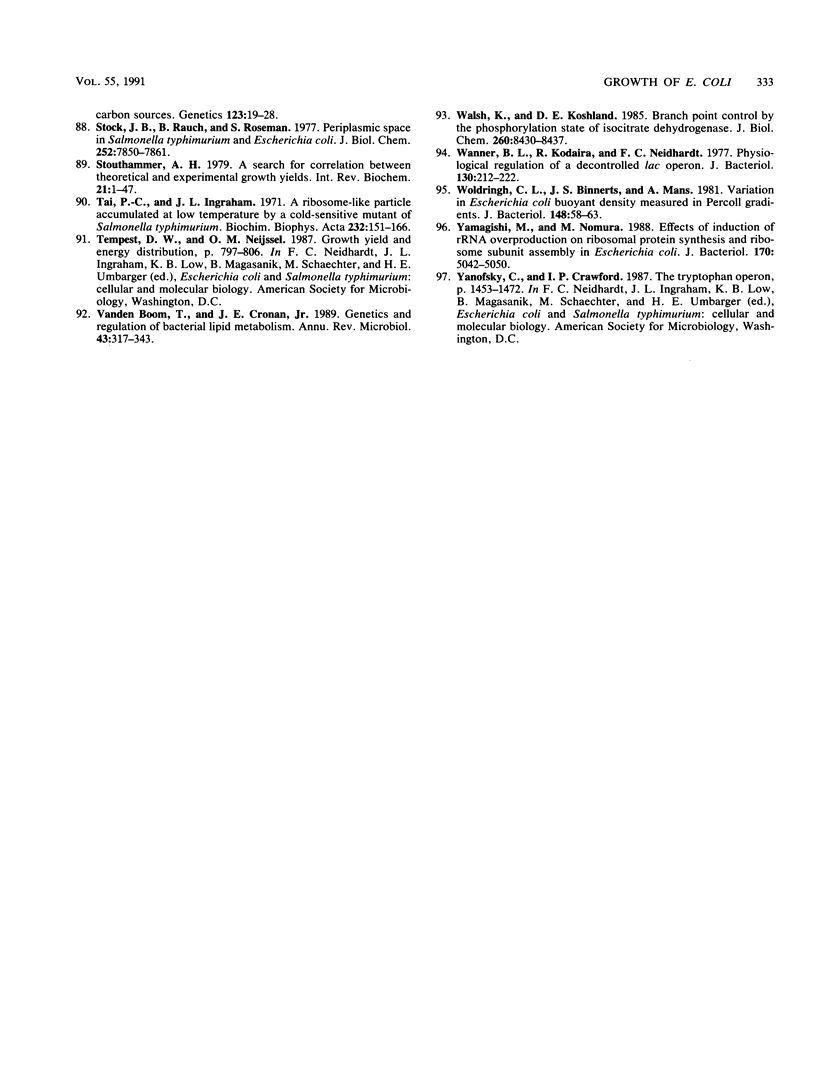
Selected References
These references are in PubMed. This may not be the complete list of references from this article.
- ATWOOD K. C., SCHNEIDER L. K., RYAN F. J. Periodic selection in Escherichia coli. Proc Natl Acad Sci U S A. 1951 Mar;37(3):146–155. doi: 10.1073/pnas.37.3.146. [DOI] [PMC free article] [PubMed] [Google Scholar]
- Andersen K. B., von Meyenburg K. Are growth rates of Escherichia coli in batch cultures limited by respiration? J Bacteriol. 1980 Oct;144(1):114–123. doi: 10.1128/jb.144.1.114-123.1980. [DOI] [PMC free article] [PubMed] [Google Scholar]
- Anderson P., Roth J. Spontaneous tandem genetic duplications in Salmonella typhimurium arise by unequal recombination between rRNA (rrn) cistrons. Proc Natl Acad Sci U S A. 1981 May;78(5):3113–3117. doi: 10.1073/pnas.78.5.3113. [DOI] [PMC free article] [PubMed] [Google Scholar]
- BAUCHOP T., ELSDEN S. R. The growth of micro-organisms in relation to their energy supply. J Gen Microbiol. 1960 Dec;23:457–469. doi: 10.1099/00221287-23-3-457. [DOI] [PubMed] [Google Scholar]
- Beck C., Ingraham J., Maaloe O., Neuhard J. Relationship between the concentration of nucleoside triphosphates and the rate of synthesis of RNA. J Mol Biol. 1973 Jun 25;78(1):117–121. doi: 10.1016/0022-2836(73)90431-2. [DOI] [PubMed] [Google Scholar]
- Bliss R. D., Painter P. R., Marr A. G. Role of feedback inhibition in stabilizing the classical operon. J Theor Biol. 1982 Jul 21;97(2):177–193. doi: 10.1016/0022-5193(82)90098-4. [DOI] [PubMed] [Google Scholar]
- Bremer H., Dennis P. P. Transition period following a nutritional shift-up in the bacterium Escherichia coli B/r: stable RNA and protein synthesis. J Theor Biol. 1975 Aug;52(2):365–382. doi: 10.1016/0022-5193(75)90007-7. [DOI] [PubMed] [Google Scholar]
- Brunschede H., Dove T. L., Bremer H. Establishment of exponential growth after a nutritional shift-up in Escherichia coli B/r: accumulation of deoxyribonucleic acid, ribonucleic acid, and protein. J Bacteriol. 1977 Feb;129(2):1020–1033. doi: 10.1128/jb.129.2.1020-1033.1977. [DOI] [PMC free article] [PubMed] [Google Scholar]
- Cashel M., Gallant J. Control of RNA synthesis in Escherichia coli. I. Amino acid dependence of the synthesis of the substrates of RNA polymerase. J Mol Biol. 1968 Jul 14;34(2):317–330. doi: 10.1016/0022-2836(68)90256-8. [DOI] [PubMed] [Google Scholar]
- Chapman A. G., Fall L., Atkinson D. E. Adenylate energy charge in Escherichia coli during growth and starvation. J Bacteriol. 1971 Dec;108(3):1072–1086. doi: 10.1128/jb.108.3.1072-1086.1971. [DOI] [PMC free article] [PubMed] [Google Scholar]
- Chesbro W. The domains of slow bacterial growth. Can J Microbiol. 1988 Apr;34(4):427–435. doi: 10.1139/m88-075. [DOI] [PubMed] [Google Scholar]
- Coffman R. L., Norris T. E., Koch A. L. Chain elongation rate of messenger and polypeptides in slowly growing Escherichia coli. J Mol Biol. 1971 Aug 28;60(1):1–19. doi: 10.1016/0022-2836(71)90442-6. [DOI] [PubMed] [Google Scholar]
- Cole J. R., Olsson C. L., Hershey J. W., Grunberg-Manago M., Nomura M. Feedback regulation of rRNA synthesis in Escherichia coli. Requirement for initiation factor IF2. J Mol Biol. 1987 Dec 5;198(3):383–392. doi: 10.1016/0022-2836(87)90288-9. [DOI] [PubMed] [Google Scholar]
- Cooper S. The Escherichia coli cell cycle. Res Microbiol. 1990 Jan;141(1):17–29. doi: 10.1016/0923-2508(90)90094-7. [DOI] [PubMed] [Google Scholar]
- Dalbow D. G., Young R. Synthesis time of beta-galactosidase in Escherichia coli B/r as a function of growth rate. Biochem J. 1975 Jul;150(1):13–20. doi: 10.1042/bj1500013. [DOI] [PMC free article] [PubMed] [Google Scholar]
- Dennis P. P. Synthesis of individual ribosomal proteins in Escherichia coli B/r. J Mol Biol. 1974 Oct 15;89(1):223–232. [PubMed] [Google Scholar]
- Dykhuizen D. E., Hartl D. L. Selection in chemostats. Microbiol Rev. 1983 Jun;47(2):150–168. doi: 10.1128/mr.47.2.150-168.1983. [DOI] [PMC free article] [PubMed] [Google Scholar]
- Ehrenberg M., Kurland C. G. Costs of accuracy determined by a maximal growth rate constraint. Q Rev Biophys. 1984 Feb;17(1):45–82. doi: 10.1017/s0033583500005254. [DOI] [PubMed] [Google Scholar]
- Ellwood M., Nomura M. Chromosomal locations of the genes for rRNA in Escherichia coli K-12. J Bacteriol. 1982 Feb;149(2):458–468. doi: 10.1128/jb.149.2.458-468.1982. [DOI] [PMC free article] [PubMed] [Google Scholar]
- Ellwood M., Nomura M. Deletion of a ribosomal ribonucleic acid operon in Escherichia coli. J Bacteriol. 1980 Aug;143(2):1077–1080. doi: 10.1128/jb.143.2.1077-1080.1980. [DOI] [PMC free article] [PubMed] [Google Scholar]
- FRANZEN J. S., BINKLEY S. B. Comparison of the acid-soluble nucleotides in Escherichia coli at different growth rates. J Biol Chem. 1961 Feb;236:515–519. [PubMed] [Google Scholar]
- Forchhammer J., Lindahl L. Growth rate of polypeptide chains as a function of the cell growth rate in a mutant of Escherichia coli 15. J Mol Biol. 1971 Feb 14;55(3):563–568. doi: 10.1016/0022-2836(71)90337-8. [DOI] [PubMed] [Google Scholar]
- Fraenkel D. G. The accumulation of glucose 6-phosphate from glucose and its effect in an Escherichia coli mutant lacking phosphoglucose isomerase and glucose 6-phosphate dehydrogenase. J Biol Chem. 1968 Dec 25;243(24):6451–6457. [PubMed] [Google Scholar]
- Gaal T., Gourse R. L. Guanosine 3'-diphosphate 5'-diphosphate is not required for growth rate-dependent control of rRNA synthesis in Escherichia coli. Proc Natl Acad Sci U S A. 1990 Jul;87(14):5533–5537. doi: 10.1073/pnas.87.14.5533. [DOI] [PMC free article] [PubMed] [Google Scholar]
- Gausing K. Regulation of ribosome production in Escherichia coli: synthesis and stability of ribosomal RNA and of ribosomal protein messenger RNA at different growth rates. J Mol Biol. 1977 Sep 25;115(3):335–354. doi: 10.1016/0022-2836(77)90158-9. [DOI] [PubMed] [Google Scholar]
- Gausing K. Ribosomal protein in E. coli: rate of synthesis and pool size at different growth rates. Mol Gen Genet. 1974 Mar 6;129(1):61–75. doi: 10.1007/BF00269266. [DOI] [PubMed] [Google Scholar]
- Gold L. Posttranscriptional regulatory mechanisms in Escherichia coli. Annu Rev Biochem. 1988;57:199–233. doi: 10.1146/annurev.bi.57.070188.001215. [DOI] [PubMed] [Google Scholar]
- Hansen M. T., Pato M. L., Molin S., Fill N. P., von Meyenburg K. Simple downshift and resulting lack of correlation between ppGpp pool size and ribonucleic acid accumulation. J Bacteriol. 1975 May;122(2):585–591. doi: 10.1128/jb.122.2.585-591.1975. [DOI] [PMC free article] [PubMed] [Google Scholar]
- Harvey R. J. Fraction of ribosomes synthesizing protein as a function of specific growth rate. J Bacteriol. 1973 Apr;114(1):287–293. doi: 10.1128/jb.114.1.287-293.1973. [DOI] [PMC free article] [PubMed] [Google Scholar]
- Haseltine W. A., Block R. Synthesis of guanosine tetra- and pentaphosphate requires the presence of a codon-specific, uncharged transfer ribonucleic acid in the acceptor site of ribosomes. Proc Natl Acad Sci U S A. 1973 May;70(5):1564–1568. doi: 10.1073/pnas.70.5.1564. [DOI] [PMC free article] [PubMed] [Google Scholar]
- Hempfling W. P., Mainzer S. E. Effects of varying the carbon source limiting growth on yield and maintenance characteristics of Escherichia coli in continuous culture. J Bacteriol. 1975 Sep;123(3):1076–1087. doi: 10.1128/jb.123.3.1076-1087.1975. [DOI] [PMC free article] [PubMed] [Google Scholar]
- Holms W. H. Control of flux through the citric acid cycle and the glyoxylate bypass in Escherichia coli. Biochem Soc Symp. 1987;54:17–31. [PubMed] [Google Scholar]
- Holms W. H. The central metabolic pathways of Escherichia coli: relationship between flux and control at a branch point, efficiency of conversion to biomass, and excretion of acetate. Curr Top Cell Regul. 1986;28:69–105. doi: 10.1016/b978-0-12-152828-7.50004-4. [DOI] [PubMed] [Google Scholar]
- Ikeda T., LaPorte D. C. Isocitrate dehydrogenase kinase/phosphatase: aceK alleles that express kinase but not phosphatase activity. J Bacteriol. 1991 Mar;173(5):1801–1806. doi: 10.1128/jb.173.5.1801-1806.1991. [DOI] [PMC free article] [PubMed] [Google Scholar]
- Jensen K. F., Pedersen S. Metabolic growth rate control in Escherichia coli may be a consequence of subsaturation of the macromolecular biosynthetic apparatus with substrates and catalytic components. Microbiol Rev. 1990 Jun;54(2):89–100. doi: 10.1128/mr.54.2.89-100.1990. [DOI] [PMC free article] [PubMed] [Google Scholar]
- KJELDGAARD N. O., MAALOE O., SCHAECHTER M. The transition between different physiological states during balanced growth of Salmonella typhimurium. J Gen Microbiol. 1958 Dec;19(3):607–616. doi: 10.1099/00221287-19-3-607. [DOI] [PubMed] [Google Scholar]
- KJELDGAARD N. O. The kinetics of ribonucleic acid- and protein formation in Salmonella typhimurium during the transition between different states of balance growth. Biochim Biophys Acta. 1961 Apr 29;49:64–76. doi: 10.1016/0006-3002(61)90870-8. [DOI] [PubMed] [Google Scholar]
- Koch A. L. Biophysics of bacterial walls viewed as stress-bearing fabric. Microbiol Rev. 1988 Sep;52(3):337–353. doi: 10.1128/mr.52.3.337-353.1988. [DOI] [PMC free article] [PubMed] [Google Scholar]
- Koch A. L., Coffman R. Diffusion, permeation, or enzyme limitation: a probe for the kinetics of enzyme induction. Biotechnol Bioeng. 1970 Sep;12(5):651–677. doi: 10.1002/bit.260120503. [DOI] [PubMed] [Google Scholar]
- Koch A. L., Deppe C. S. In vivo assay of protein synthesizing capacity of Escherichia coli from slowly growing chemostat cultures. J Mol Biol. 1971 Feb 14;55(3):549–562. doi: 10.1016/0022-2836(71)90336-6. [DOI] [PubMed] [Google Scholar]
- Koch A. L. The adaptive responses of Escherichia coli to a feast and famine existence. Adv Microb Physiol. 1971;6:147–217. doi: 10.1016/s0065-2911(08)60069-7. [DOI] [PubMed] [Google Scholar]
- Koch A. L. The protein burden of lac operon products. J Mol Evol. 1983;19(6):455–462. doi: 10.1007/BF02102321. [DOI] [PubMed] [Google Scholar]
- Koch A. L. The surface stress theory for the case of Escherichia coli: the paradoxes of gram-negative growth. Res Microbiol. 1990 Jan;141(1):119–130. doi: 10.1016/0923-2508(90)90103-w. [DOI] [PubMed] [Google Scholar]
- Koch A. L. The surface stress theory of microbial morphogenesis. Adv Microb Physiol. 1983;24:301–366. doi: 10.1016/s0065-2911(08)60388-4. [DOI] [PubMed] [Google Scholar]
- Koch A. L. Why can't a cell grow infinitely fast? Can J Microbiol. 1988 Apr;34(4):421–426. doi: 10.1139/m88-074. [DOI] [PubMed] [Google Scholar]
- Kubitschek H. E., Baldwin W. W., Graetzer R. Buoyant density constancy during the cell cycle of Escherichia coli. J Bacteriol. 1983 Sep;155(3):1027–1032. doi: 10.1128/jb.155.3.1027-1032.1983. [DOI] [PMC free article] [PubMed] [Google Scholar]
- Kubitschek H. E., Baldwin W. W., Schroeter S. J., Graetzer R. Independence of buoyant cell density and growth rate in Escherichia coli. J Bacteriol. 1984 Apr;158(1):296–299. doi: 10.1128/jb.158.1.296-299.1984. [DOI] [PMC free article] [PubMed] [Google Scholar]
- LaPorte D. C., Thorsness P. E., Koshland D. E., Jr Compensatory phosphorylation of isocitrate dehydrogenase. A mechanism for adaptation to the intracellular environment. J Biol Chem. 1985 Sep 5;260(19):10563–10568. [PubMed] [Google Scholar]
- LaPorte D. C., Walsh K., Koshland D. E., Jr The branch point effect. Ultrasensitivity and subsensitivity to metabolic control. J Biol Chem. 1984 Nov 25;259(22):14068–14075. [PubMed] [Google Scholar]
- Lagosky P. A., Chang F. N. Influence of amino acid starvation on guanosine 5'-diphosphate 3'-diphosphate basal-level synthesis in Escherichia coli. J Bacteriol. 1980 Nov;144(2):499–508. doi: 10.1128/jb.144.2.499-508.1980. [DOI] [PMC free article] [PubMed] [Google Scholar]
- Lowry O. H., Carter J., Ward J. B., Glaser L. The effect of carbon and nitrogen sources on the level of metabolic intermediates in Escherichia coli. J Biol Chem. 1971 Nov;246(21):6511–6521. [PubMed] [Google Scholar]
- Matin A., Auger E. A., Blum P. H., Schultz J. E. Genetic basis of starvation survival in nondifferentiating bacteria. Annu Rev Microbiol. 1989;43:293–316. doi: 10.1146/annurev.mi.43.100189.001453. [DOI] [PubMed] [Google Scholar]
- Molin S., Von Meyenburg K., Maaloe O., Hansen M. T., Pato M. L. Control of ribosome synthesis in Escherichia coli: analysis of an energy source shift-down. J Bacteriol. 1977 Jul;131(1):7–17. doi: 10.1128/jb.131.1.7-17.1977. [DOI] [PMC free article] [PubMed] [Google Scholar]
- NOVICK A., HORIUCHI T. Hyper-production of beta-galactosidase by Escherichia coli bacteria. Cold Spring Harb Symp Quant Biol. 1961;26:239–245. doi: 10.1101/sqb.1961.026.01.029. [DOI] [PubMed] [Google Scholar]
- Neidhardt F. C., Bloch P. L., Pedersen S., Reeh S. Chemical measurement of steady-state levels of ten aminoacyl-transfer ribonucleic acid synthetases in Escherichia coli. J Bacteriol. 1977 Jan;129(1):378–387. doi: 10.1128/jb.129.1.378-387.1977. [DOI] [PMC free article] [PubMed] [Google Scholar]
- Norris T. E., Koch A. L. Effect of growth rate on the relative rates of synthesis of messenger, ribosomal and transfer RNA in Escherichia coli. J Mol Biol. 1972 Mar 14;64(3):633–649. doi: 10.1016/0022-2836(72)90088-5. [DOI] [PubMed] [Google Scholar]
- Novick A., Weiner M. ENZYME INDUCTION AS AN ALL-OR-NONE PHENOMENON. Proc Natl Acad Sci U S A. 1957 Jul 15;43(7):553–566. doi: 10.1073/pnas.43.7.553. [DOI] [PMC free article] [PubMed] [Google Scholar]
- Painter P. R. A model for the control of the batch culture growth rate of enteric bacteria. J Theor Biol. 1976 Feb;56(2):477–489. doi: 10.1016/s0022-5193(76)80087-2. [DOI] [PubMed] [Google Scholar]
- Pedersen F. S., Lund E., Kjeldgaard N. O. Codon specific, tRNA dependent in vitro synthesis of ppGpp and pppGpp. Nat New Biol. 1973 May 2;243(122):13–15. [PubMed] [Google Scholar]
- Pedersen S., Bloch P. L., Reeh S., Neidhardt F. C. Patterns of protein synthesis in E. coli: a catalog of the amount of 140 individual proteins at different growth rates. Cell. 1978 May;14(1):179–190. doi: 10.1016/0092-8674(78)90312-4. [DOI] [PubMed] [Google Scholar]
- Pedersen S. Escherichia coli ribosomes translate in vivo with variable rate. EMBO J. 1984 Dec 1;3(12):2895–2898. doi: 10.1002/j.1460-2075.1984.tb02227.x. [DOI] [PMC free article] [PubMed] [Google Scholar]
- Pirt S. J. The maintenance energy of bacteria in growing cultures. Proc R Soc Lond B Biol Sci. 1965 Oct 12;163(991):224–231. doi: 10.1098/rspb.1965.0069. [DOI] [PubMed] [Google Scholar]
- Rheinberger H. J., Sternbach H., Nierhaus K. H. Codon-anticodon interaction at the ribosomal E site. J Biol Chem. 1986 Jul 15;261(20):9140–9143. [PubMed] [Google Scholar]
- Rojiani M. V., Jakubowski H., Goldman E. Effect of variation of charged and uncharged tRNA(Trp) levels on ppGpp synthesis in Escherichia coli. J Bacteriol. 1989 Dec;171(12):6493–6502. doi: 10.1128/jb.171.12.6493-6502.1989. [DOI] [PMC free article] [PubMed] [Google Scholar]
- Ryals J., Little R., Bremer H. Control of rRNA and tRNA syntheses in Escherichia coli by guanosine tetraphosphate. J Bacteriol. 1982 Sep;151(3):1261–1268. doi: 10.1128/jb.151.3.1261-1268.1982. [DOI] [PMC free article] [PubMed] [Google Scholar]
- SCHAECHTER M., MAALOE O., KJELDGAARD N. O. Dependency on medium and temperature of cell size and chemical composition during balanced grown of Salmonella typhimurium. J Gen Microbiol. 1958 Dec;19(3):592–606. doi: 10.1099/00221287-19-3-592. [DOI] [PubMed] [Google Scholar]
- Sonti R. V., Roth J. R. Role of gene duplications in the adaptation of Salmonella typhimurium to growth on limiting carbon sources. Genetics. 1989 Sep;123(1):19–28. doi: 10.1093/genetics/123.1.19. [DOI] [PMC free article] [PubMed] [Google Scholar]
- Stock J. B., Rauch B., Roseman S. Periplasmic space in Salmonella typhimurium and Escherichia coli. J Biol Chem. 1977 Nov 10;252(21):7850–7861. [PubMed] [Google Scholar]
- Tai P. C., Ingraham J. L. A ribosome-like particle accumulated at low temperature by a cold-sensitive mutant of Salmonella typhimurium. Biochim Biophys Acta. 1971 Feb 25;232(1):151–166. doi: 10.1016/0005-2787(71)90499-0. [DOI] [PubMed] [Google Scholar]
- Vanden Boom T., Cronan J. E., Jr Genetics and regulation of bacterial lipid metabolism. Annu Rev Microbiol. 1989;43:317–343. doi: 10.1146/annurev.mi.43.100189.001533. [DOI] [PubMed] [Google Scholar]
- Walsh K., Koshland D. E., Jr Branch point control by the phosphorylation state of isocitrate dehydrogenase. A quantitative examination of fluxes during a regulatory transition. J Biol Chem. 1985 Jul 15;260(14):8430–8437. [PubMed] [Google Scholar]
- Wanner B. L., Kodaira R., Neidhardt F. C. Physiological regulation of a decontrolled lac operon. J Bacteriol. 1977 Apr;130(1):212–222. doi: 10.1128/jb.130.1.212-222.1977. [DOI] [PMC free article] [PubMed] [Google Scholar]
- Woldringh C. L., Binnerts J. S., Mans A. Variation in Escherichia coli buoyant density measured in Percoll gradients. J Bacteriol. 1981 Oct;148(1):58–63. doi: 10.1128/jb.148.1.58-63.1981. [DOI] [PMC free article] [PubMed] [Google Scholar]
- Yamagishi M., Nomura M. Effects of induction of rRNA overproduction on ribosomal protein synthesis and ribosome subunit assembly in Escherichia coli. J Bacteriol. 1988 Nov;170(11):5042–5050. doi: 10.1128/jb.170.11.5042-5050.1988. [DOI] [PMC free article] [PubMed] [Google Scholar]