Abstract
Numerous gram-negative and gram-positive bacteria take up carbohydrates through the phosphoenolpyruvate (PEP):carbohydrate phosphotransferase system (PTS). This system transports and phosphorylates carbohydrates at the expense of PEP and is the subject of this review. The PTS consists of two general proteins, enzyme I and HPr, and a number of carbohydrate-specific enzymes, the enzymes II. PTS proteins are phosphoproteins in which the phospho group is attached to either a histidine residue or, in a number of cases, a cysteine residue. After phosphorylation of enzyme I by PEP, the phospho group is transferred to HPr. The enzymes II are required for the transport of the carbohydrates across the membrane and the transfer of the phospho group from phospho-HPr to the carbohydrates. Biochemical, structural, and molecular genetic studies have shown that the various enzymes II have the same basic structure. Each enzyme II consists of domains for specific functions, e.g., binding of the carbohydrate or phosphorylation. Each enzyme II complex can consist of one to four different polypeptides. The enzymes II can be placed into at least four classes on the basis of sequence similarity. The genetics of the PTS is complex, and the expression of PTS proteins is intricately regulated because of the central roles of these proteins in nutrient acquisition. In addition to classical induction-repression mechanisms involving repressor and activator proteins, other types of regulation, such as antitermination, have been observed in some PTSs. Apart from their role in carbohydrate transport, PTS proteins are involved in chemotaxis toward PTS carbohydrates. Furthermore, the IIAGlc protein, part of the glucose-specific PTS, is a central regulatory protein which in its nonphosphorylated form can bind to and inhibit several non-PTS uptake systems and thus prevent entry of inducers. In its phosphorylated form, P-IIAGlc is involved in the activation of adenylate cyclase and thus in the regulation of gene expression. By sensing the presence of PTS carbohydrates in the medium and adjusting the phosphorylation state of IIAGlc, cells can adapt quickly to changing conditions in the environment. In gram-positive bacteria, it has been demonstrated that HPr can be phosphorylated by ATP on a serine residue and this modification may perform a regulatory function.
Full text
PDF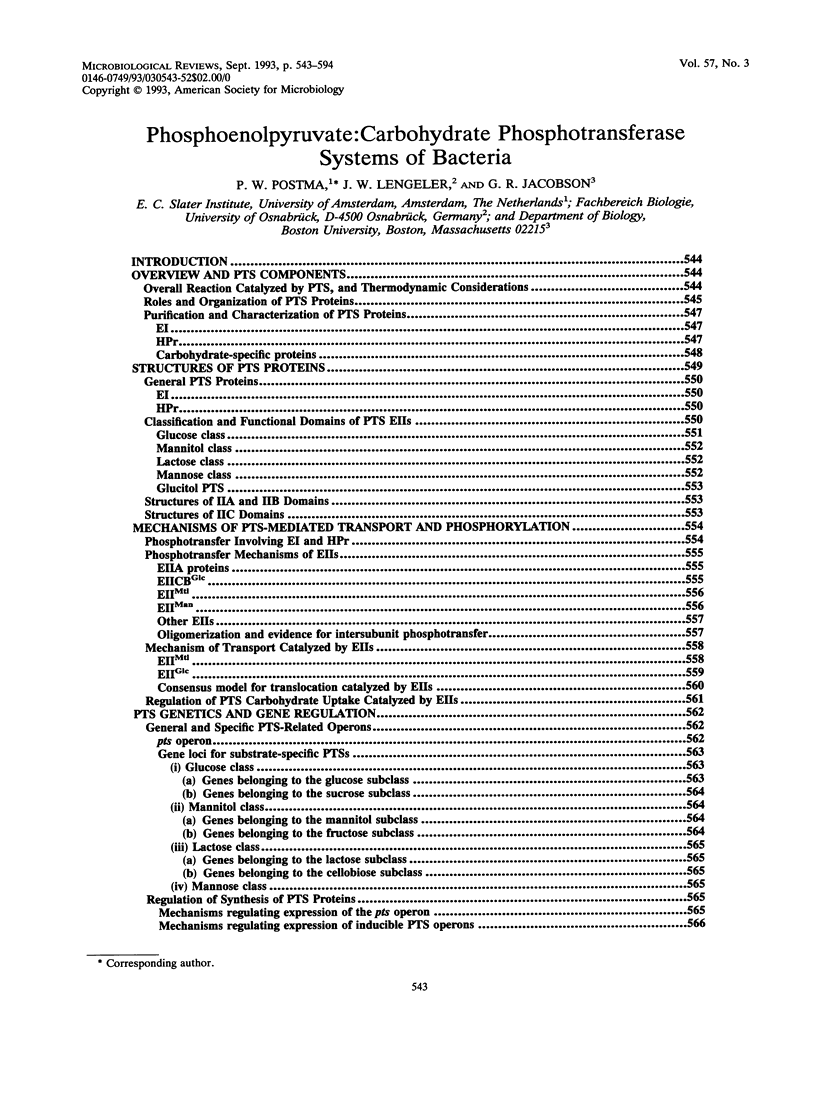
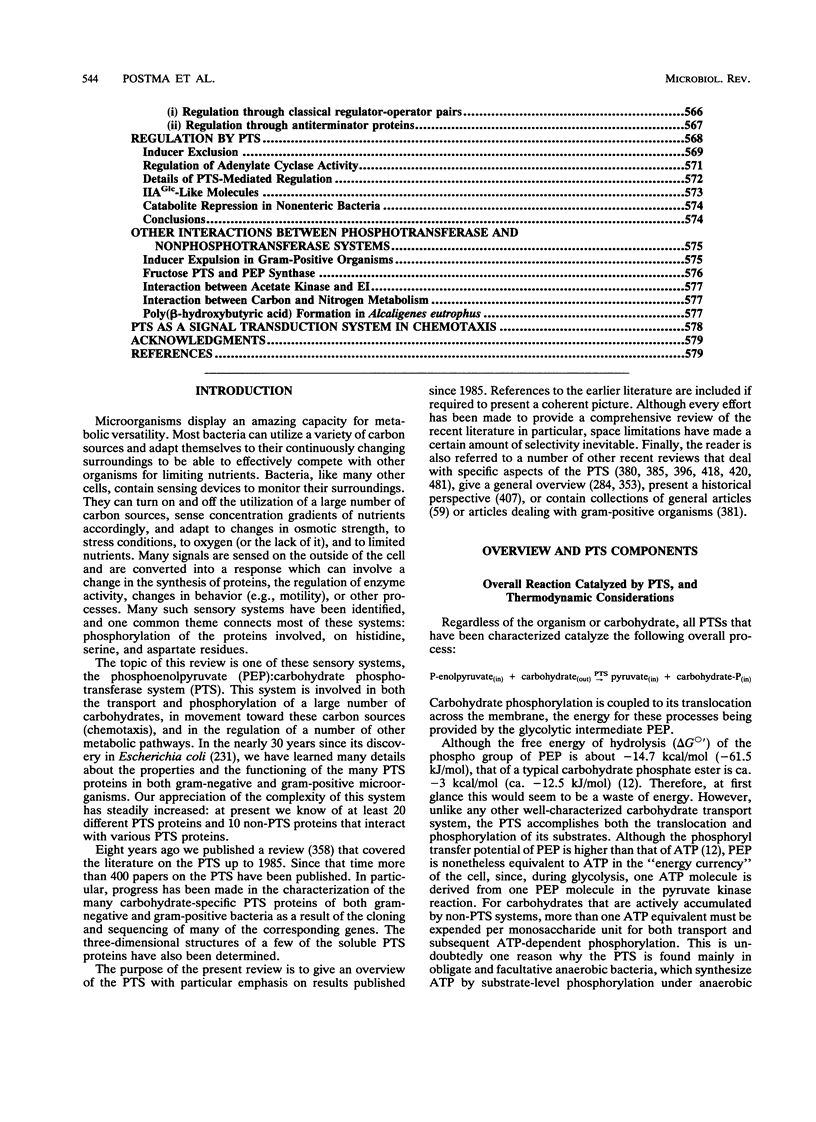
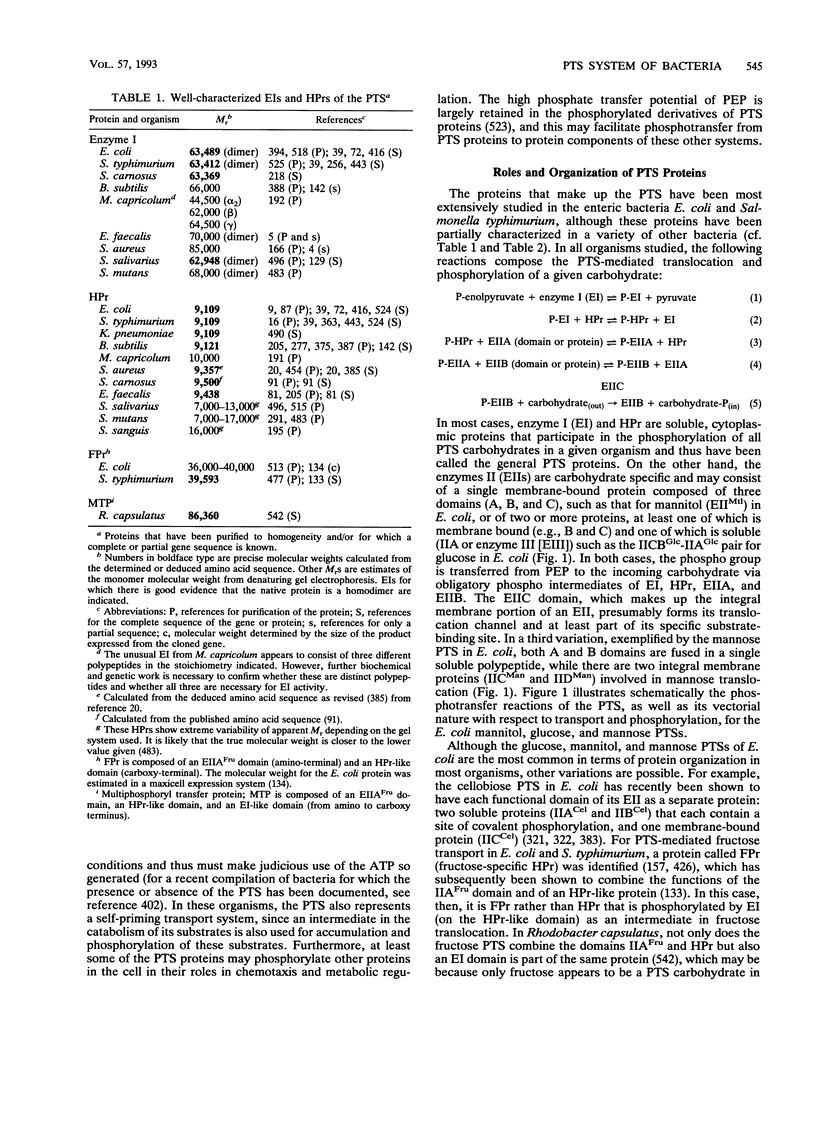
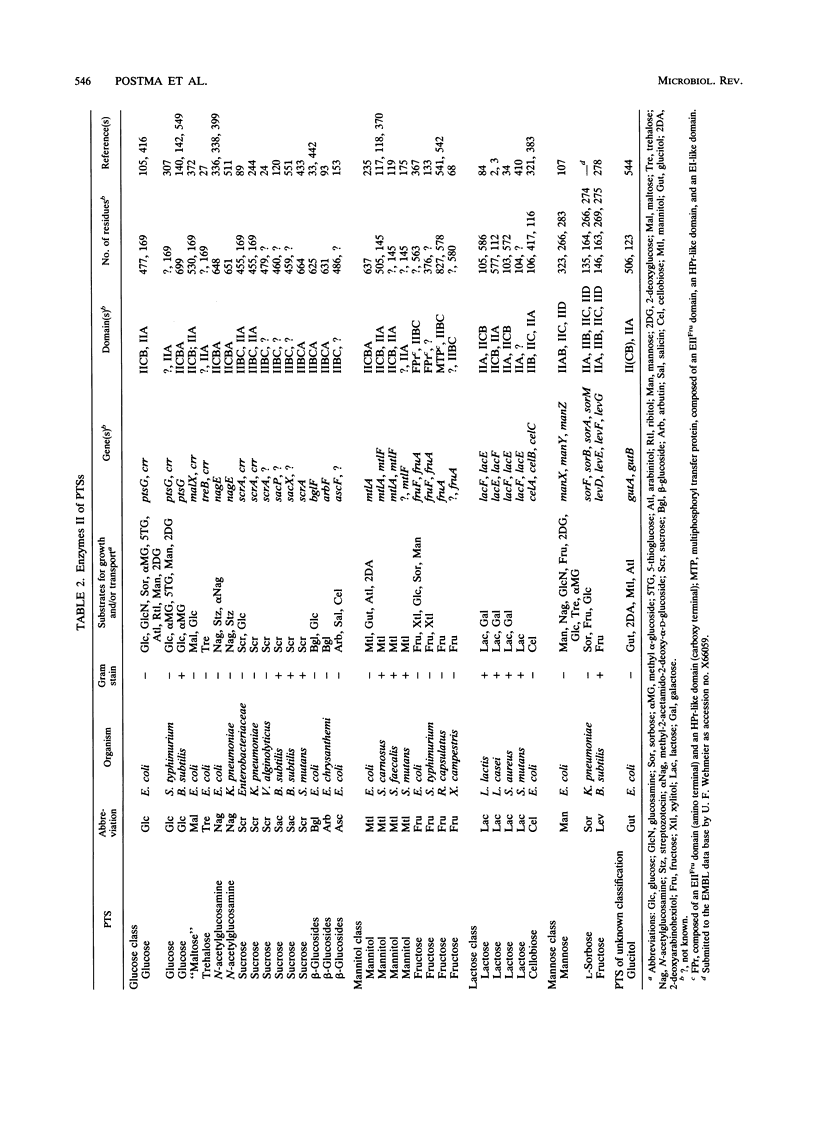
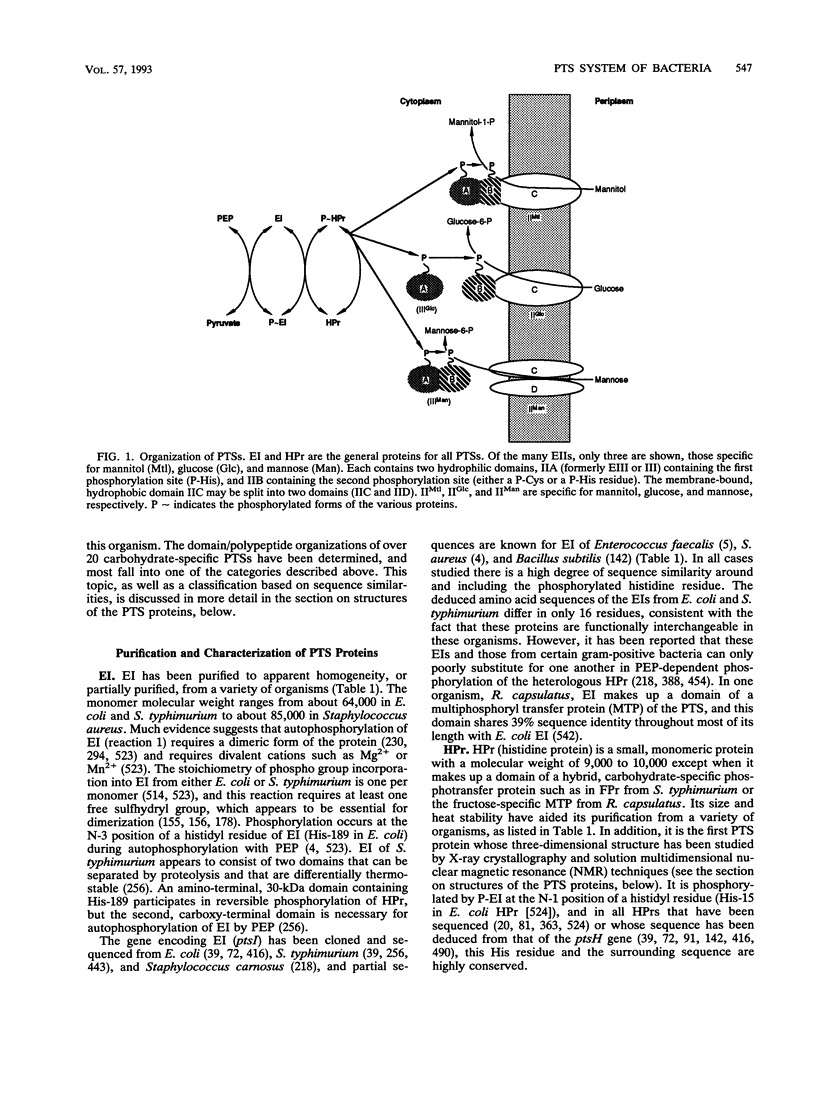
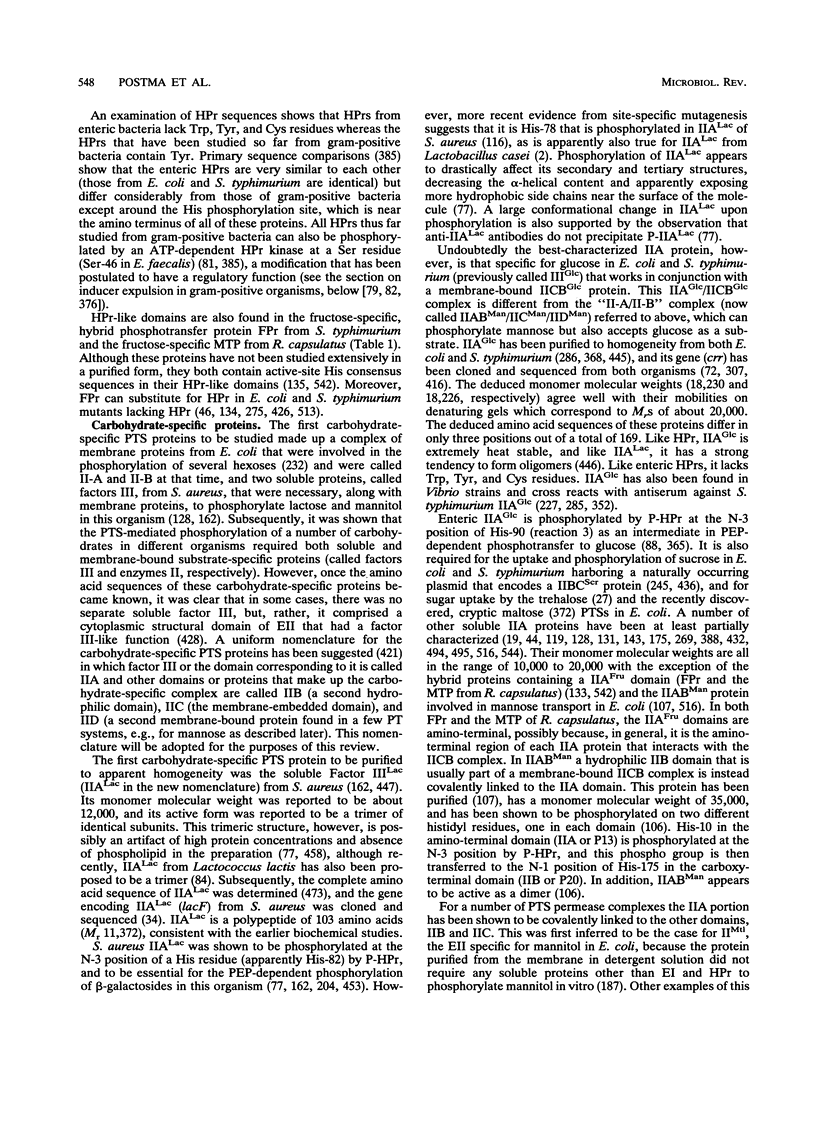
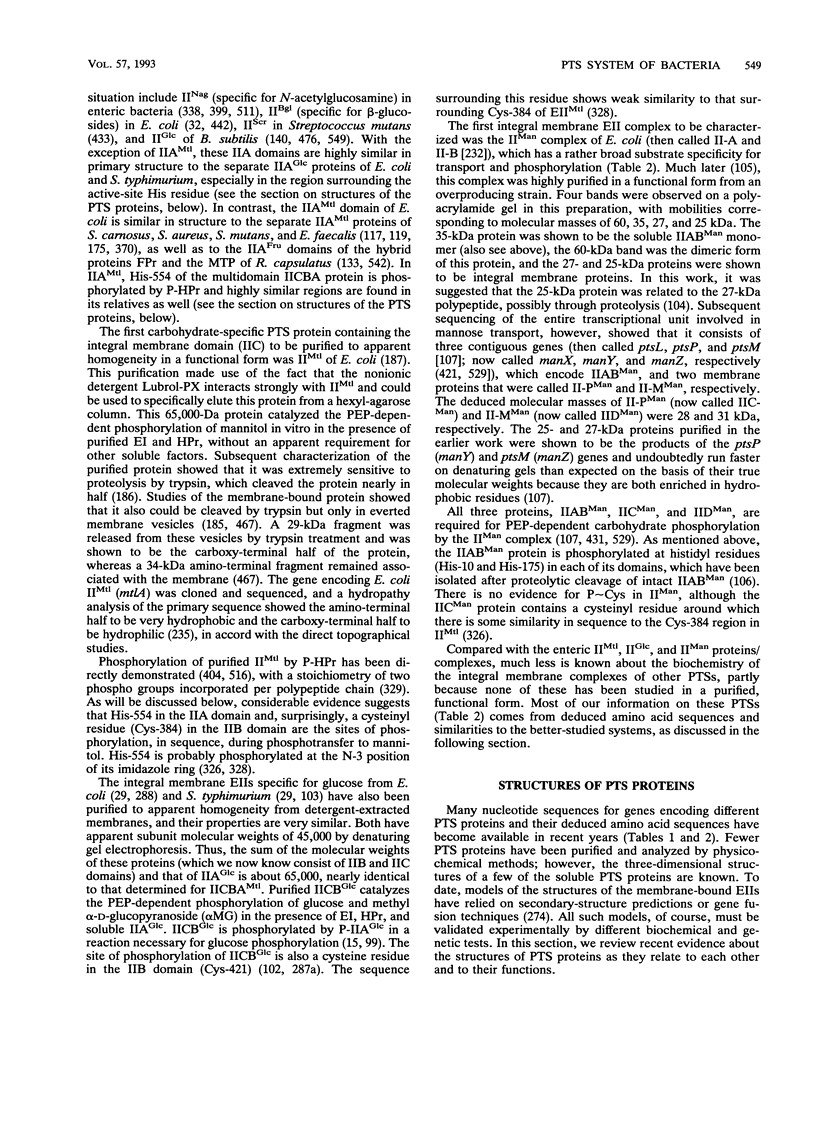
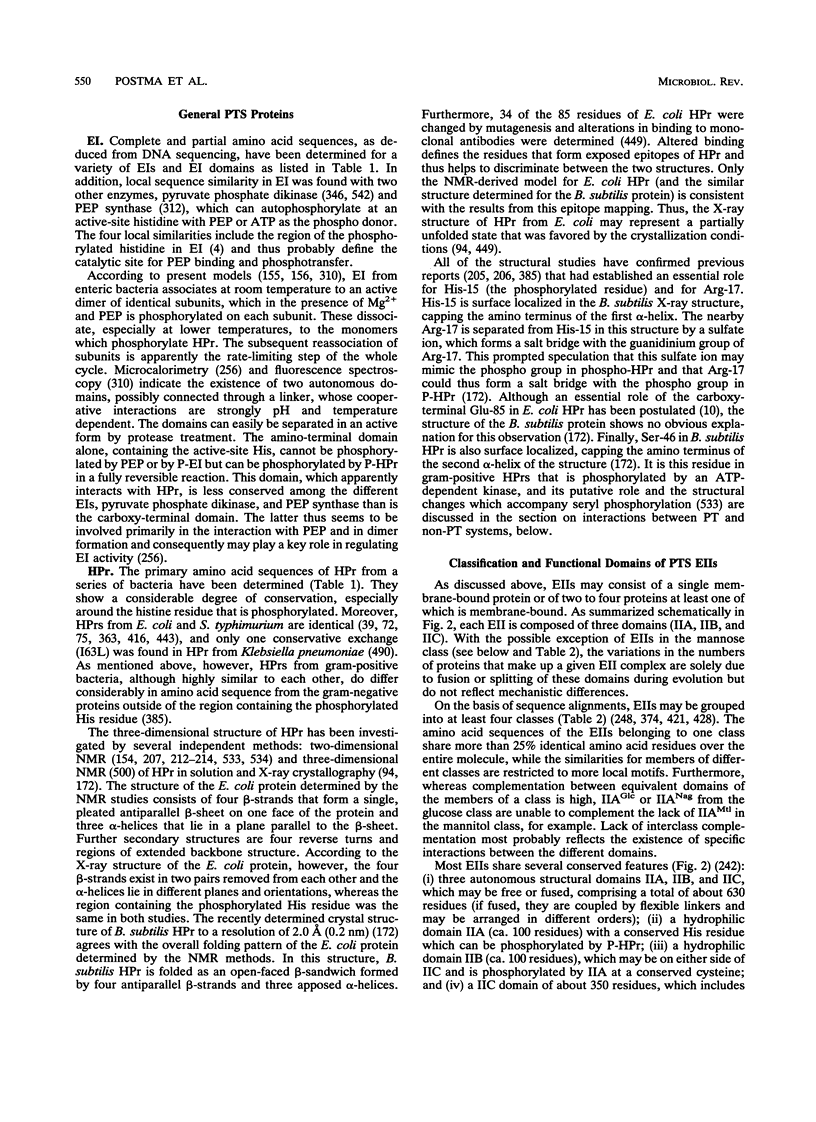
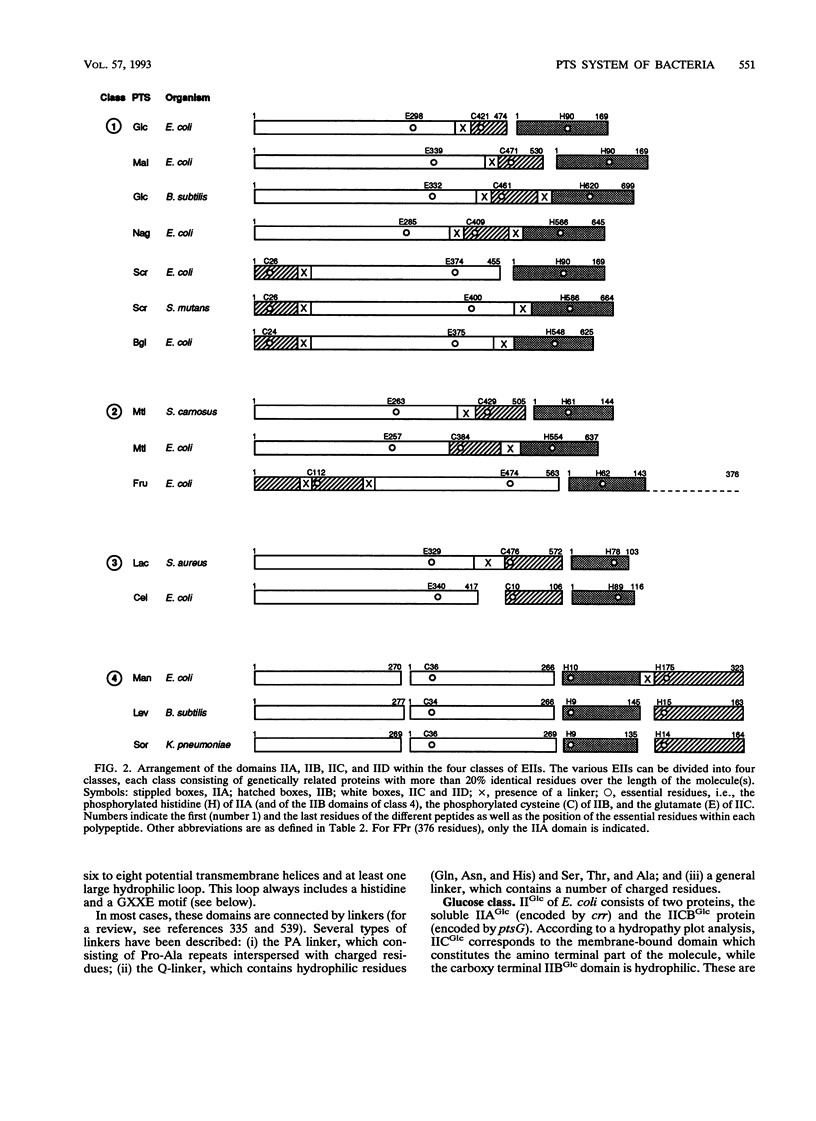
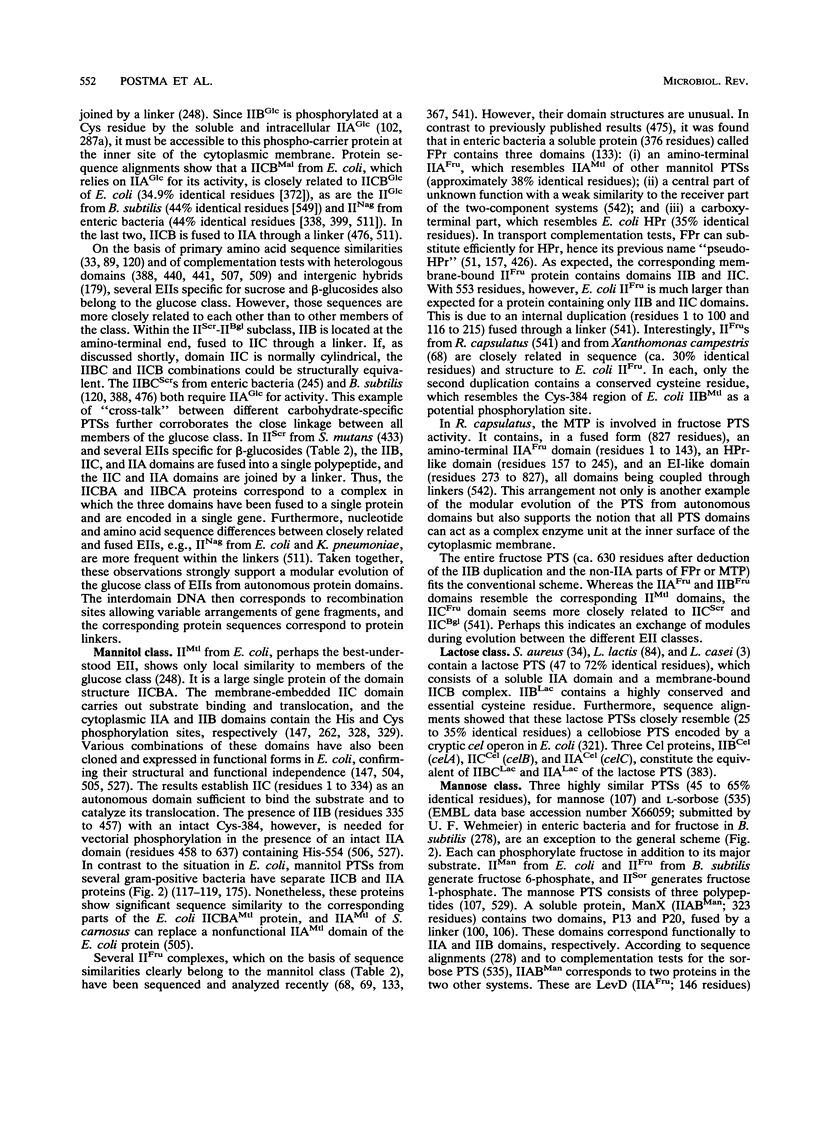
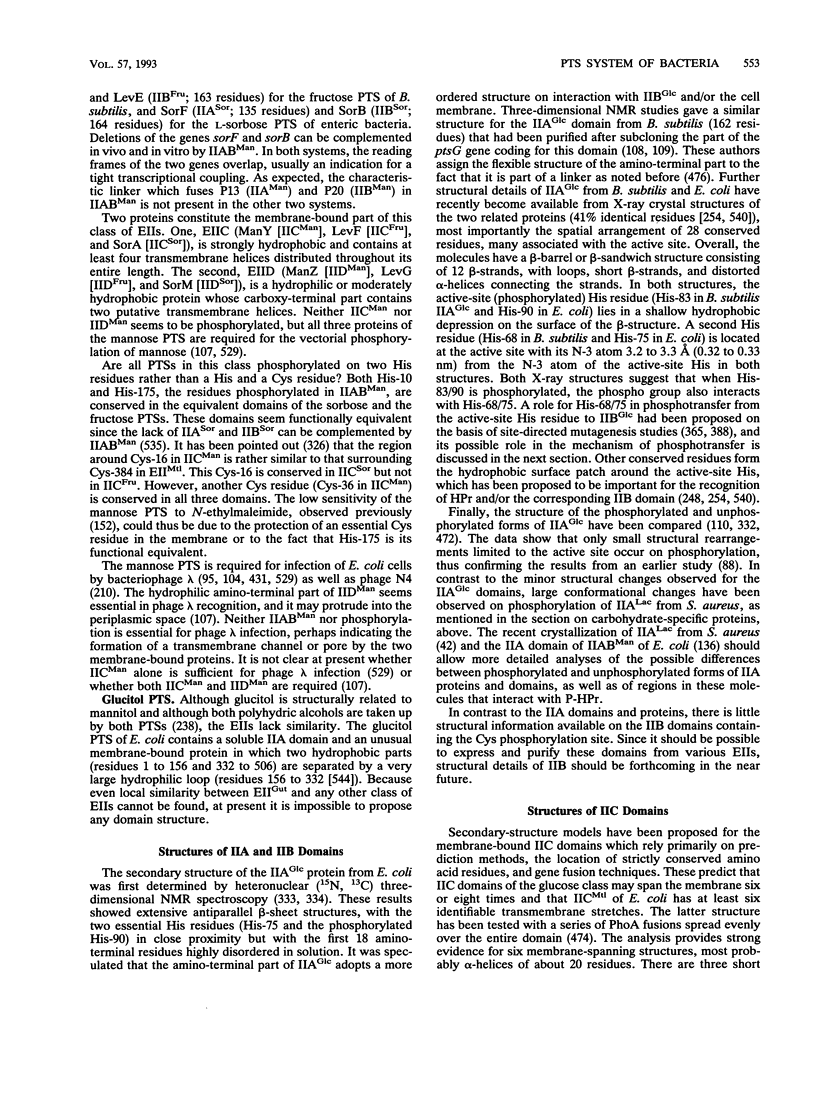
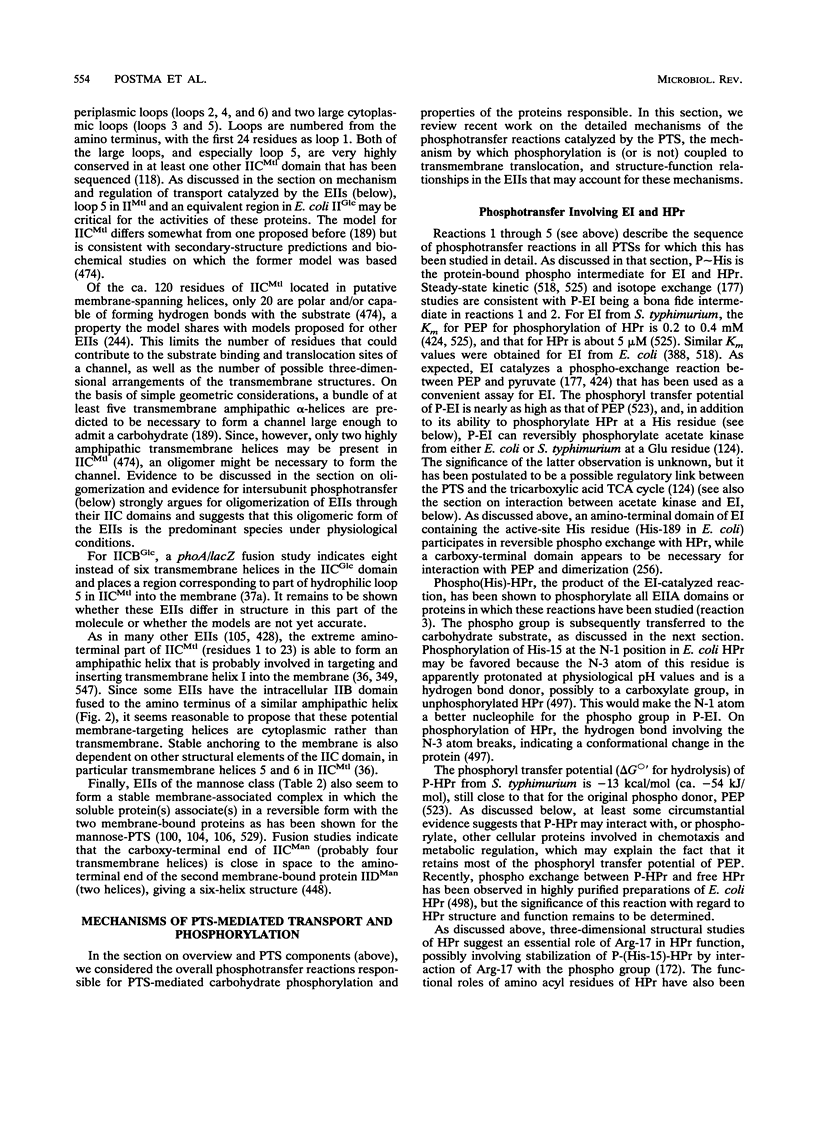
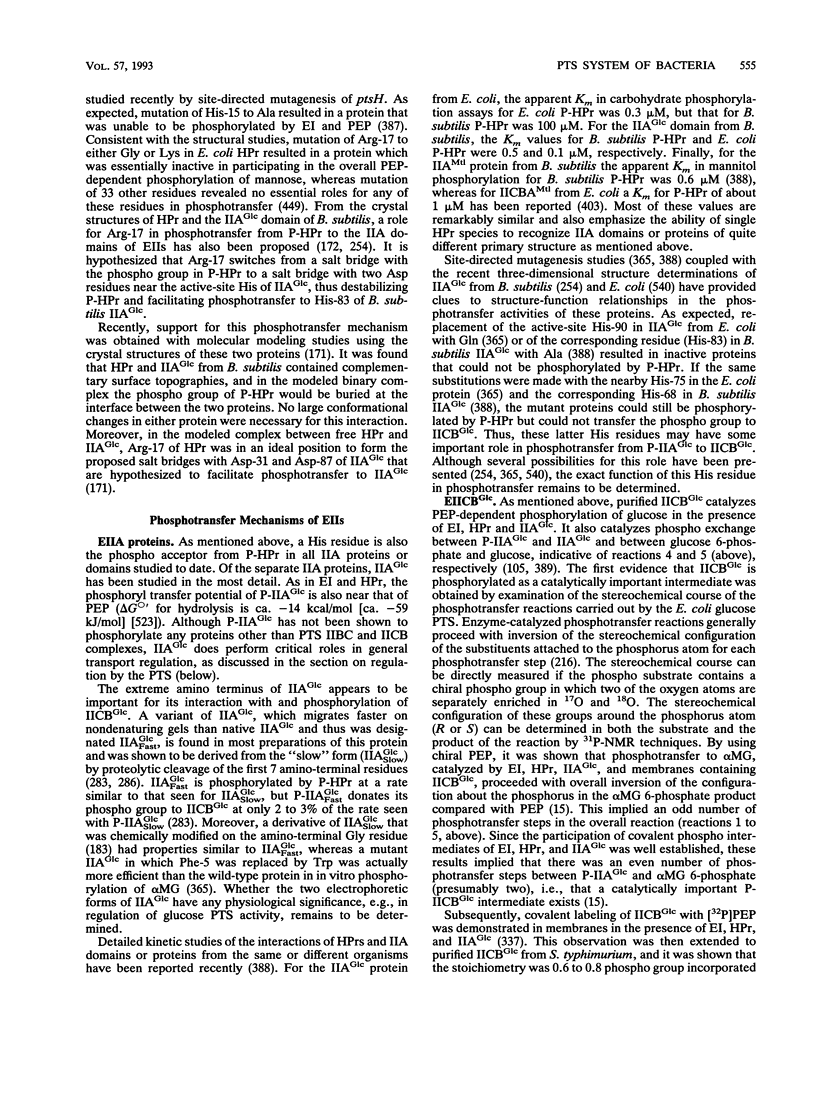
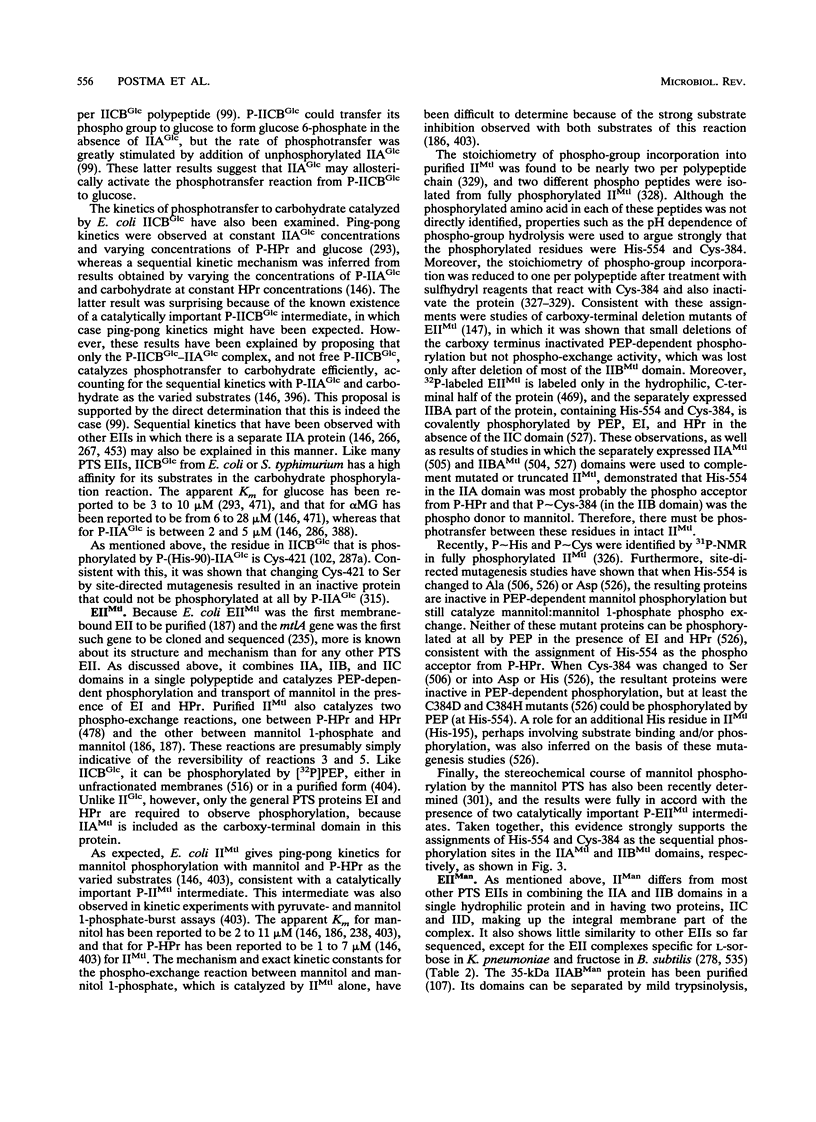
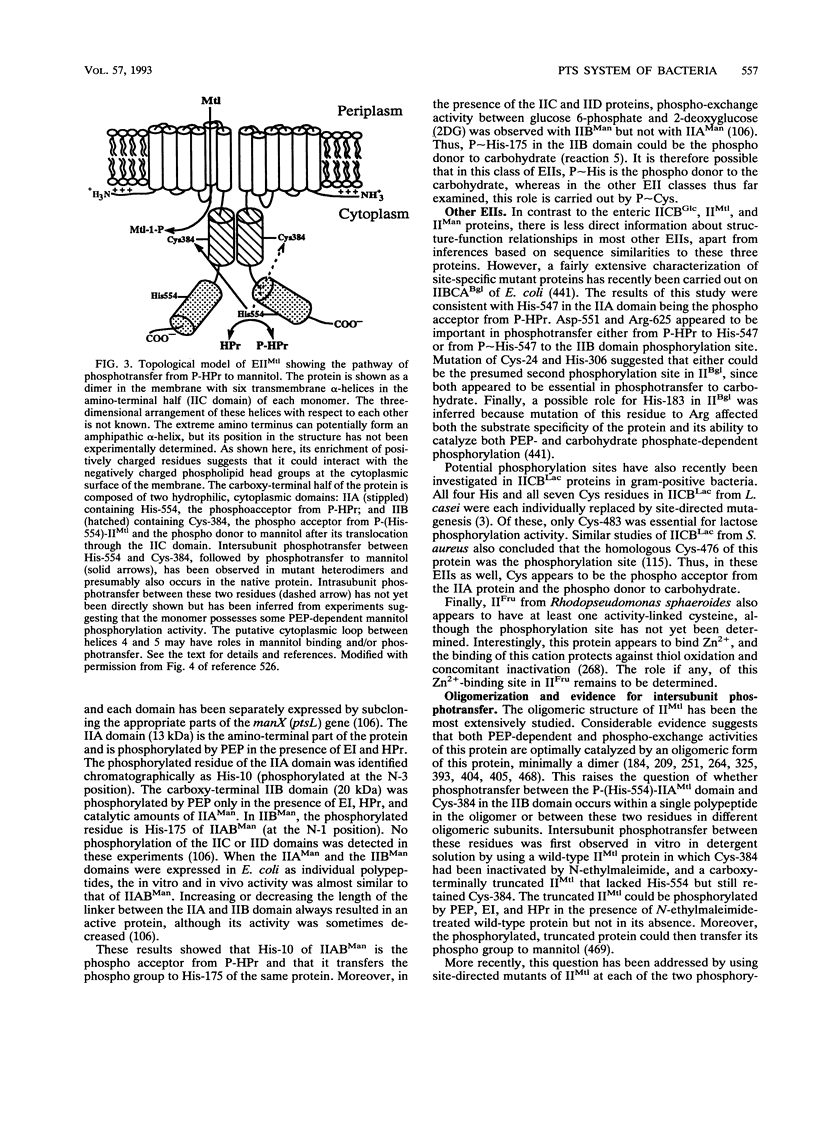
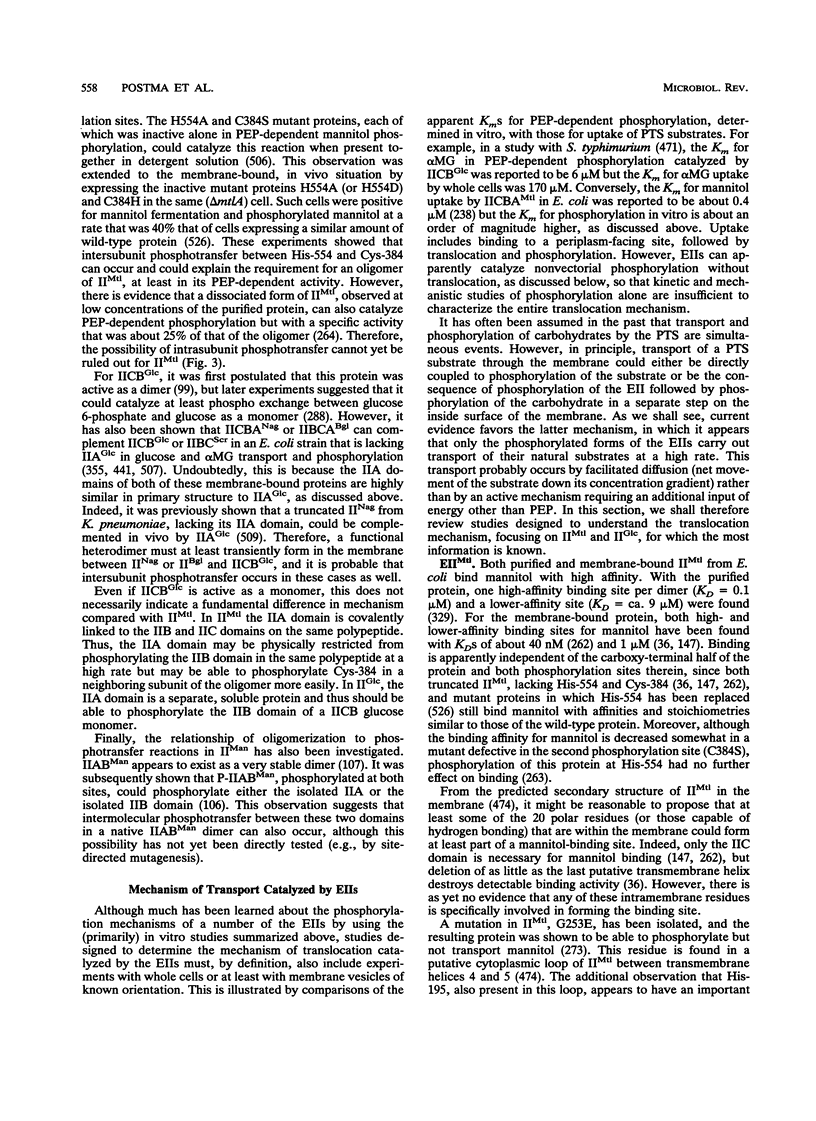
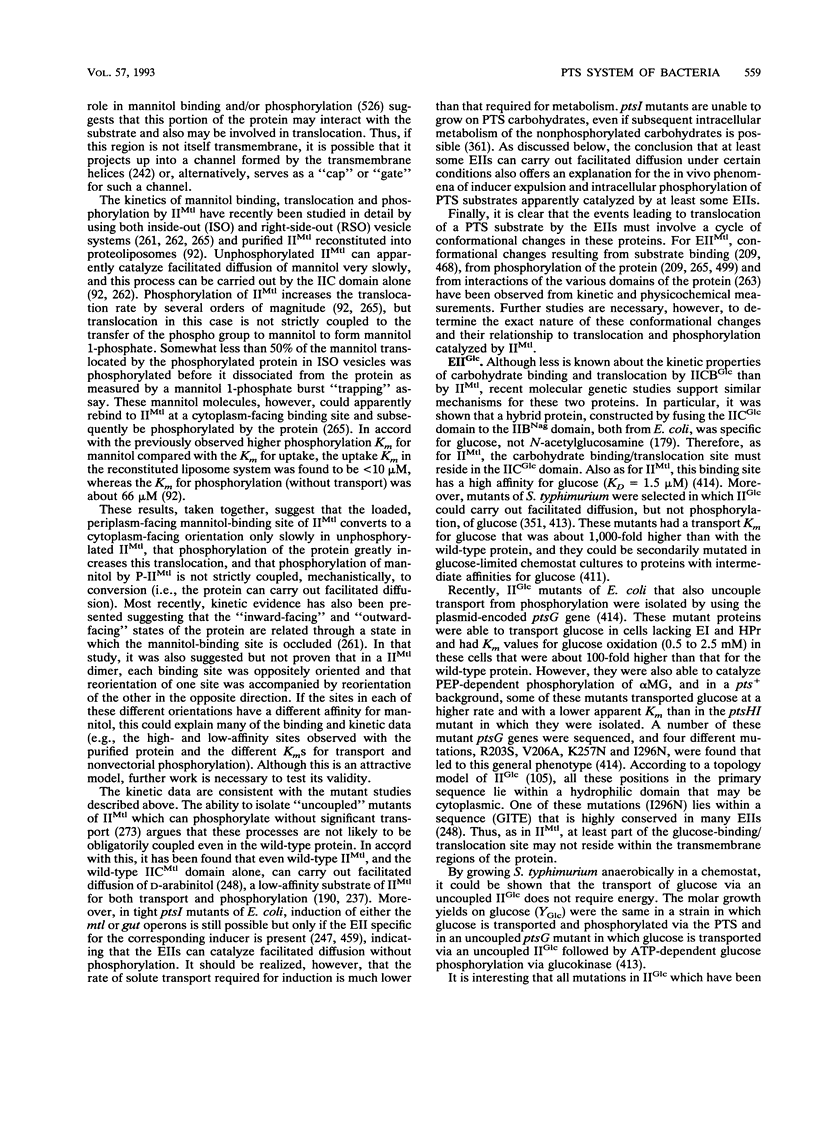
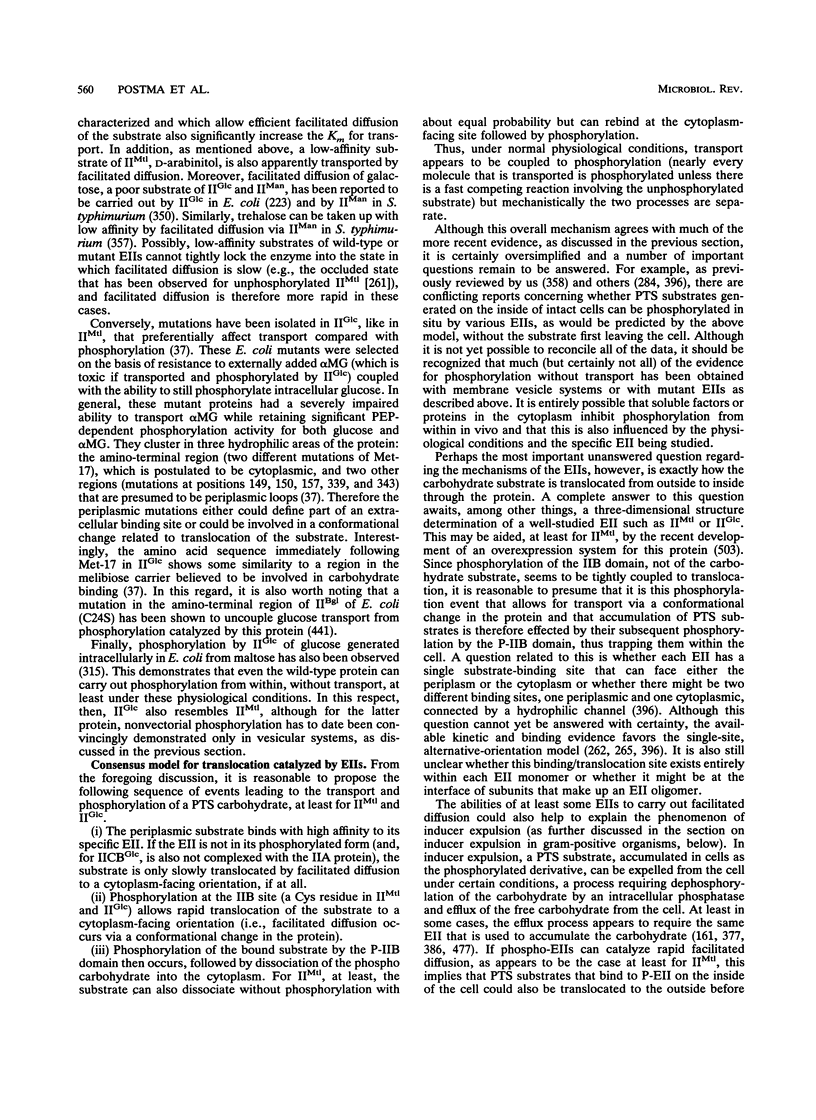
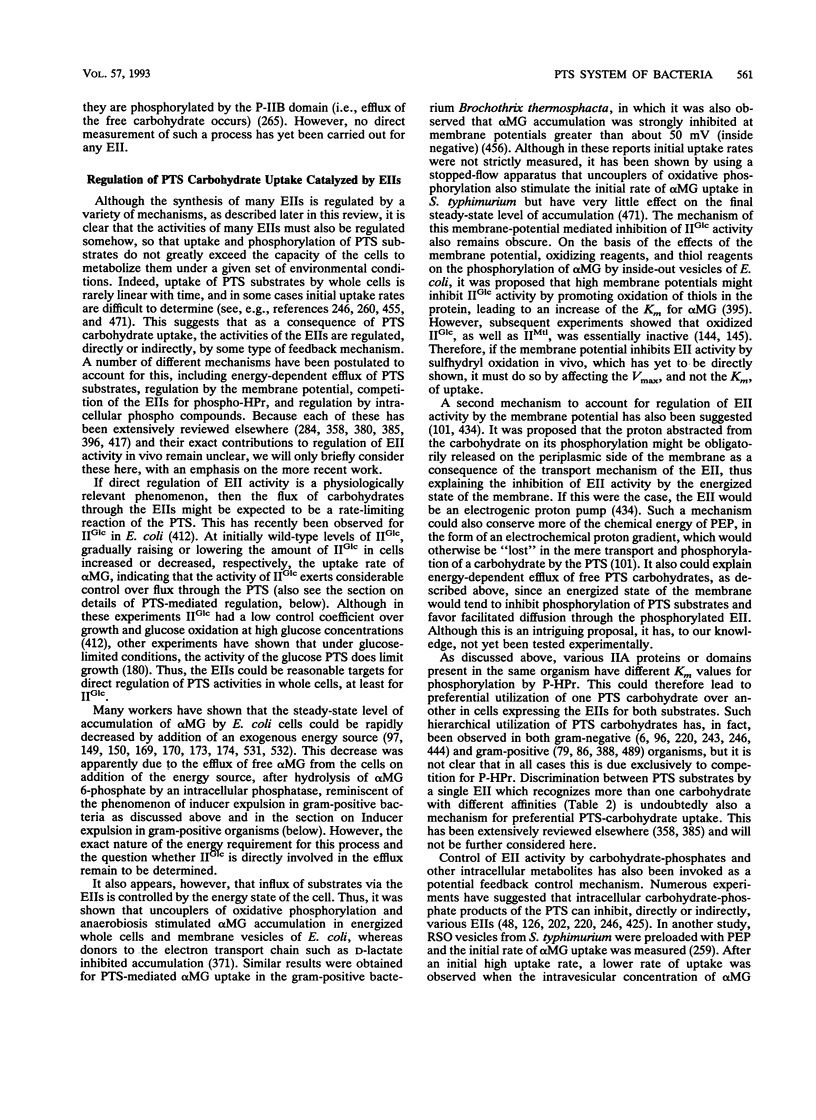
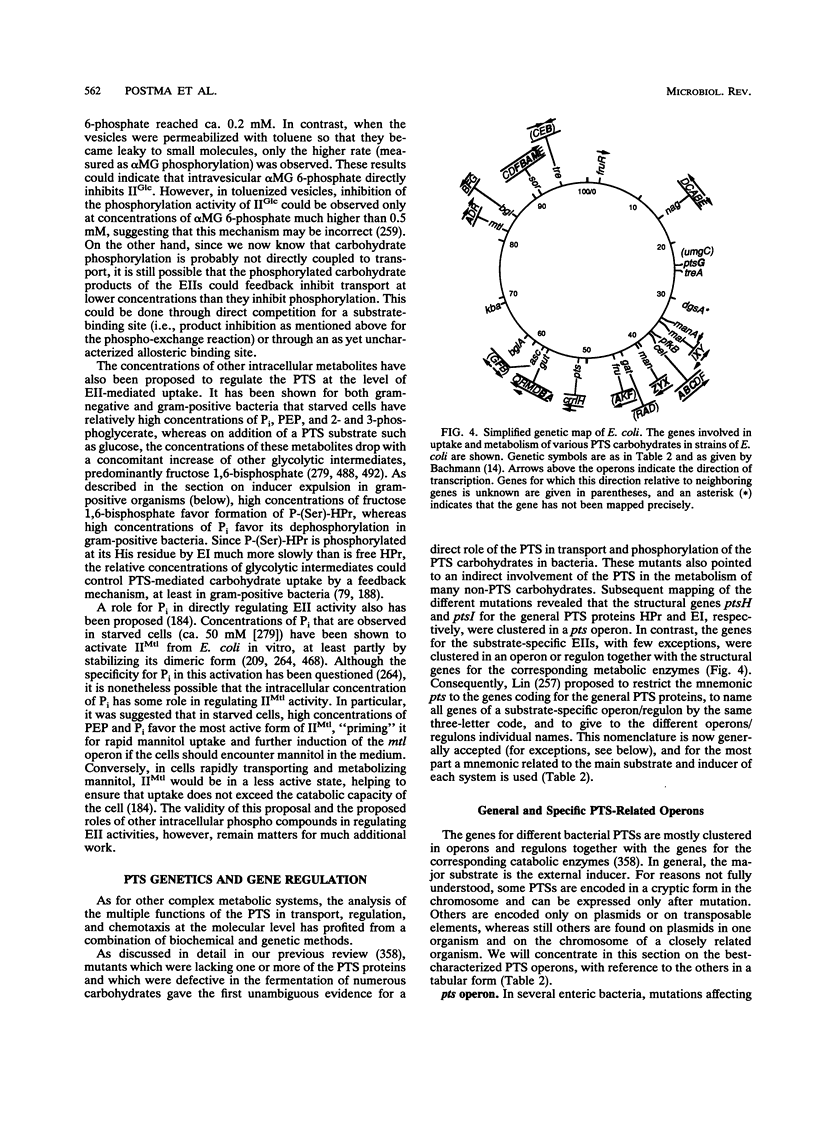
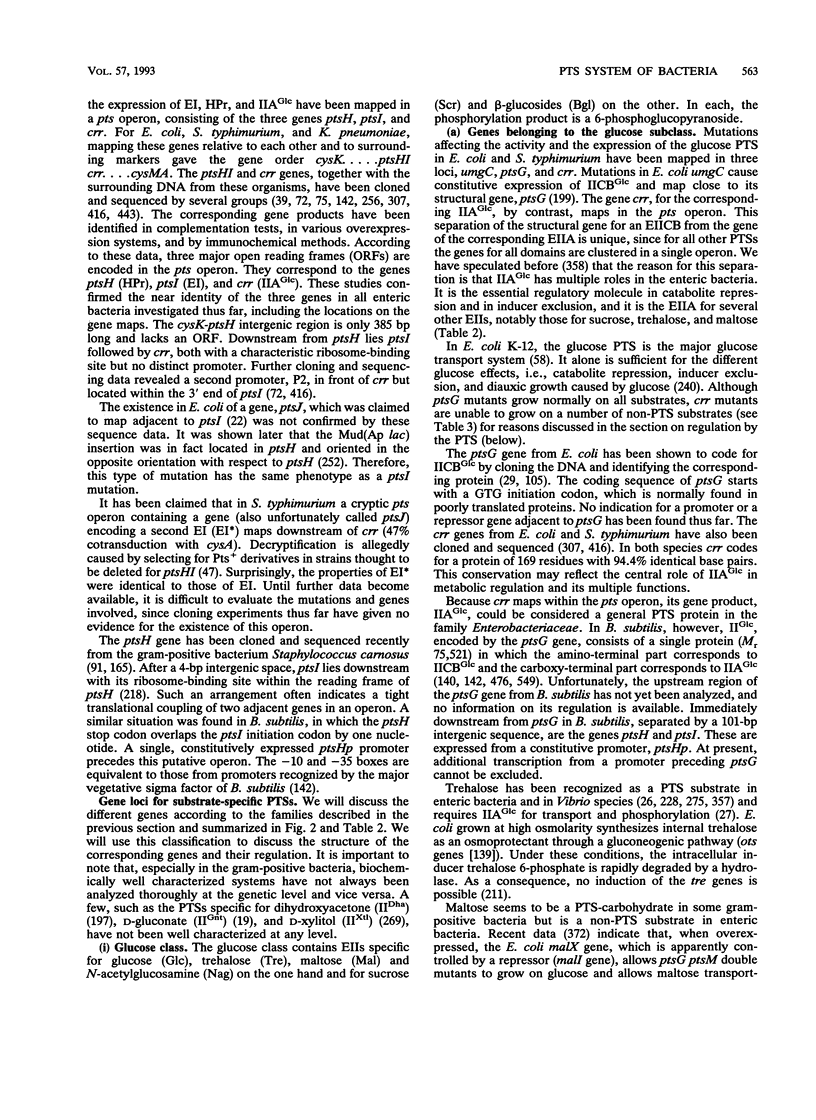
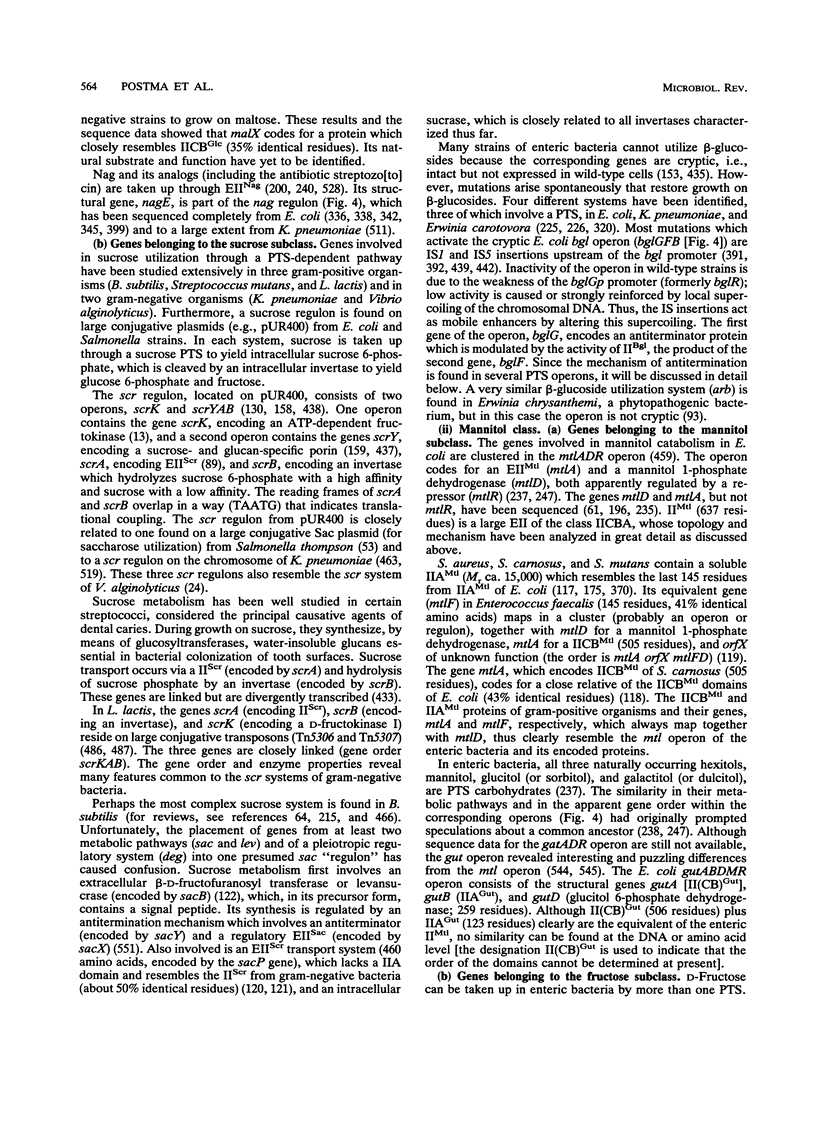
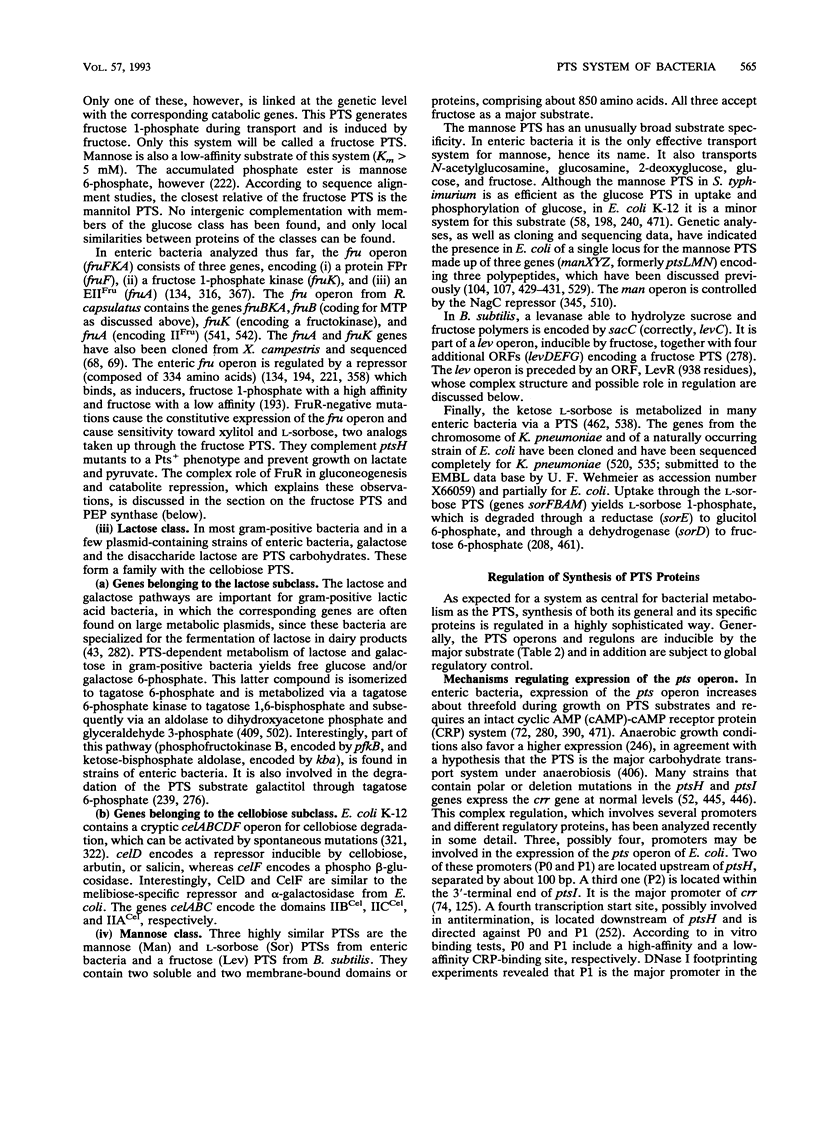
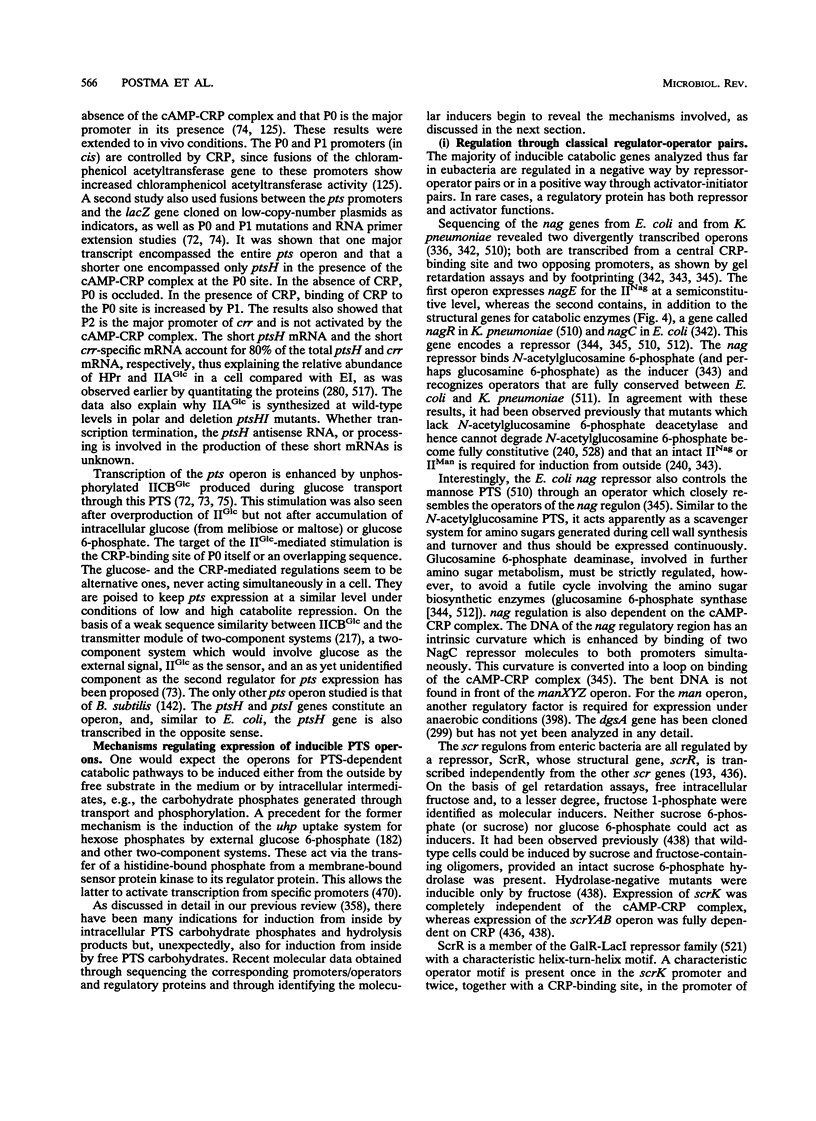
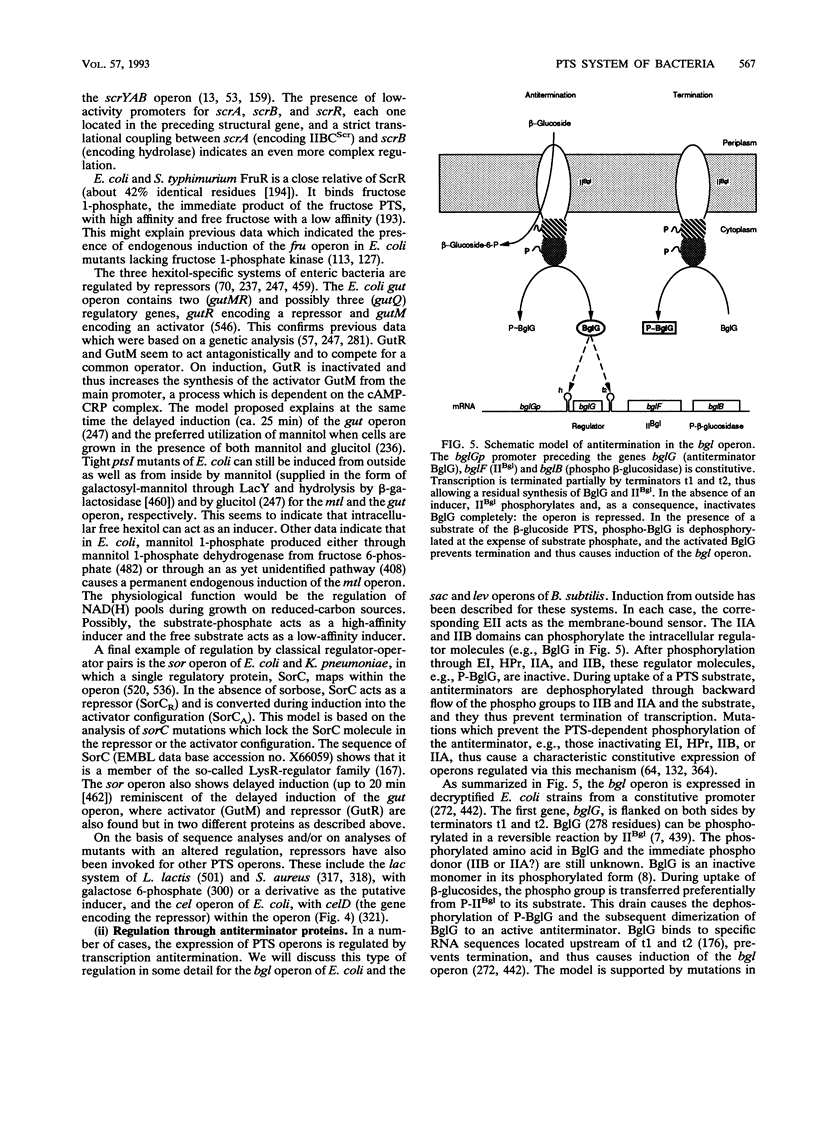
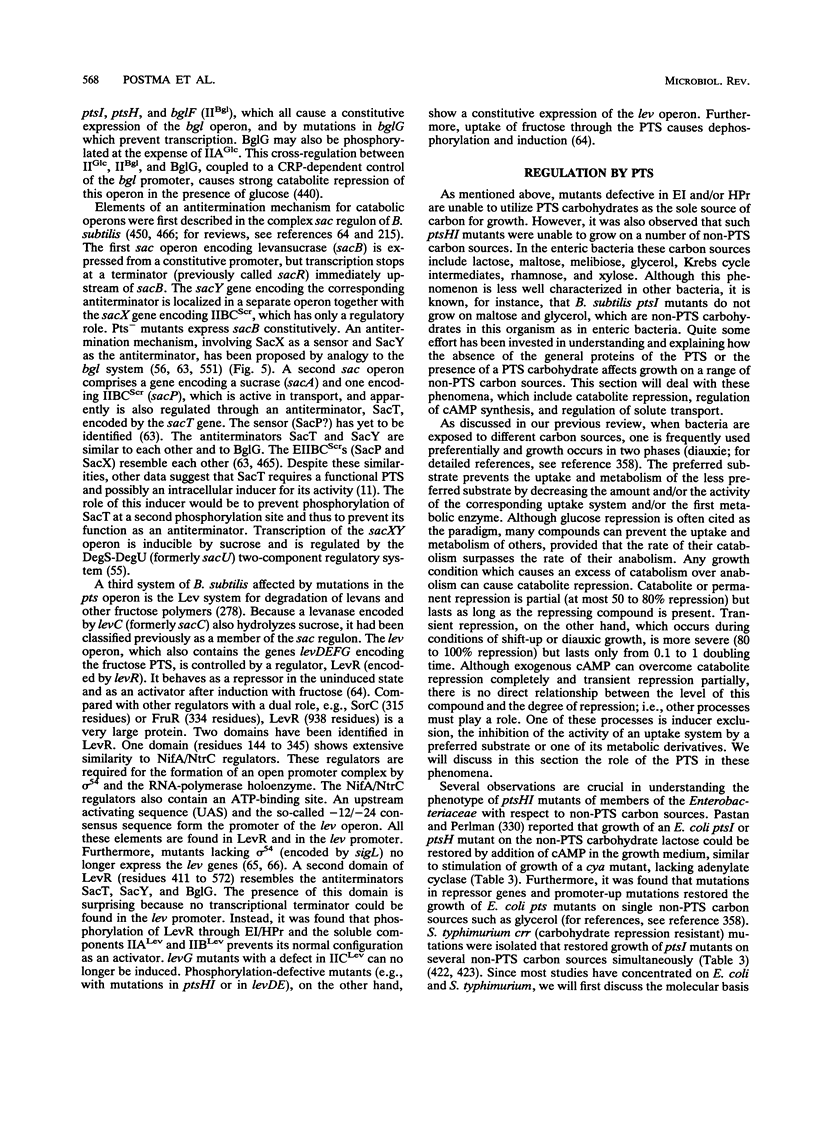
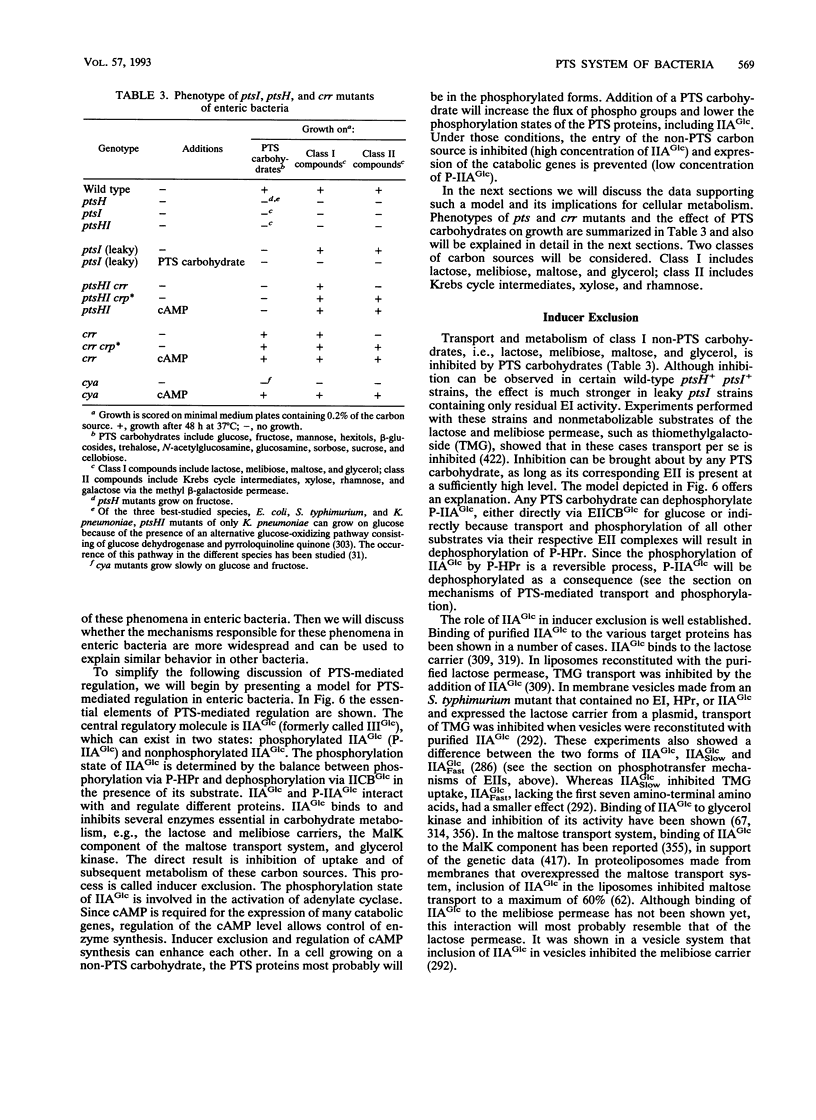
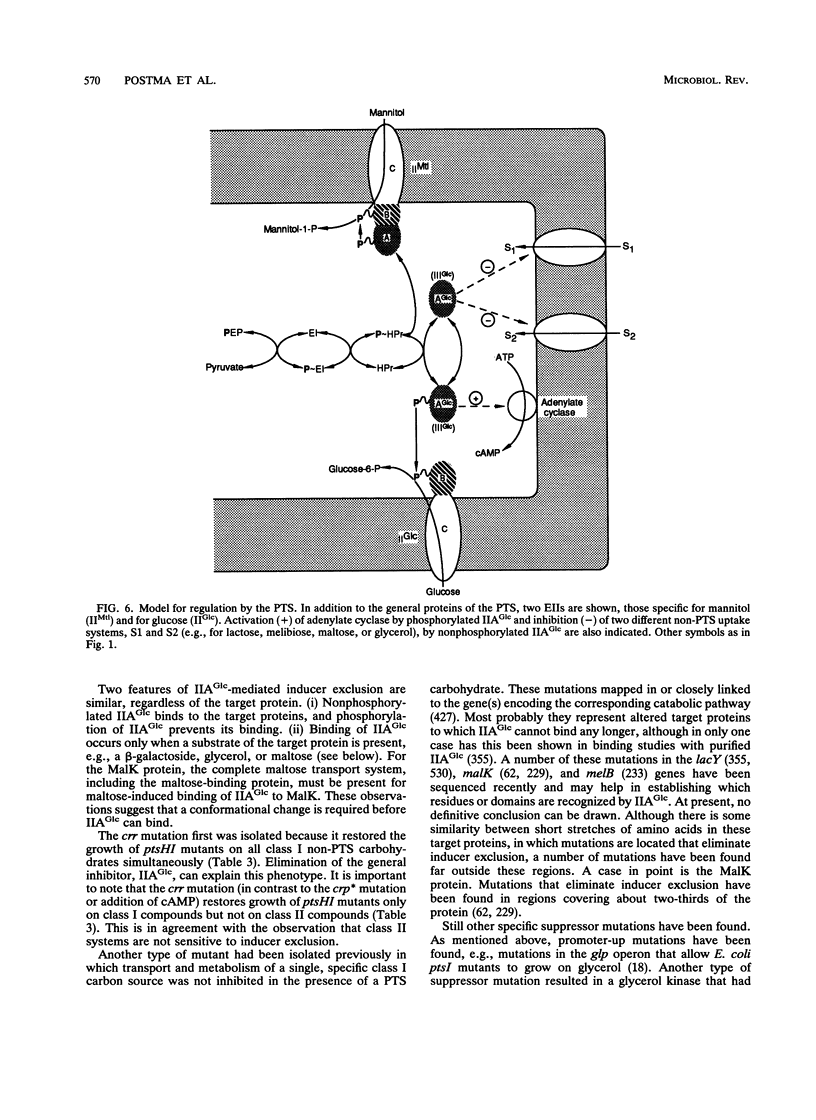
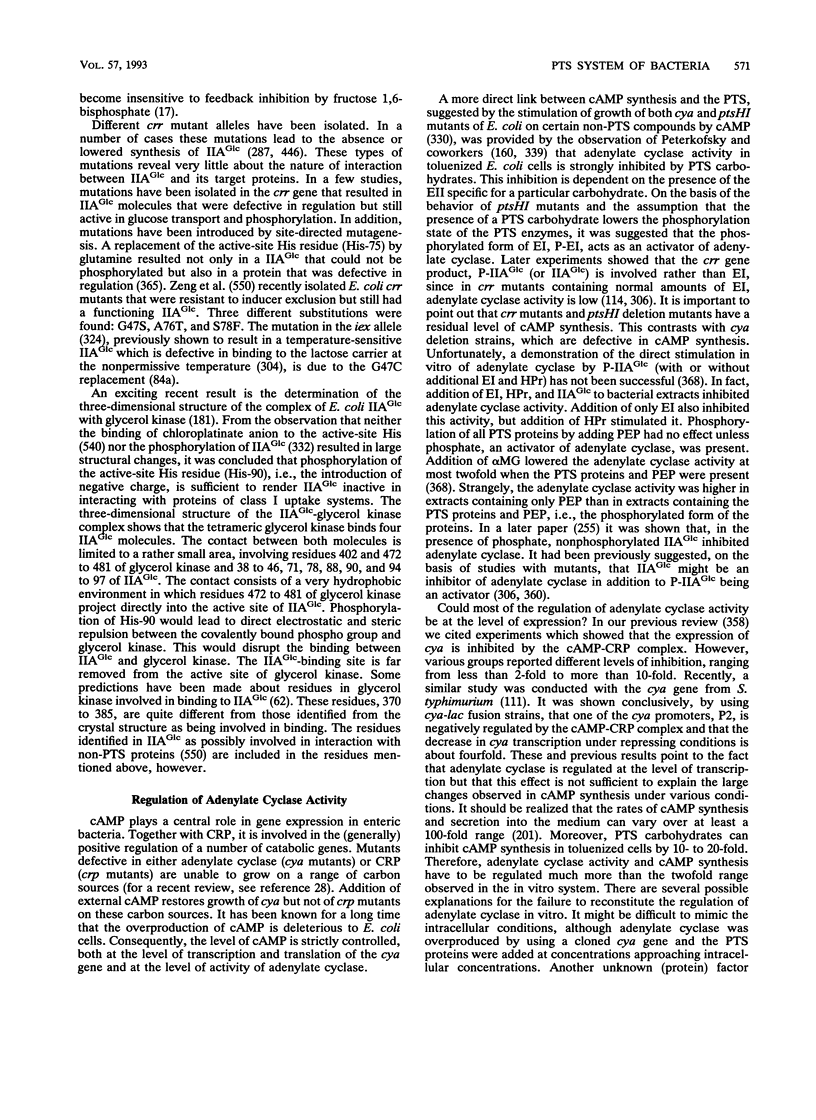
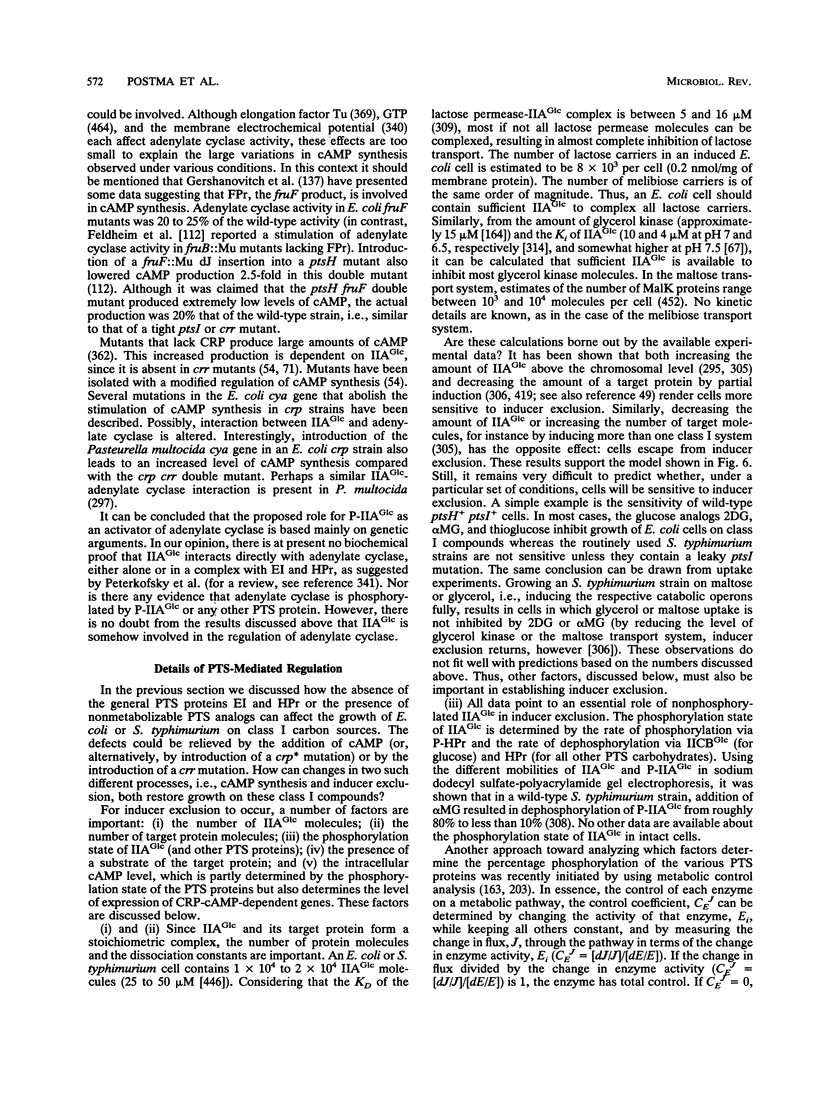
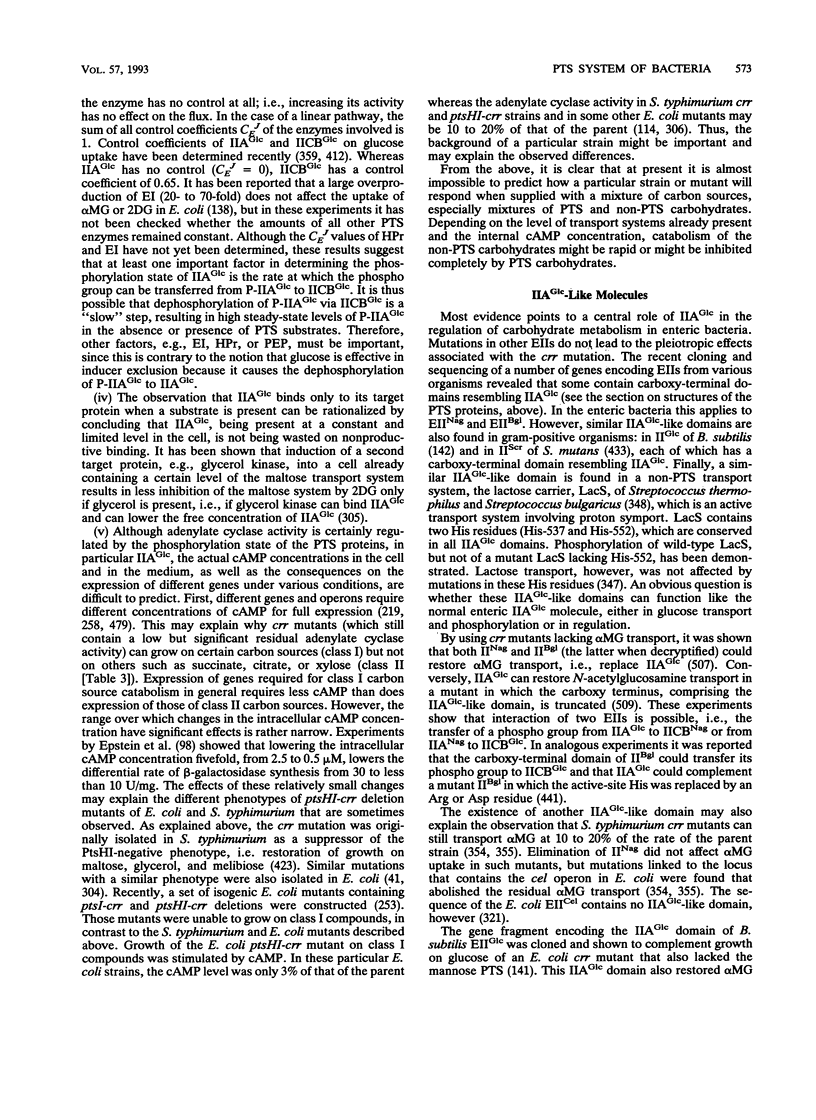
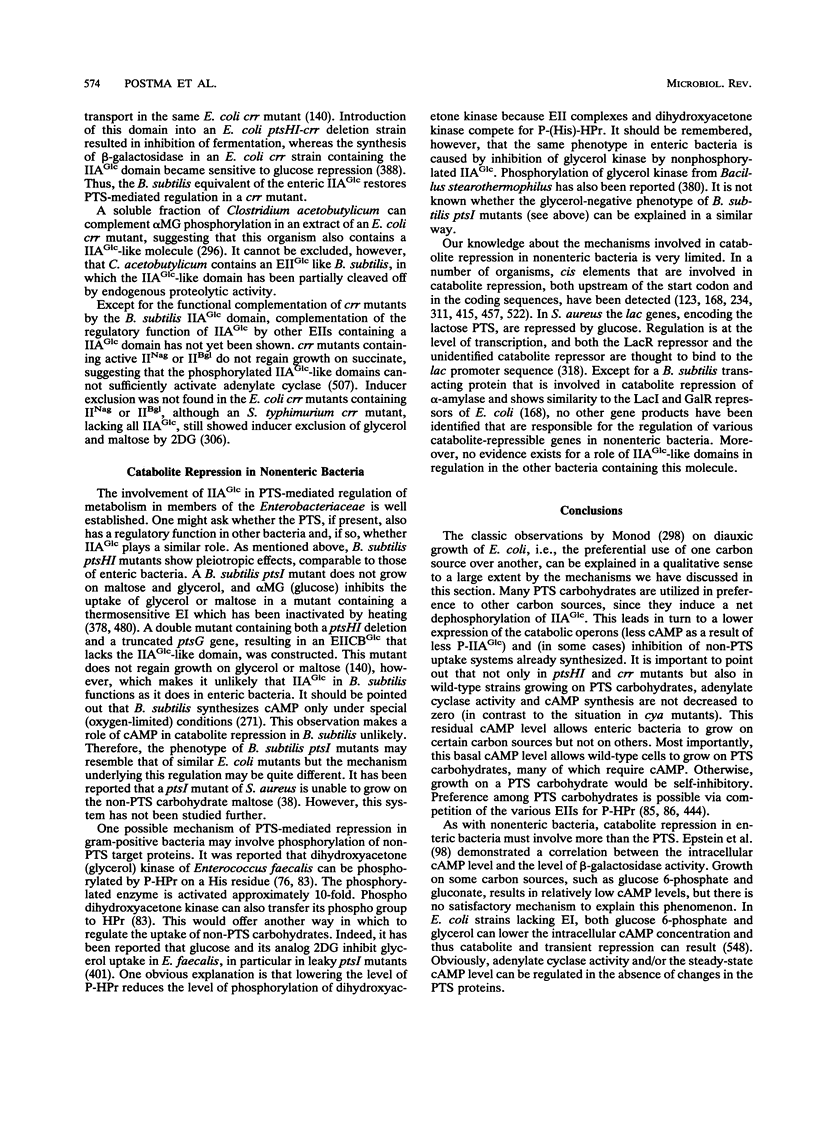
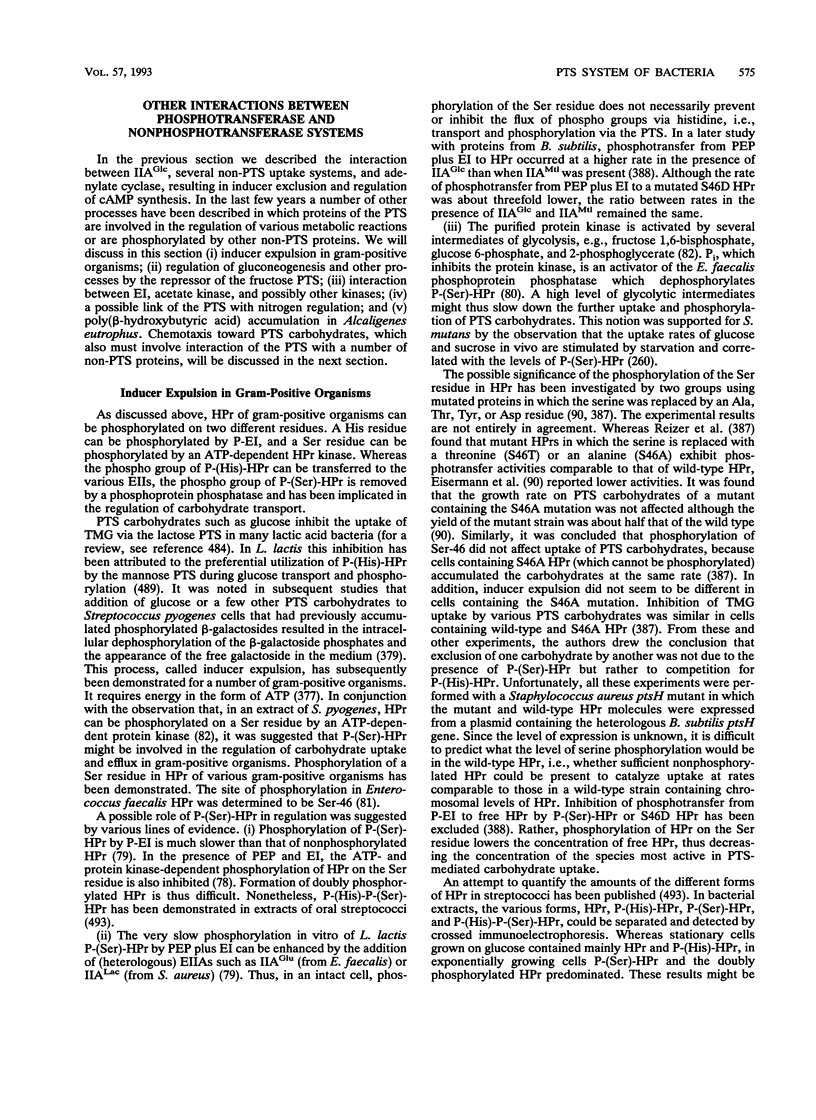
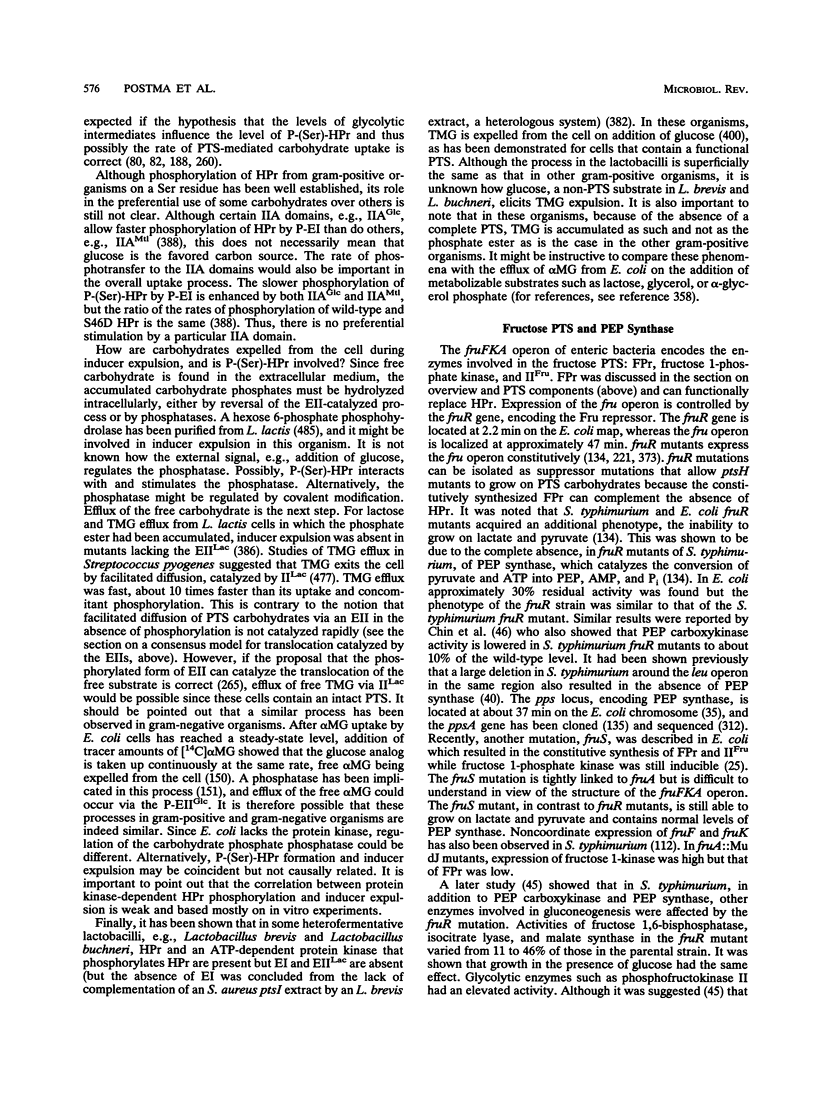
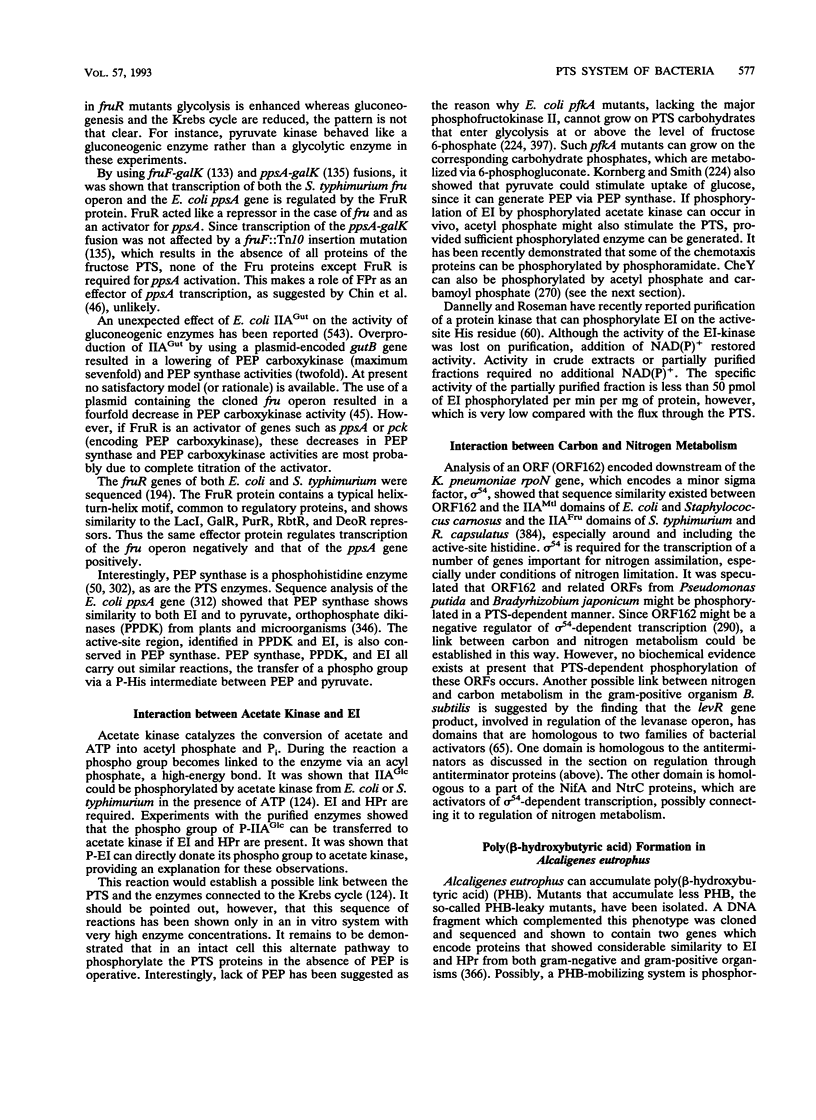
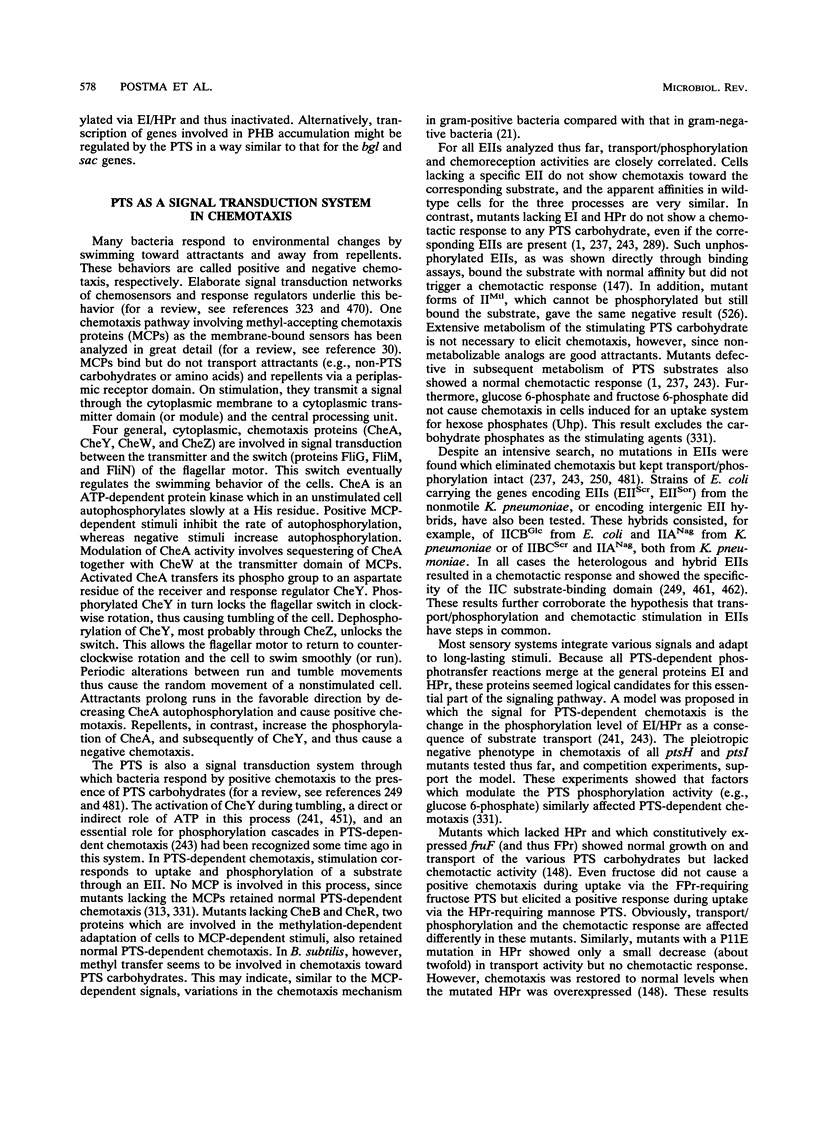
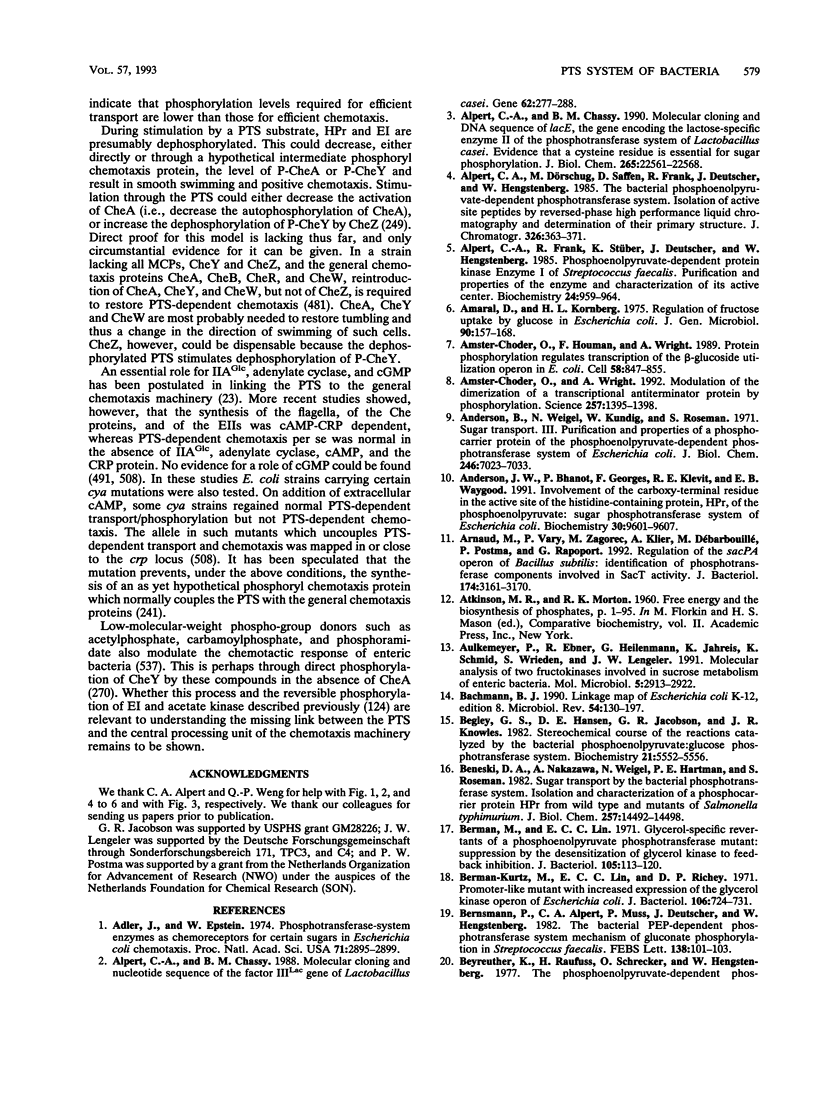
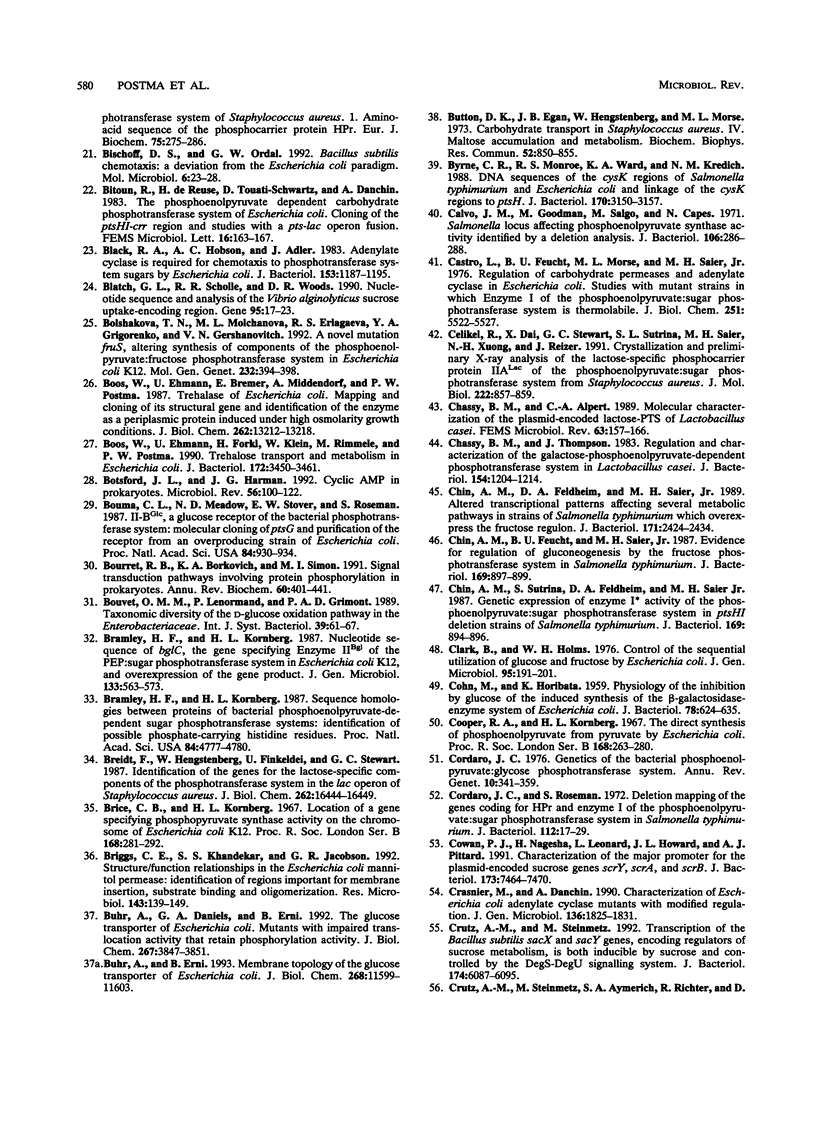
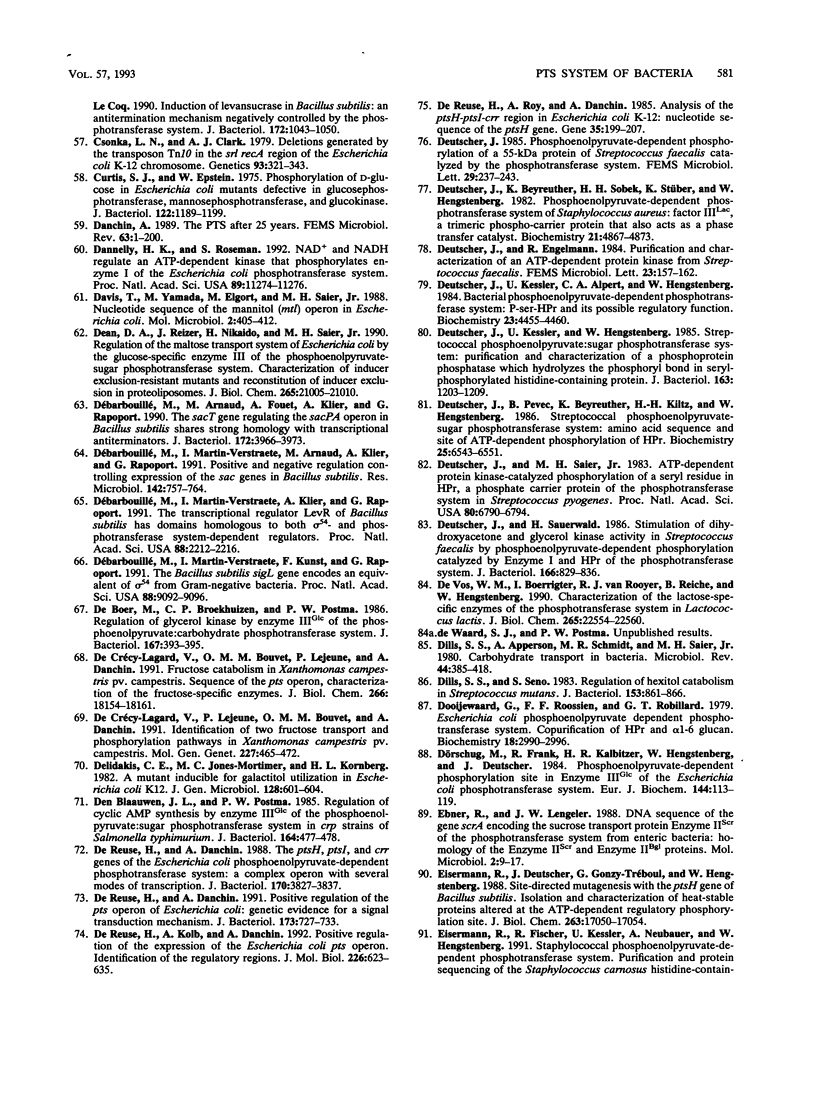
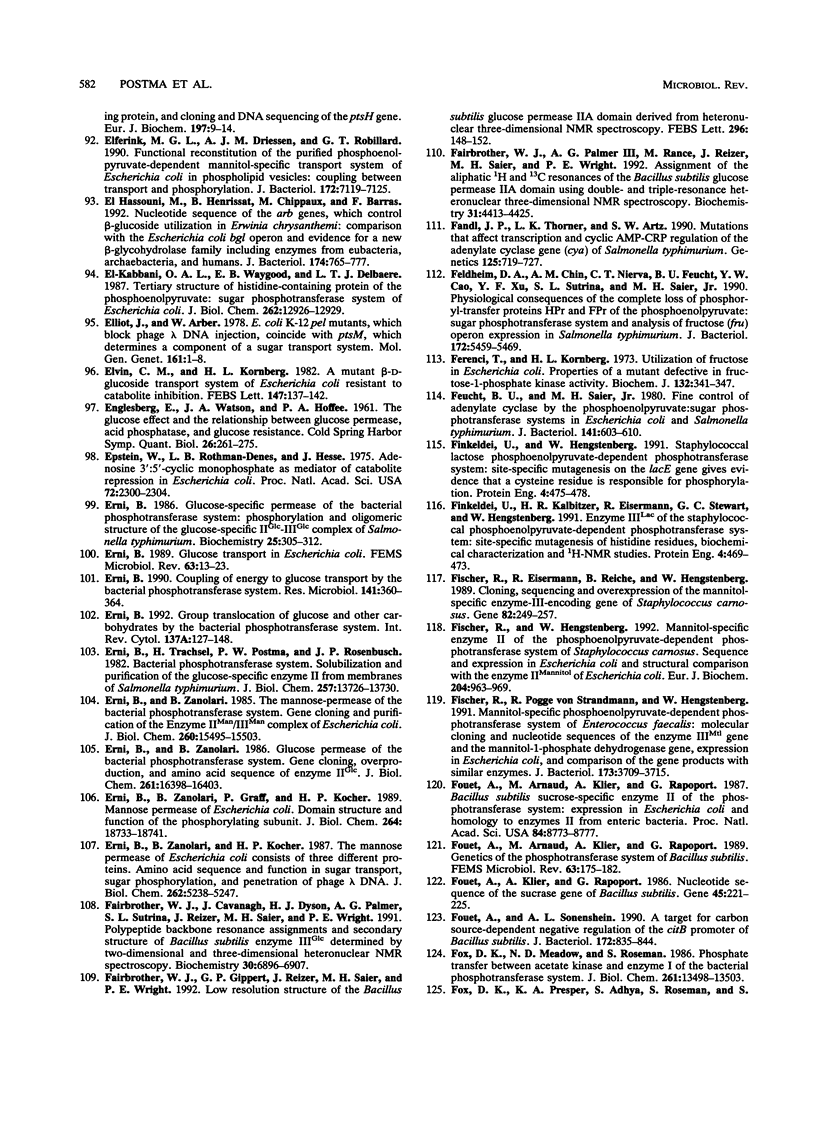
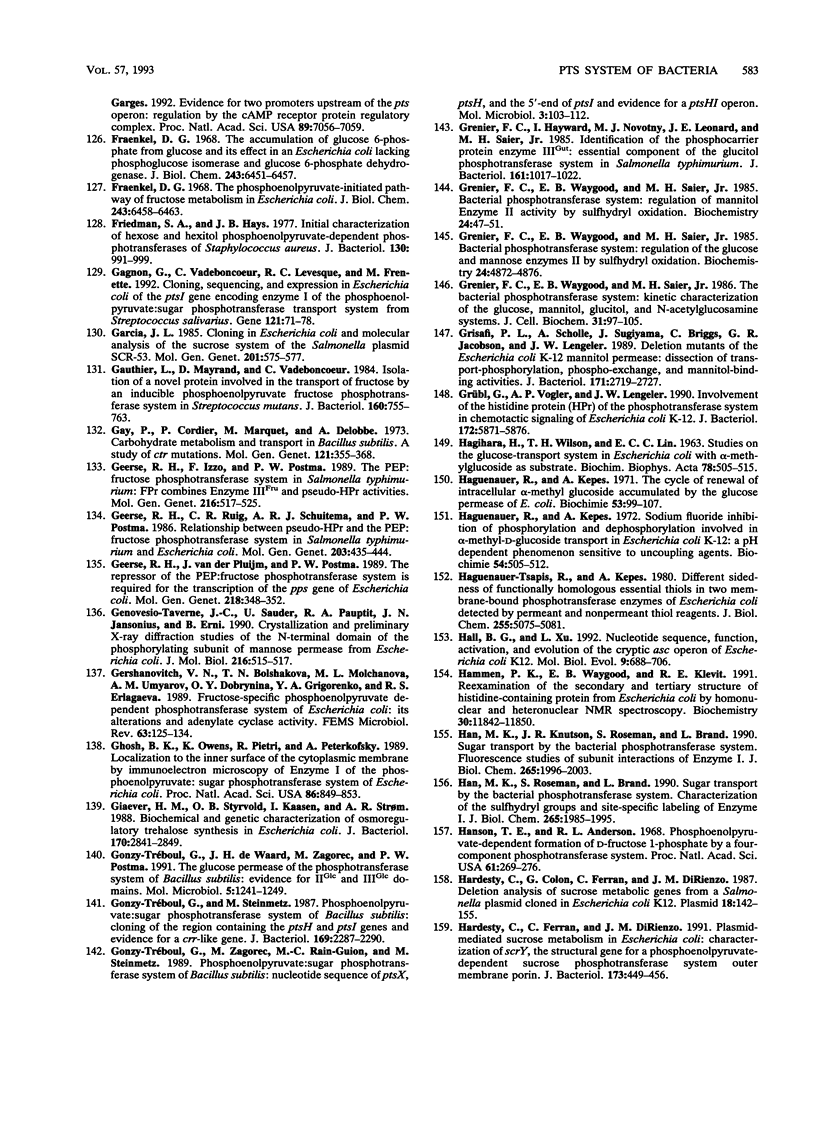
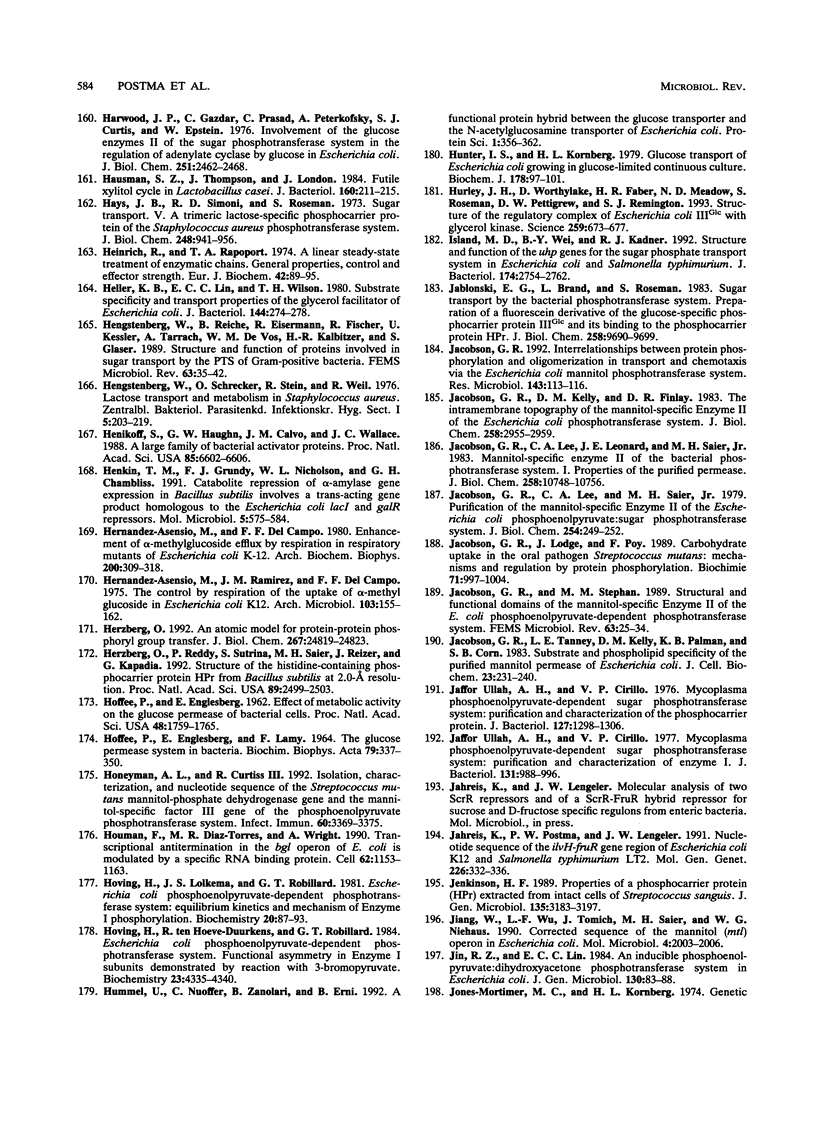
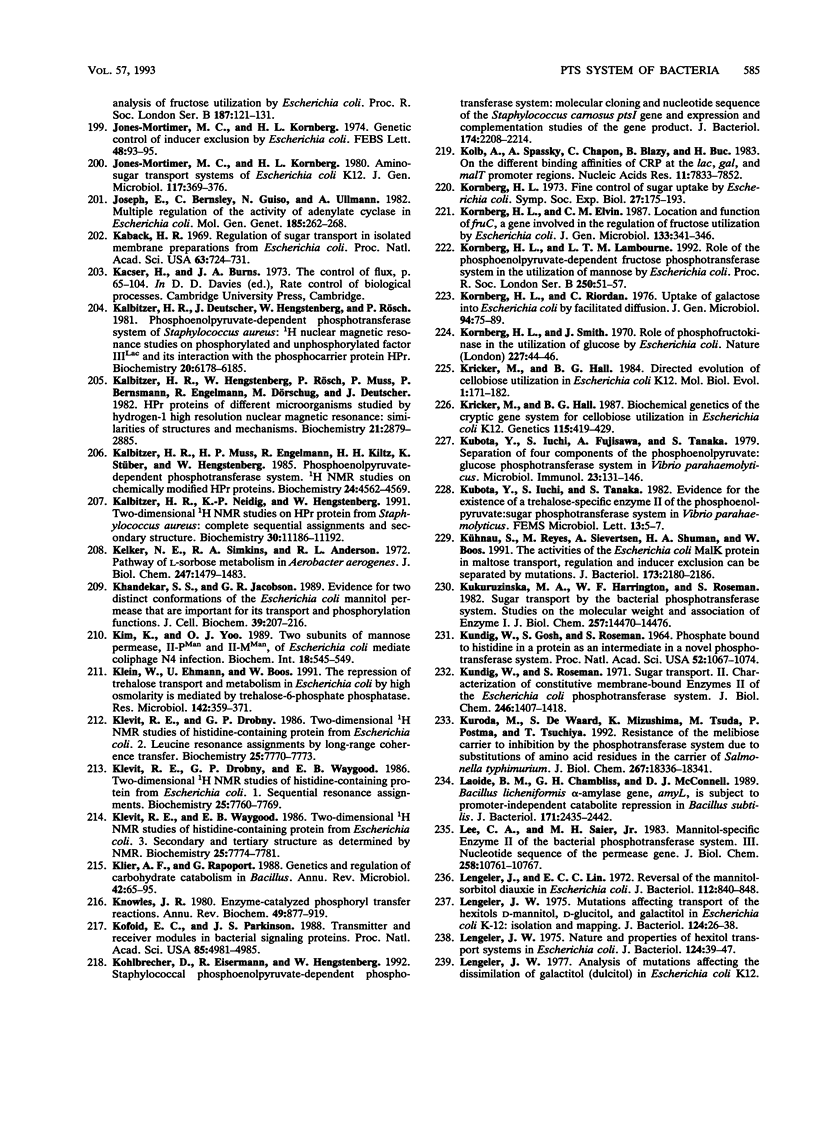
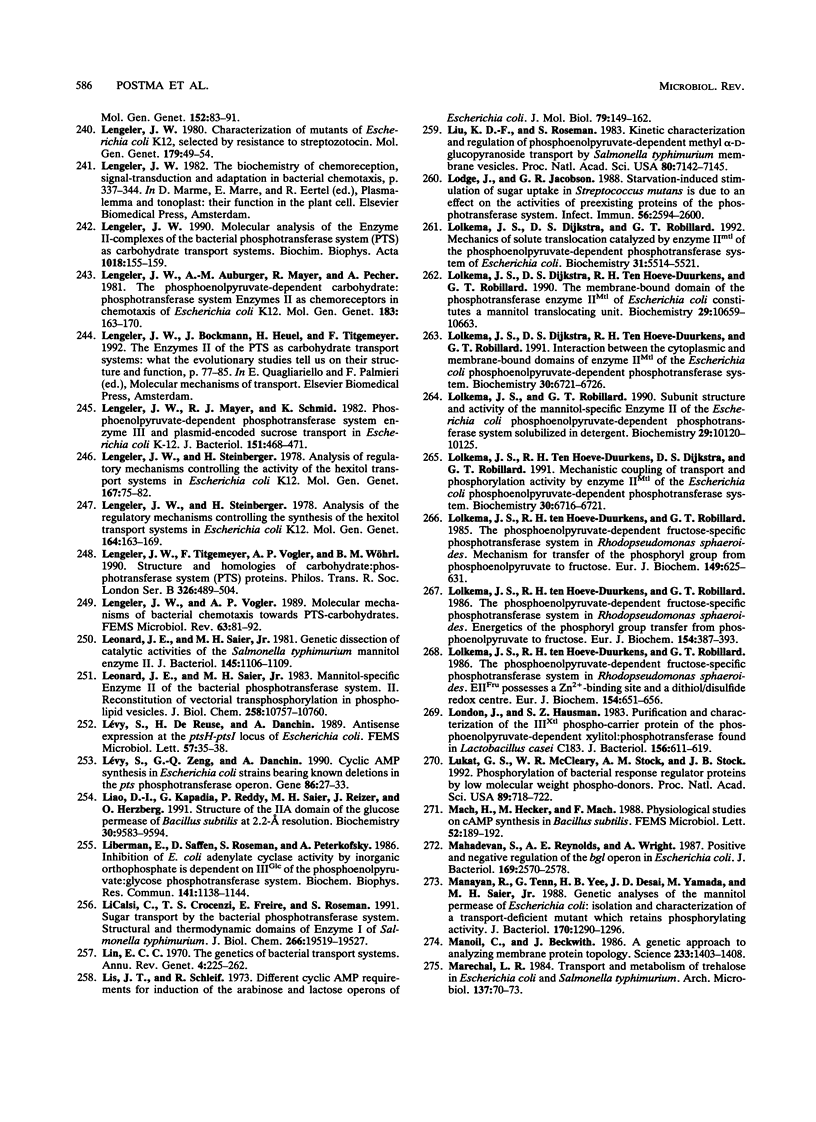
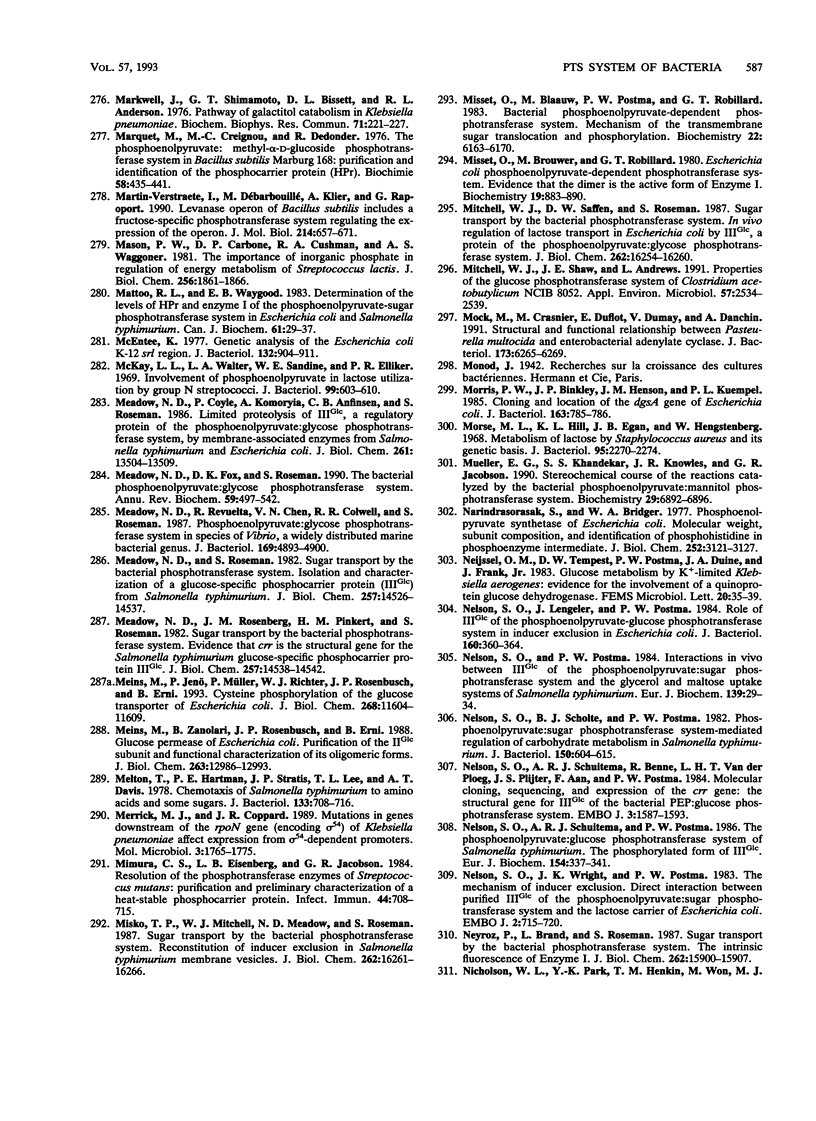
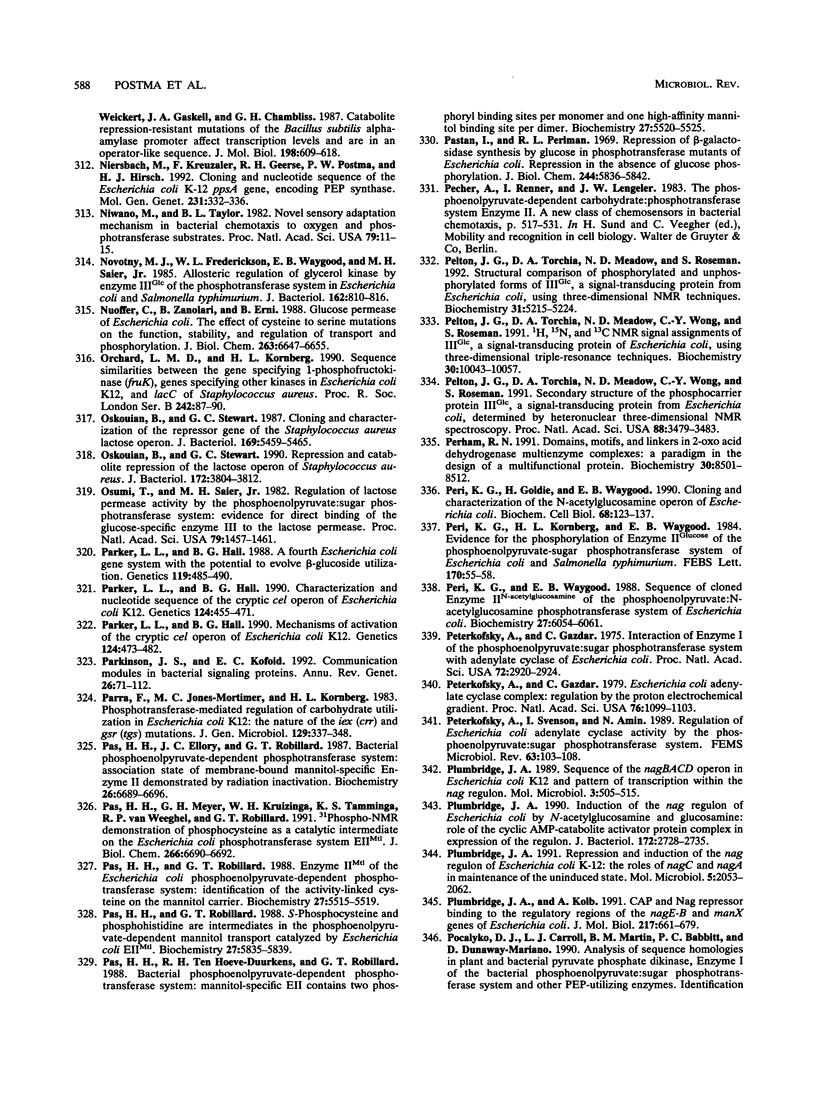
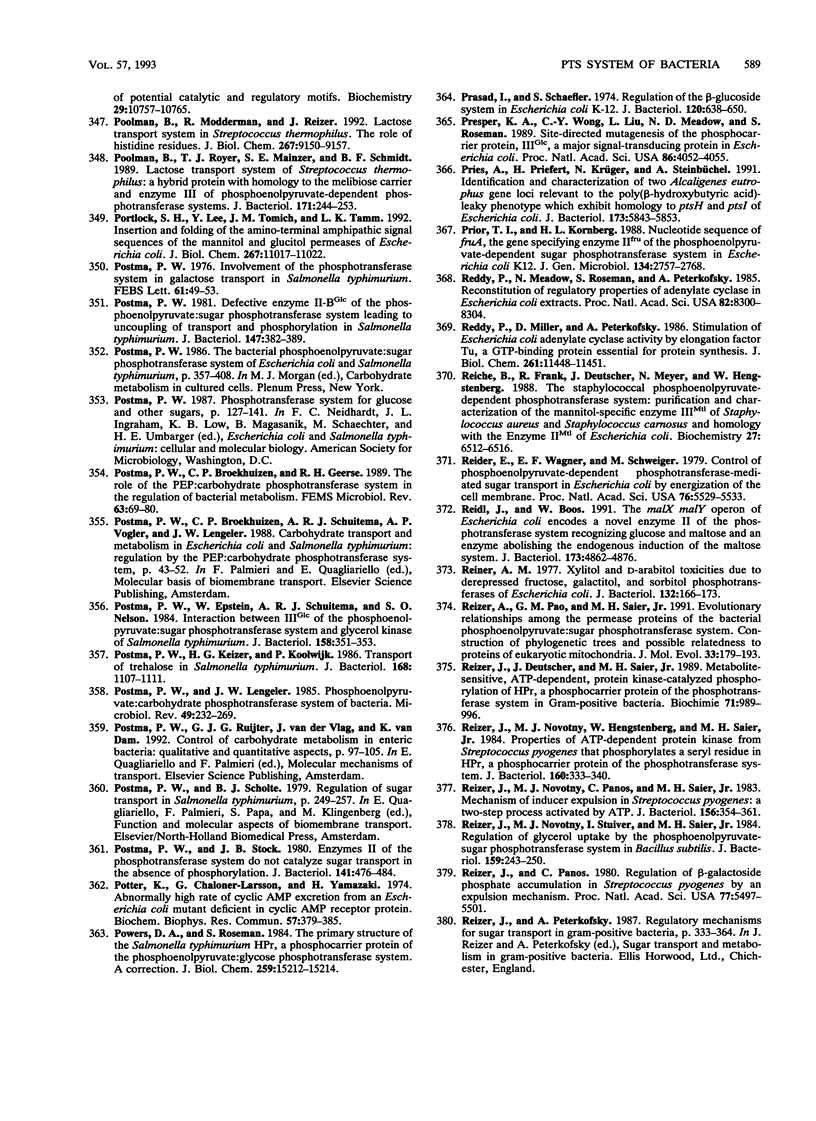
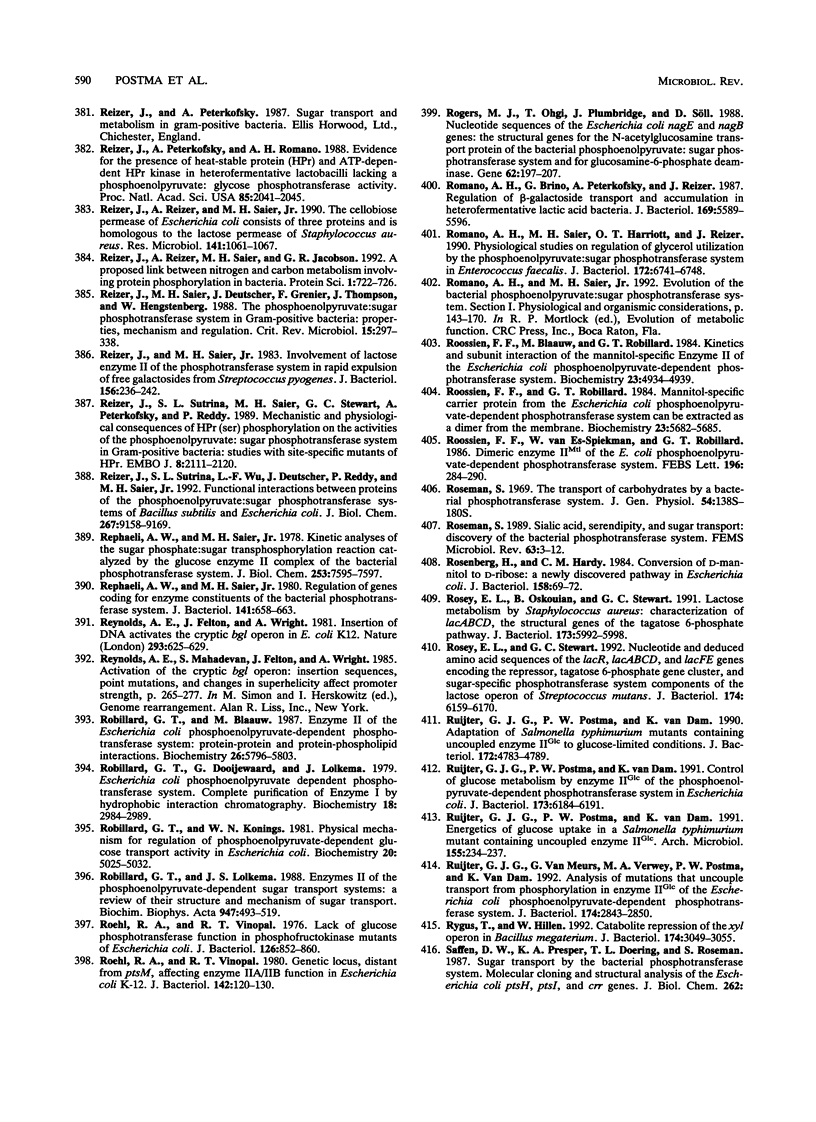
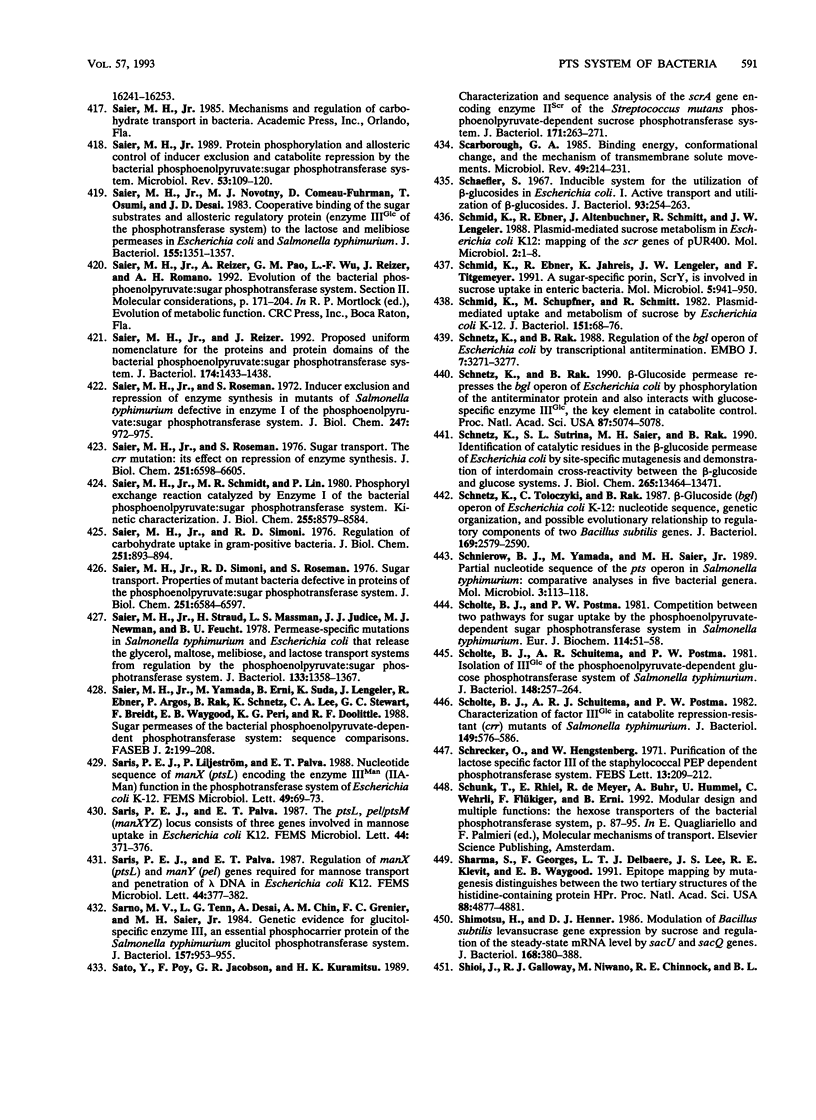
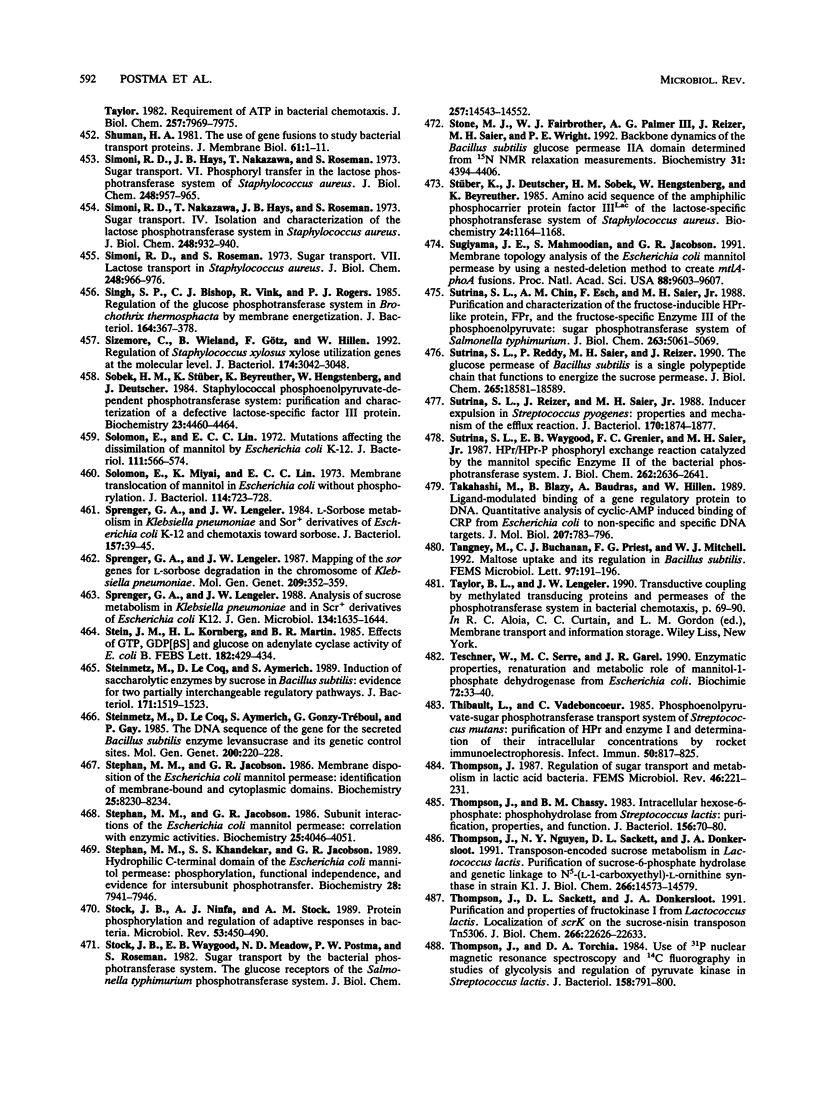
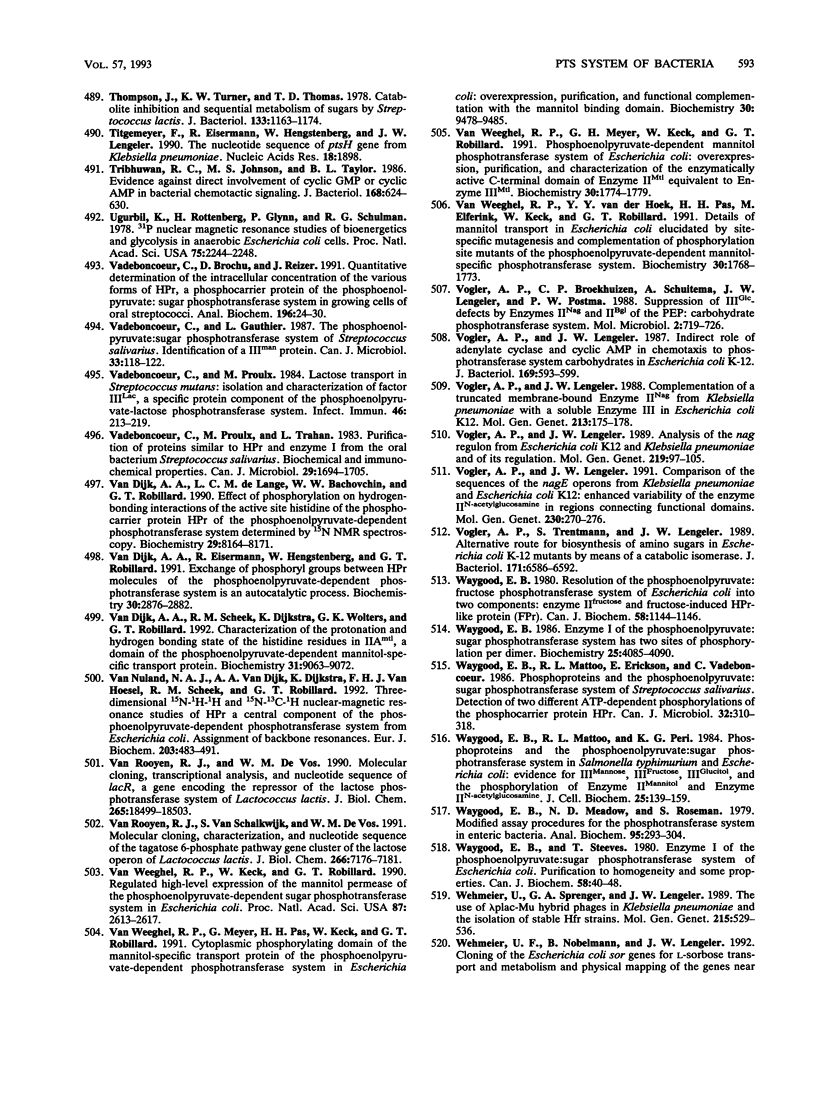
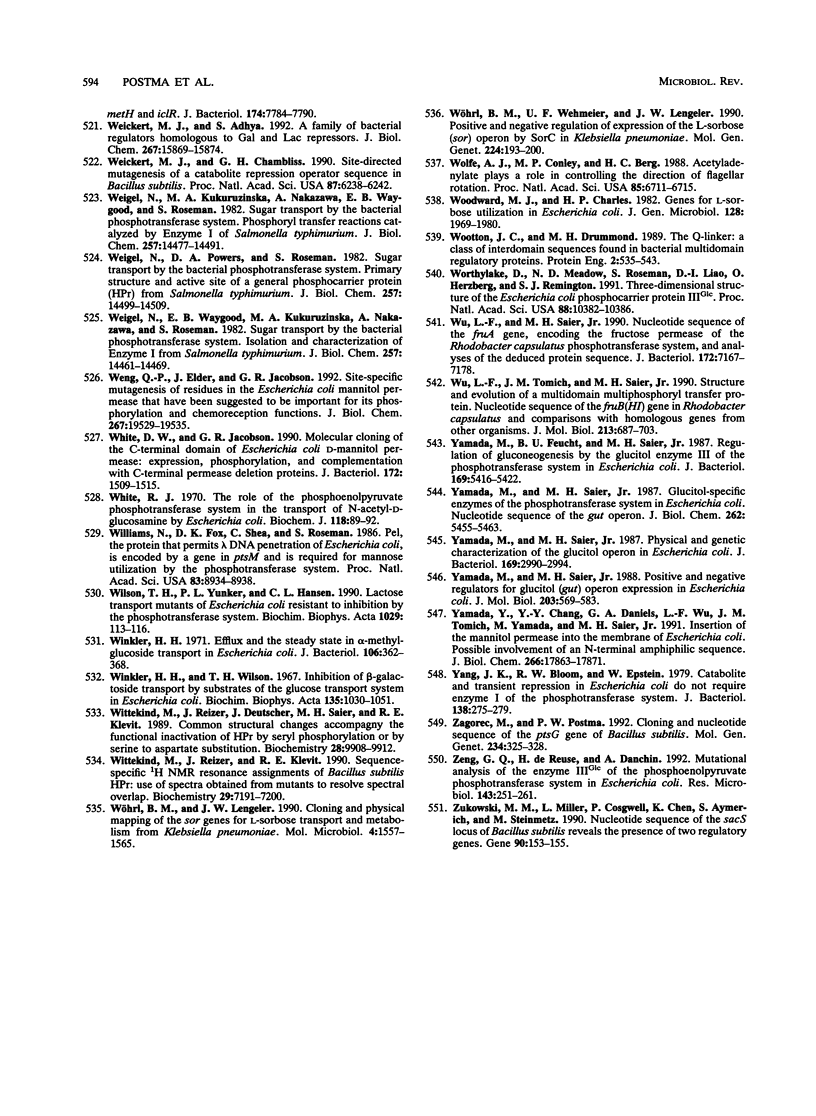
Selected References
These references are in PubMed. This may not be the complete list of references from this article.
- Adler J., Epstein W. Phosphotransferase-system enzymes as chemoreceptors for certain sugars in Escherichia coli chemotaxis. Proc Natl Acad Sci U S A. 1974 Jul;71(7):2895–2899. doi: 10.1073/pnas.71.7.2895. [DOI] [PMC free article] [PubMed] [Google Scholar]
- Alpert C. A., Chassy B. M. Molecular cloning and DNA sequence of lacE, the gene encoding the lactose-specific enzyme II of the phosphotransferase system of Lactobacillus casei. Evidence that a cysteine residue is essential for sugar phosphorylation. J Biol Chem. 1990 Dec 25;265(36):22561–22568. [PubMed] [Google Scholar]
- Alpert C. A., Chassy B. M. Molecular cloning and nucleotide sequence of the factor IIIlac gene of Lactobacillus casei. Gene. 1988;62(2):277–288. doi: 10.1016/0378-1119(88)90565-3. [DOI] [PubMed] [Google Scholar]
- Alpert C. A., Dörschug M., Saffen D., Frank R., Deutscher J., Hengstenberg W. The bacterial phosphoenolpyruvate-dependent phosphotransferase system. Isolation of active site peptides by reversed-phase high-performance liquid chromatography and determination of their primary structure. J Chromatogr. 1985 Jun 19;326:363–371. doi: 10.1016/s0021-9673(01)87462-8. [DOI] [PubMed] [Google Scholar]
- Alpert C. A., Frank R., Stüber K., Deutscher J., Hengstenberg W. Phosphoenolpyruvate-dependent protein kinase enzyme I of Streptococcus faecalis: purification and properties of the enzyme and characterization of its active center. Biochemistry. 1985 Feb 12;24(4):959–964. doi: 10.1021/bi00325a023. [DOI] [PubMed] [Google Scholar]
- Amaral D., Kornberg H. L. Regulation of fructose uptake by glucose in Escherichia coli. J Gen Microbiol. 1975 Sep;90(1):157–168. doi: 10.1099/00221287-90-1-157. [DOI] [PubMed] [Google Scholar]
- Amster-Choder O., Houman F., Wright A. Protein phosphorylation regulates transcription of the beta-glucoside utilization operon in E. coli. Cell. 1989 Sep 8;58(5):847–855. doi: 10.1016/0092-8674(89)90937-9. [DOI] [PubMed] [Google Scholar]
- Amster-Choder O., Wright A. Modulation of the dimerization of a transcriptional antiterminator protein by phosphorylation. Science. 1992 Sep 4;257(5075):1395–1398. doi: 10.1126/science.1382312. [DOI] [PubMed] [Google Scholar]
- Anderson B., Weigel N., Kundig W., Roseman S. Sugar transport. 3. Purification and properties of a phosphocarrier protein (HPr) of the phosphoenolpyruvate-dependent phosphotransferase system of Escherichia coli. J Biol Chem. 1971 Nov 25;246(22):7023–7033. [PubMed] [Google Scholar]
- Anderson J. W., Bhanot P., Georges F., Klevit R. E., Waygood E. B. Involvement of the carboxy-terminal residue in the active site of the histidine-containing protein, HPr, of the phosphoenolpyruvate:sugar phosphotransferase system of Escherichia coli. Biochemistry. 1991 Oct 8;30(40):9601–9607. doi: 10.1021/bi00104a006. [DOI] [PubMed] [Google Scholar]
- Arnaud M., Vary P., Zagorec M., Klier A., Debarbouille M., Postma P., Rapoport G. Regulation of the sacPA operon of Bacillus subtilis: identification of phosphotransferase system components involved in SacT activity. J Bacteriol. 1992 May;174(10):3161–3170. doi: 10.1128/jb.174.10.3161-3170.1992. [DOI] [PMC free article] [PubMed] [Google Scholar]
- Aulkemeyer P., Ebner R., Heilenmann G., Jahreis K., Schmid K., Wrieden S., Lengeler J. W. Molecular analysis of two fructokinases involved in sucrose metabolism of enteric bacteria. Mol Microbiol. 1991 Dec;5(12):2913–2922. doi: 10.1111/j.1365-2958.1991.tb01851.x. [DOI] [PubMed] [Google Scholar]
- Bachmann B. J. Linkage map of Escherichia coli K-12, edition 8. Microbiol Rev. 1990 Jun;54(2):130–197. doi: 10.1128/mr.54.2.130-197.1990. [DOI] [PMC free article] [PubMed] [Google Scholar]
- Begley G. S., Hansen D. E., Jacobson G. R., Knowles J. R. Stereochemical course of the reactions catalyzed by the bacterial phosphoenolpyruvate:glucose phosphotransferase system. Biochemistry. 1982 Oct 26;21(22):5552–5556. doi: 10.1021/bi00265a026. [DOI] [PubMed] [Google Scholar]
- Beneski D. A., Nakazawa A., Weigel N., Hartman P. E., Roseman S. Sugar transport by the bacterial phosphotransferase system. Isolation and characterization of a phosphocarrier protein HPr from wild type and mutants of Salmonella typhimurium. J Biol Chem. 1982 Dec 10;257(23):14492–14498. [PubMed] [Google Scholar]
- Berman-Kurtz M., Lin E. C., Richey D. P. Promoter-like mutant with increased expression of the glycerol kinase operon of Escherichia coli. J Bacteriol. 1971 Jun;106(3):724–731. doi: 10.1128/jb.106.3.724-731.1971. [DOI] [PMC free article] [PubMed] [Google Scholar]
- Berman M., Lin E. C. Glycerol-specific revertants of a phosphoenolpyruvate phosphotransferase mutant: suppression by the desensitization of glycerol kinase to feedback inhibition. J Bacteriol. 1971 Jan;105(1):113–120. doi: 10.1128/jb.105.1.113-120.1971. [DOI] [PMC free article] [PubMed] [Google Scholar]
- Bernsmann P., Alpert C. A., Muss P., Deutscher J., Hengstenberg W. The bacterial PEP-dependent phosphotransferase system mechanism of gluconate phosphorylation in Streptococcus faecalis. FEBS Lett. 1982 Feb 8;138(1):101–103. doi: 10.1016/0014-5793(82)80404-3. [DOI] [PubMed] [Google Scholar]
- Bischoff D. S., Ordal G. W. Bacillus subtilis chemotaxis: a deviation from the Escherichia coli paradigm. Mol Microbiol. 1992 Jan;6(1):23–28. doi: 10.1111/j.1365-2958.1992.tb00833.x. [DOI] [PubMed] [Google Scholar]
- Black R. A., Hobson A. C., Adler J. Adenylate cyclase is required for chemotaxis to phosphotransferase system sugars by Escherichia coli. J Bacteriol. 1983 Mar;153(3):1187–1195. doi: 10.1128/jb.153.3.1187-1195.1983. [DOI] [PMC free article] [PubMed] [Google Scholar]
- Blatch G. L., Scholle R. R., Woods D. R. Nucleotide sequence and analysis of the Vibrio alginolyticus sucrose uptake-encoding region. Gene. 1990 Oct 30;95(1):17–23. doi: 10.1016/0378-1119(90)90408-j. [DOI] [PubMed] [Google Scholar]
- Bolshakova T. N., Molchanova M. L., Erlagaeva R. S., Grigorenko Y. A., Gershanovitch V. N. A novel mutation FruS, altering synthesis of components of the phosphoenolpyruvate: fructose phosphotransferase system in Escherichia coli K12. Mol Gen Genet. 1992 Apr;232(3):394–398. doi: 10.1007/BF00266242. [DOI] [PubMed] [Google Scholar]
- Boos W., Ehmann U., Bremer E., Middendorf A., Postma P. Trehalase of Escherichia coli. Mapping and cloning of its structural gene and identification of the enzyme as a periplasmic protein induced under high osmolarity growth conditions. J Biol Chem. 1987 Sep 25;262(27):13212–13218. [PubMed] [Google Scholar]
- Boos W., Ehmann U., Forkl H., Klein W., Rimmele M., Postma P. Trehalose transport and metabolism in Escherichia coli. J Bacteriol. 1990 Jun;172(6):3450–3461. doi: 10.1128/jb.172.6.3450-3461.1990. [DOI] [PMC free article] [PubMed] [Google Scholar]
- Botsford J. L., Harman J. G. Cyclic AMP in prokaryotes. Microbiol Rev. 1992 Mar;56(1):100–122. doi: 10.1128/mr.56.1.100-122.1992. [DOI] [PMC free article] [PubMed] [Google Scholar]
- Bouma C. L., Meadow N. D., Stover E. W., Roseman S. II-BGlc, a glucose receptor of the bacterial phosphotransferase system: molecular cloning of ptsG and purification of the receptor from an overproducing strain of Escherichia coli. Proc Natl Acad Sci U S A. 1987 Feb;84(4):930–934. doi: 10.1073/pnas.84.4.930. [DOI] [PMC free article] [PubMed] [Google Scholar]
- Bourret R. B., Borkovich K. A., Simon M. I. Signal transduction pathways involving protein phosphorylation in prokaryotes. Annu Rev Biochem. 1991;60:401–441. doi: 10.1146/annurev.bi.60.070191.002153. [DOI] [PubMed] [Google Scholar]
- Bramley H. F., Kornberg H. L. Nucleotide sequence of bglC, the gene specifying enzymeIIbgl of the PEP:sugar phosphotransferase system in Escherichia coli K12, and overexpression of the gene product. J Gen Microbiol. 1987 Mar;133(3):563–573. doi: 10.1099/00221287-133-3-563. [DOI] [PubMed] [Google Scholar]
- Bramley H. F., Kornberg H. L. Sequence homologies between proteins of bacterial phosphoenolpyruvate-dependent sugar phosphotransferase systems: identification of possible phosphate-carrying histidine residues. Proc Natl Acad Sci U S A. 1987 Jul;84(14):4777–4780. doi: 10.1073/pnas.84.14.4777. [DOI] [PMC free article] [PubMed] [Google Scholar]
- Breidt F., Jr, Hengstenberg W., Finkeldei U., Stewart G. C. Identification of the genes for the lactose-specific components of the phosphotransferase system in the lac operon of Staphylococcus aureus. J Biol Chem. 1987 Dec 5;262(34):16444–16449. [PubMed] [Google Scholar]
- Brice C. B., Kornberg H. L. Location of a gene specifying phosphopyruvate synthase activity on the genome of Escherichia coli, K12. Proc R Soc Lond B Biol Sci. 1967 Sep 12;168(1012):281–292. doi: 10.1098/rspb.1967.0066. [DOI] [PubMed] [Google Scholar]
- Briggs C. E., Khandekar S. S., Jacobson G. R. Structure/function relationships in the Escherichia coli mannitol permease: identification of regions important for membrane insertion, substrate binding and oligomerization. Res Microbiol. 1992 Feb;143(2):139–149. doi: 10.1016/0923-2508(92)90003-7. [DOI] [PubMed] [Google Scholar]
- Buhr A., Daniels G. A., Erni B. The glucose transporter of Escherichia coli. Mutants with impaired translocation activity that retain phosphorylation activity. J Biol Chem. 1992 Feb 25;267(6):3847–3851. [PubMed] [Google Scholar]
- Buhr A., Erni B. Membrane topology of the glucose transporter of Escherichia coli. J Biol Chem. 1993 Jun 5;268(16):11599–11603. [PubMed] [Google Scholar]
- Button D. K., Egan J. B., Hengstenberg W., Morse M. L. Carbohydrate transport in Staphylococcus aureus. IV. Maltose accumulation and metabolism. Biochem Biophys Res Commun. 1973 Jun 8;52(3):850–855. doi: 10.1016/0006-291x(73)91015-2. [DOI] [PubMed] [Google Scholar]
- Byrne C. R., Monroe R. S., Ward K. A., Kredich N. M. DNA sequences of the cysK regions of Salmonella typhimurium and Escherichia coli and linkage of the cysK regions to ptsH. J Bacteriol. 1988 Jul;170(7):3150–3157. doi: 10.1128/jb.170.7.3150-3157.1988. [DOI] [PMC free article] [PubMed] [Google Scholar]
- COHN M., HORIBATA K. Physiology of the inhibition by glucose of the induced synthesis of the beta-galactosideenzyme system of Escherichia coli. J Bacteriol. 1959 Nov;78:624–635. doi: 10.1128/jb.78.5.624-635.1959. [DOI] [PMC free article] [PubMed] [Google Scholar]
- Calvo J. M., Goodman M., Salgo M., Capes N. Salmonella locus affecting phosphoenolpyruvate synthase activity identified by a deletion analysis. J Bacteriol. 1971 Apr;106(1):286–288. doi: 10.1128/jb.106.1.286-288.1971. [DOI] [PMC free article] [PubMed] [Google Scholar]
- Castro L., Feucht B. U., Morse M. L., Saier M. H., Jr Regulation of carbohydrate permeases and adenylate cyclase in Escherichia coli. Studies with mutant strains in which enzyme I of the phosphoenolpyruvate:sugar phosphotransferase system is thermolabile. J Biol Chem. 1976 Sep 25;251(18):5522–5527. [PubMed] [Google Scholar]
- Celikel R., Dai X. P., Stewart G. C., Sutrina S. L., Saier M. H., Jr, Xuong N. H., Reizer J. Crystallization and preliminary X-ray analysis of the lactose-specific phosphocarrier protein IIAlac of the phosphoenolpyruvate: sugar phosphotransferase system from Staphylococcus aureus. J Mol Biol. 1991 Dec 20;222(4):857–859. doi: 10.1016/0022-2836(91)90576-r. [DOI] [PubMed] [Google Scholar]
- Chassy B. M., Alpert C. A. Molecular characterization of the plasmid-encoded lactose-PTS of Lactobacillus casei. FEMS Microbiol Rev. 1989 Jun;5(1-2):157–165. doi: 10.1016/0168-6445(89)90020-x. [DOI] [PubMed] [Google Scholar]
- Chassy B. M., Thompson J. Regulation and characterization of the galactose-phosphoenolpyruvate-dependent phosphotransferase system in Lactobacillus casei. J Bacteriol. 1983 Jun;154(3):1204–1214. doi: 10.1128/jb.154.3.1204-1214.1983. [DOI] [PMC free article] [PubMed] [Google Scholar]
- Chin A. M., Feldheim D. A., Saier M. H., Jr Altered transcriptional patterns affecting several metabolic pathways in strains of Salmonella typhimurium which overexpress the fructose regulon. J Bacteriol. 1989 May;171(5):2424–2434. doi: 10.1128/jb.171.5.2424-2434.1989. [DOI] [PMC free article] [PubMed] [Google Scholar]
- Chin A. M., Feucht B. U., Saier M. H., Jr Evidence for regulation of gluconeogenesis by the fructose phosphotransferase system in Salmonella typhimurium. J Bacteriol. 1987 Feb;169(2):897–899. doi: 10.1128/jb.169.2.897-899.1987. [DOI] [PMC free article] [PubMed] [Google Scholar]
- Chin A. M., Sutrina S., Feldheim D. A., Saier M. H., Jr Genetic expression of enzyme I activity of the phosphoenolpyruvate:sugar phosphotransferase system in ptsHI deletion strains of Salmonella typhimurium. J Bacteriol. 1987 Feb;169(2):894–896. doi: 10.1128/jb.169.2.894-896.1987. [DOI] [PMC free article] [PubMed] [Google Scholar]
- Clark B., Holms W. H. Control of the sequential utilization of glucose and fructose by Escherichia coli. J Gen Microbiol. 1976 Aug;96(2):191–201. doi: 10.1099/00221287-95-2-191. [DOI] [PubMed] [Google Scholar]
- Cooper R. A., Kornberg H. L. The direct synthesis of phosphoenolpyruvate from pyruvate by Escherichia coli. Proc R Soc Lond B Biol Sci. 1967 Sep 12;168(1012):263–280. doi: 10.1098/rspb.1967.0065. [DOI] [PubMed] [Google Scholar]
- Cordaro C. Genetics of the bacterial phosphoenolpyruvate: glycose phosphotransferase system. Annu Rev Genet. 1976;10:341–359. doi: 10.1146/annurev.ge.10.120176.002013. [DOI] [PubMed] [Google Scholar]
- Cordaro J. C., Roseman S. Deletion mapping of the genes coding for HPr and enzyme I of the phosphoenolpyruvate: sugar phosphotransferase system in Salmonella typhimurium. J Bacteriol. 1972 Oct;112(1):17–29. doi: 10.1128/jb.112.1.17-29.1972. [DOI] [PMC free article] [PubMed] [Google Scholar]
- Cowan P. J., Nagesha H., Leonard L., Howard J. L., Pittard A. J. Characterization of the major promoter for the plasmid-encoded sucrose genes scrY, scrA, and scrB. J Bacteriol. 1991 Dec;173(23):7464–7470. doi: 10.1128/jb.173.23.7464-7470.1991. [DOI] [PMC free article] [PubMed] [Google Scholar]
- Crasnier M., Danchin A. Characterization of Escherichia coli adenylate cyclase mutants with modified regulation. J Gen Microbiol. 1990 Sep;136(9):1825–1831. doi: 10.1099/00221287-136-9-1825. [DOI] [PubMed] [Google Scholar]
- Crutz A. M., Steinmetz M., Aymerich S., Richter R., Le Coq D. Induction of levansucrase in Bacillus subtilis: an antitermination mechanism negatively controlled by the phosphotransferase system. J Bacteriol. 1990 Feb;172(2):1043–1050. doi: 10.1128/jb.172.2.1043-1050.1990. [DOI] [PMC free article] [PubMed] [Google Scholar]
- Crutz A. M., Steinmetz M. Transcription of the Bacillus subtilis sacX and sacY genes, encoding regulators of sucrose metabolism, is both inducible by sucrose and controlled by the DegS-DegU signalling system. J Bacteriol. 1992 Oct;174(19):6087–6095. doi: 10.1128/jb.174.19.6087-6095.1992. [DOI] [PMC free article] [PubMed] [Google Scholar]
- Csonka L. N., Clark A. J. Deletions generated by the transposon Tn10 in the srl recA region of the Escherichia coli K-12 chromosome. Genetics. 1979 Oct;93(2):321–343. doi: 10.1093/genetics/93.2.321. [DOI] [PMC free article] [PubMed] [Google Scholar]
- Curtis S. J., Epstein W. Phosphorylation of D-glucose in Escherichia coli mutants defective in glucosephosphotransferase, mannosephosphotransferase, and glucokinase. J Bacteriol. 1975 Jun;122(3):1189–1199. doi: 10.1128/jb.122.3.1189-1199.1975. [DOI] [PMC free article] [PubMed] [Google Scholar]
- Dannelly H. K., Roseman S. NAD+ and NADH regulate an ATP-dependent kinase that phosphorylates enzyme I of the Escherichia coli phosphotransferase system. Proc Natl Acad Sci U S A. 1992 Dec 1;89(23):11274–11276. doi: 10.1073/pnas.89.23.11274. [DOI] [PMC free article] [PubMed] [Google Scholar]
- Davis T., Yamada M., Elgort M., Saier M. H., Jr Nucleotide sequence of the mannitol (mtl) operon in Escherichia coli. Mol Microbiol. 1988 May;2(3):405–412. doi: 10.1111/j.1365-2958.1988.tb00045.x. [DOI] [PubMed] [Google Scholar]
- De Reuse H., Danchin A. Positive regulation of the pts operon of Escherichia coli: genetic evidence for a signal transduction mechanism. J Bacteriol. 1991 Jan;173(2):727–733. doi: 10.1128/jb.173.2.727-733.1991. [DOI] [PMC free article] [PubMed] [Google Scholar]
- De Reuse H., Danchin A. The ptsH, ptsI, and crr genes of the Escherichia coli phosphoenolpyruvate-dependent phosphotransferase system: a complex operon with several modes of transcription. J Bacteriol. 1988 Sep;170(9):3827–3837. doi: 10.1128/jb.170.9.3827-3837.1988. [DOI] [PMC free article] [PubMed] [Google Scholar]
- De Reuse H., Kolb A., Danchin A. Positive regulation of the expression of the Escherichia coli pts operon. Identification of the regulatory regions. J Mol Biol. 1992 Aug 5;226(3):623–635. doi: 10.1016/0022-2836(92)90620-y. [DOI] [PubMed] [Google Scholar]
- De Reuse H., Roy A., Danchin A. Analysis of the ptsH-ptsI-crr region in Escherichia coli K-12: nucleotide sequence of the ptsH gene. Gene. 1985;35(1-2):199–207. doi: 10.1016/0378-1119(85)90172-6. [DOI] [PubMed] [Google Scholar]
- Dean D. A., Reizer J., Nikaido H., Saier M. H., Jr Regulation of the maltose transport system of Escherichia coli by the glucose-specific enzyme III of the phosphoenolpyruvate-sugar phosphotransferase system. Characterization of inducer exclusion-resistant mutants and reconstitution of inducer exclusion in proteoliposomes. J Biol Chem. 1990 Dec 5;265(34):21005–21010. [PubMed] [Google Scholar]
- Debarbouille M., Arnaud M., Fouet A., Klier A., Rapoport G. The sacT gene regulating the sacPA operon in Bacillus subtilis shares strong homology with transcriptional antiterminators. J Bacteriol. 1990 Jul;172(7):3966–3973. doi: 10.1128/jb.172.7.3966-3973.1990. [DOI] [PMC free article] [PubMed] [Google Scholar]
- Delidakis C. E., Jones-Mortimer M. C., Kornberg H. L. A mutant inducible for galactitol utilization in Escherichia coli K12. J Gen Microbiol. 1982 Mar;128(3):601–604. doi: 10.1099/00221287-128-3-601. [DOI] [PubMed] [Google Scholar]
- Deutscher J., Beyreuther K., Sobek H. M., Stüber K., Hengstenberg W. Phosphoenolpyruvate-dependent phosphotransferase system of Staphylococcus aureus: factor IIIlac, a trimeric phospho-carrier protein that also acts as a phase transfer catalyst. Biochemistry. 1982 Sep 28;21(20):4867–4873. doi: 10.1021/bi00263a006. [DOI] [PubMed] [Google Scholar]
- Deutscher J., Kessler U., Hengstenberg W. Streptococcal phosphoenolpyruvate: sugar phosphotransferase system: purification and characterization of a phosphoprotein phosphatase which hydrolyzes the phosphoryl bond in seryl-phosphorylated histidine-containing protein. J Bacteriol. 1985 Sep;163(3):1203–1209. doi: 10.1128/jb.163.3.1203-1209.1985. [DOI] [PMC free article] [PubMed] [Google Scholar]
- Deutscher J., Pevec B., Beyreuther K., Kiltz H. H., Hengstenberg W. Streptococcal phosphoenolpyruvate-sugar phosphotransferase system: amino acid sequence and site of ATP-dependent phosphorylation of HPr. Biochemistry. 1986 Oct 21;25(21):6543–6551. doi: 10.1021/bi00369a031. [DOI] [PubMed] [Google Scholar]
- Deutscher J., Saier M. H., Jr ATP-dependent protein kinase-catalyzed phosphorylation of a seryl residue in HPr, a phosphate carrier protein of the phosphotransferase system in Streptococcus pyogenes. Proc Natl Acad Sci U S A. 1983 Nov;80(22):6790–6794. doi: 10.1073/pnas.80.22.6790. [DOI] [PMC free article] [PubMed] [Google Scholar]
- Deutscher J., Sauerwald H. Stimulation of dihydroxyacetone and glycerol kinase activity in Streptococcus faecalis by phosphoenolpyruvate-dependent phosphorylation catalyzed by enzyme I and HPr of the phosphotransferase system. J Bacteriol. 1986 Jun;166(3):829–836. doi: 10.1128/jb.166.3.829-836.1986. [DOI] [PMC free article] [PubMed] [Google Scholar]
- Dills S. S., Apperson A., Schmidt M. R., Saier M. H., Jr Carbohydrate transport in bacteria. Microbiol Rev. 1980 Sep;44(3):385–418. doi: 10.1128/mr.44.3.385-418.1980. [DOI] [PMC free article] [PubMed] [Google Scholar]
- Dills S. S., Seno S. Regulation of hexitol catabolism in Streptococcus mutans. J Bacteriol. 1983 Feb;153(2):861–866. doi: 10.1128/jb.153.2.861-866.1983. [DOI] [PMC free article] [PubMed] [Google Scholar]
- Dooijewaard G., Roossien F. F., Robillard G. T. Escherichia coli phosphoenolpyruvate dependent phosphotransferase system. Copurification of HPr and alpha 1-6 glucan. Biochemistry. 1979 Jul 10;18(14):2990–2996. doi: 10.1021/bi00581a013. [DOI] [PubMed] [Google Scholar]
- Débarbouillé M., Martin-Verstraete I., Arnaud M., Klier A., Rapoport G. Positive and negative regulation controlling expression of the sac genes in Bacillus subtilis. Res Microbiol. 1991 Sep-Oct;142(7-8):757–764. doi: 10.1016/0923-2508(91)90052-c. [DOI] [PubMed] [Google Scholar]
- Débarbouillé M., Martin-Verstraete I., Klier A., Rapoport G. The transcriptional regulator LevR of Bacillus subtilis has domains homologous to both sigma 54- and phosphotransferase system-dependent regulators. Proc Natl Acad Sci U S A. 1991 Mar 15;88(6):2212–2216. doi: 10.1073/pnas.88.6.2212. [DOI] [PMC free article] [PubMed] [Google Scholar]
- Débarbouillé M., Martin-Verstraete I., Kunst F., Rapoport G. The Bacillus subtilis sigL gene encodes an equivalent of sigma 54 from gram-negative bacteria. Proc Natl Acad Sci U S A. 1991 Oct 15;88(20):9092–9096. doi: 10.1073/pnas.88.20.9092. [DOI] [PMC free article] [PubMed] [Google Scholar]
- Dörschug M., Frank R., Kalbitzer H. R., Hengstenberg W., Deutscher J. Phosphoenolpyruvate-dependent phosphorylation site in enzyme IIIglc of the Escherichia coli phosphotransferase system. Eur J Biochem. 1984 Oct 1;144(1):113–119. doi: 10.1111/j.1432-1033.1984.tb08438.x. [DOI] [PubMed] [Google Scholar]
- ENGLESBERG E., WATSON J. A., HOFFEE P. A. The glucose effect and the relationship between glucose permease, acid phosphatase, and glucose resistance. Cold Spring Harb Symp Quant Biol. 1961;26:261–276. doi: 10.1101/sqb.1961.026.01.033. [DOI] [PubMed] [Google Scholar]
- Ebner R., Lengeler J. W. DNA sequence of the gene scrA encoding the sucrose transport protein EnzymeII(Scr) of the phosphotransferase system from enteric bacteria: homology of the EnzymeII(Scr) and EnzymeII(Bgl) proteins. Mol Microbiol. 1988 Jan;2(1):9–17. [PubMed] [Google Scholar]
- Eisermann R., Deutscher J., Gonzy-Treboul G., Hengstenberg W. Site-directed mutagenesis with the ptsH gene of Bacillus subtilis. Isolation and characterization of heat-stable proteins altered at the ATP-dependent regulatory phosphorylation site. J Biol Chem. 1988 Nov 15;263(32):17050–17054. [PubMed] [Google Scholar]
- Elferink M. G., Driessen A. J., Robillard G. T. Functional reconstitution of the purified phosphoenolpyruvate-dependent mannitol-specific transport system of Escherichia coli in phospholipid vesicles: coupling between transport and phosphorylation. J Bacteriol. 1990 Dec;172(12):7119–7125. doi: 10.1128/jb.172.12.7119-7125.1990. [DOI] [PMC free article] [PubMed] [Google Scholar]
- Elliott J., Arber W. E. coli K-12 pel mutants, which block phage lambda DNA injection, coincide with ptsM, which determines a component of a sugar transport system. Mol Gen Genet. 1978 Apr 25;161(1):1–8. doi: 10.1007/BF00266608. [DOI] [PubMed] [Google Scholar]
- Elvin C. M., Kornberg H. L. A mutant beta-D-glucoside transport system of Escherichia coli resistant to catabolite inhibition. FEBS Lett. 1982 Oct 18;147(2):137–142. doi: 10.1016/0014-5793(82)81027-2. [DOI] [PubMed] [Google Scholar]
- Epstein W., Rothman-Denes L. B., Hesse J. Adenosine 3':5'-cyclic monophosphate as mediator of catabolite repression in Escherichia coli. Proc Natl Acad Sci U S A. 1975 Jun;72(6):2300–2304. doi: 10.1073/pnas.72.6.2300. [DOI] [PMC free article] [PubMed] [Google Scholar]
- Erni B. Coupling of energy to glucose transport by the bacterial phosphotransferase system. Res Microbiol. 1990 Mar-Apr;141(3):360–364. doi: 10.1016/0923-2508(90)90012-f. [DOI] [PubMed] [Google Scholar]
- Erni B. Glucose transport in Escherichia coli. FEMS Microbiol Rev. 1989 Jun;5(1-2):13–23. doi: 10.1016/0168-6445(89)90004-1. [DOI] [PubMed] [Google Scholar]
- Erni B. Glucose-specific permease of the bacterial phosphotransferase system: phosphorylation and oligomeric structure of the glucose-specific IIGlc-IIIGlc complex of Salmonella typhimurium. Biochemistry. 1986 Jan 28;25(2):305–312. doi: 10.1021/bi00350a004. [DOI] [PubMed] [Google Scholar]
- Erni B. Group translocation of glucose and other carbohydrates by the bacterial phosphotransferase system. Int Rev Cytol. 1992;137:127–148. doi: 10.1016/s0074-7696(08)62675-3. [DOI] [PubMed] [Google Scholar]
- Erni B., Trachsel H., Postma P. W., Rosenbusch J. P. Bacterial phosphotransferase system. Solubilization and purification of the glucose-specific enzyme II from membranes of Salmonella typhimurium. J Biol Chem. 1982 Nov 25;257(22):13726–13730. [PubMed] [Google Scholar]
- Erni B., Zanolari B. Glucose-permease of the bacterial phosphotransferase system. Gene cloning, overproduction, and amino acid sequence of enzyme IIGlc. J Biol Chem. 1986 Dec 15;261(35):16398–16403. [PubMed] [Google Scholar]
- Erni B., Zanolari B., Graff P., Kocher H. P. Mannose permease of Escherichia coli. Domain structure and function of the phosphorylating subunit. J Biol Chem. 1989 Nov 5;264(31):18733–18741. [PubMed] [Google Scholar]
- Erni B., Zanolari B., Kocher H. P. The mannose permease of Escherichia coli consists of three different proteins. Amino acid sequence and function in sugar transport, sugar phosphorylation, and penetration of phage lambda DNA. J Biol Chem. 1987 Apr 15;262(11):5238–5247. [PubMed] [Google Scholar]
- Erni B., Zanolari B. The mannose-permease of the bacterial phosphotransferase system. Gene cloning and purification of the enzyme IIMan/IIIMan complex of Escherichia coli. J Biol Chem. 1985 Dec 15;260(29):15495–15503. [PubMed] [Google Scholar]
- Fairbrother W. J., Cavanagh J., Dyson H. J., Palmer A. G., 3rd, Sutrina S. L., Reizer J., Saier M. H., Jr, Wright P. E. Polypeptide backbone resonance assignments and secondary structure of Bacillus subtilis enzyme IIIglc determined by two-dimensional and three-dimensional heteronuclear NMR spectroscopy. Biochemistry. 1991 Jul 16;30(28):6896–6907. doi: 10.1021/bi00242a013. [DOI] [PubMed] [Google Scholar]
- Fairbrother W. J., Gippert G. P., Reizer J., Saier M. H., Jr, Wright P. E. Low resolution solution structure of the Bacillus subtilis glucose permease IIA domain derived from heteronuclear three-dimensional NMR spectroscopy. FEBS Lett. 1992 Jan 20;296(2):148–152. doi: 10.1016/0014-5793(92)80367-p. [DOI] [PubMed] [Google Scholar]
- Fairbrother W. J., Palmer A. G., 3rd, Rance M., Reizer J., Saier M. H., Jr, Wright P. E. Assignment of the aliphatic 1H and 13C resonances of the Bacillus subtilis glucose permease IIA domain using double- and triple-resonance heteronuclear three-dimensional NMR spectroscopy. Biochemistry. 1992 May 12;31(18):4413–4425. doi: 10.1021/bi00133a005. [DOI] [PubMed] [Google Scholar]
- Fandl J. P., Thorner L. K., Artz S. W. Mutations that affect transcription and cyclic AMP-CRP regulation of the adenylate cyclase gene (cya) of Salmonella typhimurium. Genetics. 1990 Aug;125(4):719–727. doi: 10.1093/genetics/125.4.719. [DOI] [PMC free article] [PubMed] [Google Scholar]
- Feldheim D. A., Chin A. M., Nierva C. T., Feucht B. U., Cao Y. W., Xu Y. F., Sutrina S. L., Saier M. H., Jr Physiological consequences of the complete loss of phosphoryl-transfer proteins HPr and FPr of the phosphoenolpyruvate:sugar phosphotransferase system and analysis of fructose (fru) operon expression in Salmonella typhimurium. J Bacteriol. 1990 Sep;172(9):5459–5469. doi: 10.1128/jb.172.9.5459-5469.1990. [DOI] [PMC free article] [PubMed] [Google Scholar]
- Ferenci T., Kornberg H. L. The utilization of fructose by Escherichia coli. Properties of a mutant defective in fructose 1-phosphate kinase activity. Biochem J. 1973 Feb;132(2):341–347. doi: 10.1042/bj1320341. [DOI] [PMC free article] [PubMed] [Google Scholar]
- Feucht B. U., Saier M. H., Jr Fine control of adenylate cyclase by the phosphoenolpyruvate:sugar phosphotransferase systems in Escherichia coli and Salmonella typhimurium. J Bacteriol. 1980 Feb;141(2):603–610. doi: 10.1128/jb.141.2.603-610.1980. [DOI] [PMC free article] [PubMed] [Google Scholar]
- Finkeldei U., Hengstenberg W. Staphylococcal lactose phosphoenolpyruvate-dependent phosphotransferase system: site-specific mutagenesis on the lacE gene gives evidence that a cysteine residue is responsible for phosphorylation. Protein Eng. 1991 Apr;4(4):475–478. doi: 10.1093/protein/4.4.475. [DOI] [PubMed] [Google Scholar]
- Finkeldei U., Kalbitzer H. R., Eisermann R., Stewart G. C., Hengstenberg W. Enzyme IIIlac of the staphylococcal phosphoenolpyruvate-dependent phosphotransferase system: site-specific mutagenesis of histidine residues, biochemical characterization and 1H-NMR studies. Protein Eng. 1991 Apr;4(4):469–473. doi: 10.1093/protein/4.4.469. [DOI] [PubMed] [Google Scholar]
- Fischer R., Eisermann R., Reiche B., Hengstenberg W. Cloning, sequencing and overexpression of the mannitol-specific enzyme-III-encoding gene of Staphylococcus carnosus. Gene. 1989 Oct 30;82(2):249–257. doi: 10.1016/0378-1119(89)90050-4. [DOI] [PubMed] [Google Scholar]
- Fischer R., Hengstenberg W. Mannitol-specific enzyme II of the phosphoenolpyruvate-dependent phosphotransferase system of Staphylococcus carnosus. Sequence and expression in Escherichia coli and structural comparison with the enzyme IImannitol of Escherichia coli. Eur J Biochem. 1992 Mar 15;204(3):963–969. doi: 10.1111/j.1432-1033.1992.tb16717.x. [DOI] [PubMed] [Google Scholar]
- Fischer R., von Strandmann R. P., Hengstenberg W. Mannitol-specific phosphoenolpyruvate-dependent phosphotransferase system of Enterococcus faecalis: molecular cloning and nucleotide sequences of the enzyme IIIMtl gene and the mannitol-1-phosphate dehydrogenase gene, expression in Escherichia coli, and comparison of the gene products with similar enzymes. J Bacteriol. 1991 Jun;173(12):3709–3715. doi: 10.1128/jb.173.12.3709-3715.1991. [DOI] [PMC free article] [PubMed] [Google Scholar]
- Fouet A., Arnaud M., Klier A., Rapoport G. Bacillus subtilis sucrose-specific enzyme II of the phosphotransferase system: expression in Escherichia coli and homology to enzymes II from enteric bacteria. Proc Natl Acad Sci U S A. 1987 Dec;84(24):8773–8777. doi: 10.1073/pnas.84.24.8773. [DOI] [PMC free article] [PubMed] [Google Scholar]
- Fouet A., Arnaud M., Klier A., Rapoport G. Genetics of the phosphotransferase system of Bacillus subtilis. FEMS Microbiol Rev. 1989 Jun;5(1-2):175–182. doi: 10.1016/0168-6445(89)90022-3. [DOI] [PubMed] [Google Scholar]
- Fouet A., Klier A., Rapoport G. Nucleotide sequence of the sucrase gene of Bacillus subtilis. Gene. 1986;45(2):221–225. doi: 10.1016/0378-1119(86)90258-1. [DOI] [PubMed] [Google Scholar]
- Fouet A., Sonenshein A. L. A target for carbon source-dependent negative regulation of the citB promoter of Bacillus subtilis. J Bacteriol. 1990 Feb;172(2):835–844. doi: 10.1128/jb.172.2.835-844.1990. [DOI] [PMC free article] [PubMed] [Google Scholar]
- Fox D. K., Meadow N. D., Roseman S. Phosphate transfer between acetate kinase and enzyme I of the bacterial phosphotransferase system. J Biol Chem. 1986 Oct 15;261(29):13498–13503. [PubMed] [Google Scholar]
- Fox D. K., Presper K. A., Adhya S., Roseman S., Garges S. Evidence for two promoters upstream of the pts operon: regulation by the cAMP receptor protein regulatory complex. Proc Natl Acad Sci U S A. 1992 Aug 1;89(15):7056–7059. doi: 10.1073/pnas.89.15.7056. [DOI] [PMC free article] [PubMed] [Google Scholar]
- Fraenkel D. G. The accumulation of glucose 6-phosphate from glucose and its effect in an Escherichia coli mutant lacking phosphoglucose isomerase and glucose 6-phosphate dehydrogenase. J Biol Chem. 1968 Dec 25;243(24):6451–6457. [PubMed] [Google Scholar]
- Fraenkel D. G. The phosphoenolpyruvate-initiated pathway of fructose metabolism in Escherichia coli. J Biol Chem. 1968 Dec 25;243(24):6458–6463. [PubMed] [Google Scholar]
- Friedman S. A., Hays J. B. Initial characterization of hexose and hexitol phosphoenolpyruvate-dependent phosphotransferases of Staphylococcus aureus. J Bacteriol. 1977 Jun;130(3):991–999. doi: 10.1128/jb.130.3.991-999.1977. [DOI] [PMC free article] [PubMed] [Google Scholar]
- Gagnon G., Vadeboncoeur C., Levesque R. C., Frenette M. Cloning, sequencing and expression in Escherichia coli of the ptsI gene encoding enzyme I of the phosphoenolpyruvate:sugar phosphotransferase transport system from Streptococcus salivarius. Gene. 1992 Nov 2;121(1):71–78. doi: 10.1016/0378-1119(92)90163-j. [DOI] [PubMed] [Google Scholar]
- García J. L. Cloning in Escherichia coli and molecular analysis of the sucrose system of the Salmonella plasmid SCR-53. Mol Gen Genet. 1985;201(3):575–577. doi: 10.1007/BF00331358. [DOI] [PubMed] [Google Scholar]
- Gauthier L., Mayrand D., Vadeboncoeur C. Isolation of a novel protein involved in the transport of fructose by an inducible phosphoenolpyruvate fructose phosphotransferase system in Streptococcus mutans. J Bacteriol. 1984 Nov;160(2):755–763. doi: 10.1128/jb.160.2.755-763.1984. [DOI] [PMC free article] [PubMed] [Google Scholar]
- Gay P., Cordier P., Marquet M., Delobbe A. Carbohydrate metabolism and transport in Bacillus subtilis. A study of ctr mutations. Mol Gen Genet. 1973 Mar 19;121(4):355–368. doi: 10.1007/BF00433234. [DOI] [PubMed] [Google Scholar]
- Geerse R. H., Izzo F., Postma P. W. The PEP: fructose phosphotransferase system in Salmonella typhimurium: FPr combines enzyme IIIFru and pseudo-HPr activities. Mol Gen Genet. 1989 Apr;216(2-3):517–525. doi: 10.1007/BF00334399. [DOI] [PubMed] [Google Scholar]
- Geerse R. H., Ruig C. R., Schuitema A. R., Postma P. W. Relationship between pseudo-HPr and the PEP: fructose phosphotransferase system in Salmonella typhimurium and Escherichia coli. Mol Gen Genet. 1986 Jun;203(3):435–444. doi: 10.1007/BF00422068. [DOI] [PubMed] [Google Scholar]
- Geerse R. H., van der Pluijm J., Postma P. W. The repressor of the PEP:fructose phosphotransferase system is required for the transcription of the pps gene of Escherichia coli. Mol Gen Genet. 1989 Aug;218(2):348–352. doi: 10.1007/BF00331288. [DOI] [PubMed] [Google Scholar]
- Gershanovitch V. N., Bolshakova T. N., Molchanova M. L., Umyarov A. M., Dobrynina OYu, Grigorenko YuA, Erlagaeva R. S. Fructose-specific phosphoenolpyruvate dependent phosphotransferase system of Escherichia coli: its alterations and adenylate cyclase activity. FEMS Microbiol Rev. 1989 Jun;5(1-2):125–133. doi: 10.1111/j.1574-6968.1989.tb14108.x. [DOI] [PubMed] [Google Scholar]
- Ghosh B. K., Owens K., Pietri R., Peterkofsky A. Localization to the inner surface of the cytoplasmic membrane by immunoelectron microscopy of enzyme I of the phosphoenolpyruvate:sugar phosphotransferase system of Escherichia coli. Proc Natl Acad Sci U S A. 1989 Feb;86(3):849–853. doi: 10.1073/pnas.86.3.849. [DOI] [PMC free article] [PubMed] [Google Scholar]
- Giaever H. M., Styrvold O. B., Kaasen I., Strøm A. R. Biochemical and genetic characterization of osmoregulatory trehalose synthesis in Escherichia coli. J Bacteriol. 1988 Jun;170(6):2841–2849. doi: 10.1128/jb.170.6.2841-2849.1988. [DOI] [PMC free article] [PubMed] [Google Scholar]
- Gonzy-Tréboul G., Steinmetz M. Phosphoenolpyruvate:sugar phosphotransferase system of Bacillus subtilis: cloning of the region containing the ptsH and ptsI genes and evidence for a crr-like gene. J Bacteriol. 1987 May;169(5):2287–2290. doi: 10.1128/jb.169.5.2287-2290.1987. [DOI] [PMC free article] [PubMed] [Google Scholar]
- Gonzy-Tréboul G., Zagorec M., Rain-Guion M. C., Steinmetz M. Phosphoenolpyruvate:sugar phosphotransferase system of Bacillus subtilis: nucleotide sequence of ptsX, ptsH and the 5'-end of ptsI and evidence for a ptsHI operon. Mol Microbiol. 1989 Jan;3(1):103–112. doi: 10.1111/j.1365-2958.1989.tb00109.x. [DOI] [PubMed] [Google Scholar]
- Gonzy-Tréboul G., de Waard J. H., Zagorec M., Postma P. W. The glucose permease of the phosphotransferase system of Bacillus subtilis: evidence for IIGlc and IIIGlc domains. Mol Microbiol. 1991 May;5(5):1241–1249. doi: 10.1111/j.1365-2958.1991.tb01898.x. [DOI] [PubMed] [Google Scholar]
- Grenier F. C., Hayward I., Novotny M. J., Leonard J. E., Saier M. H., Jr Identification of the phosphocarrier protein enzyme IIIgut: essential component of the glucitol phosphotransferase system in Salmonella typhimurium. J Bacteriol. 1985 Mar;161(3):1017–1022. doi: 10.1128/jb.161.3.1017-1022.1985. [DOI] [PMC free article] [PubMed] [Google Scholar]
- Grenier F. C., Waygood E. B., Saier M. H., Jr Bacterial phosphotransferase system: regulation of mannitol enzyme II activity by sulfhydryl oxidation. Biochemistry. 1985 Jan 1;24(1):47–51. doi: 10.1021/bi00322a008. [DOI] [PubMed] [Google Scholar]
- Grenier F. C., Waygood E. B., Saier M. H., Jr Bacterial phosphotransferase system: regulation of the glucose and mannose enzymes II by sulfhydryl oxidation. Biochemistry. 1985 Aug 27;24(18):4872–4876. doi: 10.1021/bi00339a022. [DOI] [PubMed] [Google Scholar]
- Grenier F. C., Waygood E. B., Saier M. H., Jr The bacterial phosphotransferase system: kinetic characterization of the glucose, mannitol, glucitol, and N-acetylglucosamine systems. J Cell Biochem. 1986;31(2):97–105. doi: 10.1002/jcb.240310203. [DOI] [PubMed] [Google Scholar]
- Grisafi P. L., Scholle A., Sugiyama J., Briggs C., Jacobson G. R., Lengeler J. W. Deletion mutants of the Escherichia coli K-12 mannitol permease: dissection of transport-phosphorylation, phospho-exchange, and mannitol-binding activities. J Bacteriol. 1989 May;171(5):2719–2727. doi: 10.1128/jb.171.5.2719-2727.1989. [DOI] [PMC free article] [PubMed] [Google Scholar]
- Grübl G., Vogler A. P., Lengeler J. W. Involvement of the histidine protein (HPr) of the phosphotransferase system in chemotactic signaling of Escherichia coli K-12. J Bacteriol. 1990 Oct;172(10):5871–5876. doi: 10.1128/jb.172.10.5871-5876.1990. [DOI] [PMC free article] [PubMed] [Google Scholar]
- Génovésio-Taverne J. C., Sauder U., Pauptit R. A., Jansonius J. N., Erni B. Crystallization and preliminary X-ray diffraction studies of the N-terminal domain of the phosphorylating subunit of mannose permease from Escherichia coli. J Mol Biol. 1990 Dec 5;216(3):515–517. doi: 10.1016/0022-2836(90)90379-Z. [DOI] [PubMed] [Google Scholar]
- HAGIHIRA H., WILSON T. H., LIN E. C. STUDIES ON THE GLUCOSE-TRANSPORT SYSTEM IN ESCHERICHIA COLI WITH ALPHA-METHYLGLUCOSIDE AS SUBSTRATE. Biochim Biophys Acta. 1963 Nov 15;78:505–515. doi: 10.1016/0006-3002(63)90912-0. [DOI] [PubMed] [Google Scholar]
- HOFFEE P., ENGLESBERG E. Effect of metabolic activity on the glucose permease of bacterial cells. Proc Natl Acad Sci U S A. 1962 Oct 15;48:1759–1765. doi: 10.1073/pnas.48.10.1759. [DOI] [PMC free article] [PubMed] [Google Scholar]
- HOFFEE P., ENGLESBERG E., LAMY F. THE GLUCOSE PERMEASE SYSTEM IN BACTERIA. Biochim Biophys Acta. 1964 Mar 30;79:337–350. [PubMed] [Google Scholar]
- Haguenauer-Tsapis R., Kepes A. Different sidedness of functionally homologous essential thiols in two membrane-bound phosphotransferase enzymes of Escherichia coli detected by permeant and nonpermeant thiol reagents. J Biol Chem. 1980 Jun 10;255(11):5075–5081. [PubMed] [Google Scholar]
- Haguenauer R., Kepes A. NaF inhibition of phosphorylation and dephosphorylation involved in -methyl-D glucoside transport in E. coli K 12. A pH dependant phenomenon sensitive to uncoupling agents. Biochimie. 1972;54(4):505–512. doi: 10.1016/s0300-9084(72)80235-9. [DOI] [PubMed] [Google Scholar]
- Hall B. G., Xu L. Nucleotide sequence, function, activation, and evolution of the cryptic asc operon of Escherichia coli K12. Mol Biol Evol. 1992 Jul;9(4):688–706. doi: 10.1093/oxfordjournals.molbev.a040753. [DOI] [PubMed] [Google Scholar]
- Hammen P. K., Waygood E. B., Klevit R. E. Reexamination of the secondary and tertiary structure of histidine-containing protein from Escherichia coli by homonuclear and heteronuclear NMR spectroscopy. Biochemistry. 1991 Dec 24;30(51):11842–11850. doi: 10.1021/bi00115a014. [DOI] [PubMed] [Google Scholar]
- Han M. K., Knutson J. R., Roseman S., Brand L. Sugar transport by the bacterial phosphotransferase system. Fluorescence studies of subunit interactions of enzyme I. J Biol Chem. 1990 Feb 5;265(4):1996–2003. [PubMed] [Google Scholar]
- Han M. K., Roseman S., Brand L. Sugar transport by the bacterial phosphotransferase system. Characterization of the sulfhydryl groups and site-specific labeling of enzyme I. J Biol Chem. 1990 Feb 5;265(4):1985–1995. [PubMed] [Google Scholar]
- Hanson T. E., Anderson R. L. Phosphoenolpyruvate-dependent formation of D-fructose 1-phosphate by a four-component phosphotransferase system. Proc Natl Acad Sci U S A. 1968 Sep;61(1):269–276. doi: 10.1073/pnas.61.1.269. [DOI] [PMC free article] [PubMed] [Google Scholar]
- Hardesty C., Colón G., Ferran C., DiRienzo J. M. Deletion analysis of sucrose metabolic genes from a Salmonella plasmid cloned in Escherichia coli K12. Plasmid. 1987 Sep;18(2):142–155. doi: 10.1016/0147-619x(87)90042-4. [DOI] [PubMed] [Google Scholar]
- Hardesty C., Ferran C., DiRienzo J. M. Plasmid-mediated sucrose metabolism in Escherichia coli: characterization of scrY, the structural gene for a phosphoenolpyruvate-dependent sucrose phosphotransferase system outer membrane porin. J Bacteriol. 1991 Jan;173(2):449–456. doi: 10.1128/jb.173.2.449-456.1991. [DOI] [PMC free article] [PubMed] [Google Scholar]
- Harwood J. P., Gazdar C., Prasad C., Peterkofsky A., Curtis S. J., Epstein W. Involvement of the glucose enzymes II of the sugar phosphotransferase system in the regulation of adenylate cyclase by glucose in Escherichia coli. J Biol Chem. 1976 Apr 25;251(8):2462–2468. [PubMed] [Google Scholar]
- Hausman S. Z., Thompson J., London J. Futile xylitol cycle in Lactobacillus casei. J Bacteriol. 1984 Oct;160(1):211–215. doi: 10.1128/jb.160.1.211-215.1984. [DOI] [PMC free article] [PubMed] [Google Scholar]
- Hays J. B., Simoni R. D., Roseman S. Sugar transport. V. A trimeric lactose-specific phosphocarrier protein of the Staphylococcus aureus phosphotransferase system. J Biol Chem. 1973 Feb 10;248(3):941–956. [PubMed] [Google Scholar]
- Heinrich R., Rapoport T. A. A linear steady-state treatment of enzymatic chains. General properties, control and effector strength. Eur J Biochem. 1974 Feb 15;42(1):89–95. doi: 10.1111/j.1432-1033.1974.tb03318.x. [DOI] [PubMed] [Google Scholar]
- Heller K. B., Lin E. C., Wilson T. H. Substrate specificity and transport properties of the glycerol facilitator of Escherichia coli. J Bacteriol. 1980 Oct;144(1):274–278. doi: 10.1128/jb.144.1.274-278.1980. [DOI] [PMC free article] [PubMed] [Google Scholar]
- Hengstenberg W., Reiche B., Eisermann R., Fischer R., Kessler U., Tarrach A., De Vos W. M., Kalbitzer H. R., Glaser S. Structure and function of proteins involved in sugar transport by the PTS of gram-positive bacteria. FEMS Microbiol Rev. 1989 Jun;5(1-2):35–42. doi: 10.1111/j.1574-6968.1989.tb14098.x. [DOI] [PubMed] [Google Scholar]
- Henikoff S., Haughn G. W., Calvo J. M., Wallace J. C. A large family of bacterial activator proteins. Proc Natl Acad Sci U S A. 1988 Sep;85(18):6602–6606. doi: 10.1073/pnas.85.18.6602. [DOI] [PMC free article] [PubMed] [Google Scholar]
- Henkin T. M., Grundy F. J., Nicholson W. L., Chambliss G. H. Catabolite repression of alpha-amylase gene expression in Bacillus subtilis involves a trans-acting gene product homologous to the Escherichia coli lacl and galR repressors. Mol Microbiol. 1991 Mar;5(3):575–584. doi: 10.1111/j.1365-2958.1991.tb00728.x. [DOI] [PubMed] [Google Scholar]
- Hernandez-Asensio M., Del Campo F. F. Enhancement of alpha-methylglucoside efflux by respiration in respiratory mutants of Escherichia coli K-12. Arch Biochem Biophys. 1980 Apr 1;200(2):309–318. doi: 10.1016/0003-9861(80)90360-4. [DOI] [PubMed] [Google Scholar]
- Hernandez-Asensio M., Ramirez J. M., Del Campo F. F. The control by respiration of the uptake of alpha-methyl glucoside in Escherichia coli K12. Arch Microbiol. 1975 Apr 7;103(2):155–162. doi: 10.1007/BF00436343. [DOI] [PubMed] [Google Scholar]
- Herzberg O. An atomic model for protein-protein phosphoryl group transfer. J Biol Chem. 1992 Dec 5;267(34):24819–24823. [PubMed] [Google Scholar]
- Herzberg O., Reddy P., Sutrina S., Saier M. H., Jr, Reizer J., Kapadia G. Structure of the histidine-containing phosphocarrier protein HPr from Bacillus subtilis at 2.0-A resolution. Proc Natl Acad Sci U S A. 1992 Mar 15;89(6):2499–2503. doi: 10.1073/pnas.89.6.2499. [DOI] [PMC free article] [PubMed] [Google Scholar]
- Honeyman A. L., Curtiss R., 3rd Isolation, characterization, and nucleotide sequence of the Streptococcus mutans mannitol-phosphate dehydrogenase gene and the mannitol-specific factor III gene of the phosphoenolpyruvate phosphotransferase system. Infect Immun. 1992 Aug;60(8):3369–3375. doi: 10.1128/iai.60.8.3369-3375.1992. [DOI] [PMC free article] [PubMed] [Google Scholar]
- Houman F., Diaz-Torres M. R., Wright A. Transcriptional antitermination in the bgl operon of E. coli is modulated by a specific RNA binding protein. Cell. 1990 Sep 21;62(6):1153–1163. doi: 10.1016/0092-8674(90)90392-r. [DOI] [PubMed] [Google Scholar]
- Hoving H., Lolkema J. S., Robillard G. T. Escherichia coli phosphoenolpyruvate-dependent phosphotransferase system: equilibrium kinetics and mechanism of enzyme i phosphorylation. Biochemistry. 1981 Jan 6;20(1):87–93. doi: 10.1021/bi00504a015. [DOI] [PubMed] [Google Scholar]
- Hummel U., Nuoffer C., Zanolari B., Erni B. A functional protein hybrid between the glucose transporter and the N-acetylglucosamine transporter of Escherichia coli. Protein Sci. 1992 Mar;1(3):356–362. doi: 10.1002/pro.5560010307. [DOI] [PMC free article] [PubMed] [Google Scholar]
- Hunter I. S., Kornberg H. L. Glucose transport of Escherichia coli growing in glucose-limited continuous culture. Biochem J. 1979 Jan 15;178(1):97–101. doi: 10.1042/bj1780097. [DOI] [PMC free article] [PubMed] [Google Scholar]
- Hurley J. H., Faber H. R., Worthylake D., Meadow N. D., Roseman S., Pettigrew D. W., Remington S. J. Structure of the regulatory complex of Escherichia coli IIIGlc with glycerol kinase. Science. 1993 Jan 29;259(5095):673–677. [PubMed] [Google Scholar]
- Island M. D., Wei B. Y., Kadner R. J. Structure and function of the uhp genes for the sugar phosphate transport system in Escherichia coli and Salmonella typhimurium. J Bacteriol. 1992 May;174(9):2754–2762. doi: 10.1128/jb.174.9.2754-2762.1992. [DOI] [PMC free article] [PubMed] [Google Scholar]
- Jablonski E. G., Brand L., Roseman S. Sugar transport by the bacterial phosphotransferase system. Preparation of a fluorescein derivative of the glucose-specific phosphocarrier protein IIIGlc and its binding to the phosphocarrier protein HPr. J Biol Chem. 1983 Aug 25;258(16):9690–9699. [PubMed] [Google Scholar]
- Jacobson G. R. Interrelationships between protein phosphorylation and oligomerization in transport and chemotaxis via the Escherichia coli mannitol phosphotransferase system. Res Microbiol. 1992 Jan;143(1):113–116. doi: 10.1016/0923-2508(92)90040-u. [DOI] [PubMed] [Google Scholar]
- Jacobson G. R., Kelly D. M., Finlay D. R. The intramembrane topography of the mannitol-specific enzyme II of the Escherichia coli phosphotransferase system. J Biol Chem. 1983 Mar 10;258(5):2955–2959. [PubMed] [Google Scholar]
- Jacobson G. R., Lee C. A., Leonard J. E., Saier M. H., Jr Mannitol-specific enzyme II of the bacterial phosphotransferase system. I. Properties of the purified permease. J Biol Chem. 1983 Sep 10;258(17):10748–10756. [PubMed] [Google Scholar]
- Jacobson G. R., Lee C. A., Saier M. H., Jr Purification of the mannitol-specific enzyme II of the Escherichia coli phosphoenolpyruvate:sugar phosphotransferase system. J Biol Chem. 1979 Jan 25;254(2):249–252. [PubMed] [Google Scholar]
- Jacobson G. R., Lodge J., Poy F. Carbohydrate uptake in the oral pathogen Streptococcus mutans: mechanisms and regulation by protein phosphorylation. Biochimie. 1989 Sep-Oct;71(9-10):997–1004. doi: 10.1016/0300-9084(89)90103-x. [DOI] [PubMed] [Google Scholar]
- Jacobson G. R., Stephan M. M. Structural and functional domains of the mannitol-specific enzyme II of the E. coli phosphoenolpyruvate-dependent phosphotransferase system. FEMS Microbiol Rev. 1989 Jun;5(1-2):25–34. doi: 10.1016/0168-6445(89)90005-3. [DOI] [PubMed] [Google Scholar]
- Jacobson G. R., Tanney L. E., Kelly D. M., Palman K. B., Corn S. B. Substrate and phospholipid specificity of the purified mannitol permease of Escherichia coli. J Cell Biochem. 1983;23(1-4):231–240. doi: 10.1002/jcb.240230120. [DOI] [PubMed] [Google Scholar]
- Jaffor Ullah A. H., Cirillo V. P. Mycoplasma phosphoenolpyruvate-dependent sugar phosphotransferase system: purification and characterization of enzyme I. J Bacteriol. 1977 Sep;131(3):988–996. doi: 10.1128/jb.131.3.988-996.1977. [DOI] [PMC free article] [PubMed] [Google Scholar]
- Jahreis K., Postma P. W., Lengeler J. W. Nucleotide sequence of the ilvH-fruR gene region of Escherichia coli K12 and Salmonella typhimurium LT2. Mol Gen Genet. 1991 Apr;226(1-2):332–336. doi: 10.1007/BF00273623. [DOI] [PubMed] [Google Scholar]
- Jenkinson H. F. Properties of a phosphocarrier protein (HPr) extracted from intact cells of Streptococcus sanguis. J Gen Microbiol. 1989 Dec;135(12):3183–3197. doi: 10.1099/00221287-135-12-3183. [DOI] [PubMed] [Google Scholar]
- Jiang W., Wu L. F., Tomich J., Saier M. H., Jr, Niehaus W. G. Corrected sequence of the mannitol (mtl) operon in Escherichia coli. Mol Microbiol. 1990 Nov;4(11):2003–2006. doi: 10.1111/j.1365-2958.1990.tb02050.x. [DOI] [PubMed] [Google Scholar]
- Jin R. Z., Lin E. C. An inducible phosphoenolpyruvate: dihydroxyacetone phosphotransferase system in Escherichia coli. J Gen Microbiol. 1984 Jan;130(1):83–88. doi: 10.1099/00221287-130-1-83. [DOI] [PubMed] [Google Scholar]
- Jones-Mortimer M. C., Kornberg H. L. Amino-sugar transport systems of Escherichia coli K12. J Gen Microbiol. 1980 Apr;117(2):369–376. doi: 10.1099/00221287-117-2-369. [DOI] [PubMed] [Google Scholar]
- Jones-Mortimer M. C., Kornberg H. L. Genetic control of inducer exclusion by Escherichia coli. FEBS Lett. 1974 Nov 1;48(1):93–95. doi: 10.1016/0014-5793(74)81070-7. [DOI] [PubMed] [Google Scholar]
- Jones-Mortimer M. C., Kornberg H. L. Genetical analysis of fructose utilization by Escherichia coli. Proc R Soc Lond B Biol Sci. 1974 Sep 17;187(1087):121–131. doi: 10.1098/rspb.1974.0066. [DOI] [PubMed] [Google Scholar]
- Joseph E., Bernsley C., Guiso N., Ullmann A. Multiple regulation of the activity of adenylate cyclase in Escherichia coli. Mol Gen Genet. 1982;185(2):262–268. doi: 10.1007/BF00330796. [DOI] [PubMed] [Google Scholar]
- KUNDIG W., GHOSH S., ROSEMAN S. PHOSPHATE BOUND TO HISTIDINE IN A PROTEIN AS AN INTERMEDIATE IN A NOVEL PHOSPHO-TRANSFERASE SYSTEM. Proc Natl Acad Sci U S A. 1964 Oct;52:1067–1074. doi: 10.1073/pnas.52.4.1067. [DOI] [PMC free article] [PubMed] [Google Scholar]
- Kaback H. R. Regulation of sugar transport in isolated bacterial membrane preparations from Escherichia coli. Proc Natl Acad Sci U S A. 1969 Jul;63(3):724–731. doi: 10.1073/pnas.63.3.724. [DOI] [PMC free article] [PubMed] [Google Scholar]
- Kacser H., Burns J. A. The control of flux. Symp Soc Exp Biol. 1973;27:65–104. [PubMed] [Google Scholar]
- Kalbitzer H. R., Deutscher J., Hengstenberg W., Rösch P. Phosphoenolpyruvate-dependent phosphotransferase system of Staphylococcus aureus: 1H nuclear magnetic resonance studies on phosphorylated and unphosphorylated factor IIIlac and its interaction with the phosphocarrier protein HPr. Biochemistry. 1981 Oct 13;20(21):6178–6185. doi: 10.1021/bi00524a041. [DOI] [PubMed] [Google Scholar]
- Kalbitzer H. R., Hengstenberg W., Rösch P., Muss P., Bernsmann P., Engelmann R., Dörschug M., Deutscher J. HPr proteins of different microorganisms studied by hydrogen-1 high-resolution nuclear magnetic resonance: similarities of structures and mechanisms. Biochemistry. 1982 Jun 8;21(12):2879–2885. doi: 10.1021/bi00541a012. [DOI] [PubMed] [Google Scholar]
- Kalbitzer H. R., Muss H. P., Engelmann R., Kiltz H. H., Stüber K., Hengstenberg W. Phosphoenolpyruvate-dependent phosphotransferase system. 1H NMR studies on chemically modified HPr proteins. Biochemistry. 1985 Aug 13;24(17):4562–4569. doi: 10.1021/bi00338a012. [DOI] [PubMed] [Google Scholar]
- Kalbitzer H. R., Neidig K. P., Hengstenberg W. Two-dimensional 1H NMR studies on HPr protein from Staphylococcus aureus: complete sequential assignments and secondary structure. Biochemistry. 1991 Nov 19;30(46):11186–11192. doi: 10.1021/bi00110a024. [DOI] [PubMed] [Google Scholar]
- Kelker N. E., Simkins R. A., Anderson R. L. Pathway of L-sorbose metabolism in Aerobacter aerogenes. J Biol Chem. 1972 Mar 10;247(5):1479–1483. [PubMed] [Google Scholar]
- Khandekar S. S., Jacobson G. R. Evidence for two distinct conformations of the Escherichia coli mannitol permease that are important for its transport and phosphorylation functions. J Cell Biochem. 1989 Feb;39(2):207–216. doi: 10.1002/jcb.240390212. [DOI] [PubMed] [Google Scholar]
- Kim K., Yoo O. J. Two subunits of mannose permease, II-PMan and II-MMan, of Escherichia coli mediate coliphage N4 infection. Biochem Int. 1989 Mar;18(3):545–549. [PubMed] [Google Scholar]
- Klein W., Ehmann U., Boos W. The repression of trehalose transport and metabolism in Escherichia coli by high osmolarity is mediated by trehalose-6-phosphate phosphatase. Res Microbiol. 1991 May;142(4):359–371. doi: 10.1016/0923-2508(91)90105-j. [DOI] [PubMed] [Google Scholar]
- Klevit R. E., Drobny G. P. Two-dimensional 1H NMR studies of histidine-containing protein from Escherichia coli. 2. Leucine resonance assignments by long-range coherence transfer. Biochemistry. 1986 Nov 18;25(23):7770–7773. doi: 10.1021/bi00371a072. [DOI] [PubMed] [Google Scholar]
- Klevit R. E., Drobny G. P., Waygood E. B. Two-dimensional 1H NMR studies of histidine-containing protein from Escherichia coli. 1. Sequential resonance assignments. Biochemistry. 1986 Nov 18;25(23):7760–7769. doi: 10.1021/bi00371a071. [DOI] [PubMed] [Google Scholar]
- Klevit R. E., Waygood E. B. Two-dimensional 1H NMR studies of histidine-containing protein from Escherichia coli. 3. Secondary and tertiary structure as determined by NMR. Biochemistry. 1986 Nov 18;25(23):7774–7781. doi: 10.1021/bi00371a073. [DOI] [PubMed] [Google Scholar]
- Klier A. F., Rapoport G. Genetics and regulation of carbohydrate catabolism in Bacillus. Annu Rev Microbiol. 1988;42:65–95. doi: 10.1146/annurev.mi.42.100188.000433. [DOI] [PubMed] [Google Scholar]
- Knowles J. R. Enzyme-catalyzed phosphoryl transfer reactions. Annu Rev Biochem. 1980;49:877–919. doi: 10.1146/annurev.bi.49.070180.004305. [DOI] [PubMed] [Google Scholar]
- Kofoid E. C., Parkinson J. S. Transmitter and receiver modules in bacterial signaling proteins. Proc Natl Acad Sci U S A. 1988 Jul;85(14):4981–4985. doi: 10.1073/pnas.85.14.4981. [DOI] [PMC free article] [PubMed] [Google Scholar]
- Kohlbrecher D., Eisermann R., Hengstenberg W. Staphylococcal phosphoenolpyruvate-dependent phosphotransferase system: molecular cloning and nucleotide sequence of the Staphylococcus carnosus ptsI gene and expression and complementation studies of the gene product. J Bacteriol. 1992 Apr;174(7):2208–2214. doi: 10.1128/jb.174.7.2208-2214.1992. [DOI] [PMC free article] [PubMed] [Google Scholar]
- Kolb A., Spassky A., Chapon C., Blazy B., Buc H. On the different binding affinities of CRP at the lac, gal and malT promoter regions. Nucleic Acids Res. 1983 Nov 25;11(22):7833–7852. doi: 10.1093/nar/11.22.7833. [DOI] [PMC free article] [PubMed] [Google Scholar]
- Kornberg H. L., Elvin C. M. Location and function of fruC, a gene involved in the regulation of fructose utilization by Escherichia coli. J Gen Microbiol. 1987 Feb;133(2):341–346. doi: 10.1099/00221287-133-2-341. [DOI] [PubMed] [Google Scholar]
- Kornberg H. L. Fine control of sugar uptake by Escherichia coli. Symp Soc Exp Biol. 1973;27:175–193. [PubMed] [Google Scholar]
- Kornberg H. L., Lambourne L. T. Role of the phosphoenolpyruvate-dependent fructose phosphotransferase system in the utilization of mannose by Escherichia coli. Proc Biol Sci. 1992 Oct 22;250(1327):51–55. doi: 10.1098/rspb.1992.0129. [DOI] [PubMed] [Google Scholar]
- Kornberg H. L., Riordan C. Uptake of galactose into Escherichia coli by facilitated diffusion. J Gen Microbiol. 1976 May;94(1):75–89. doi: 10.1099/00221287-94-1-75. [DOI] [PubMed] [Google Scholar]
- Kornberg H. L., Smith J. Role of phosphofructokinase in the utilization of glucose by Escherichia coli. Nature. 1970 Jul 4;227(5253):44–46. doi: 10.1038/227044a0. [DOI] [PubMed] [Google Scholar]
- Kricker M., Hall B. G. Biochemical genetics of the cryptic gene system for cellobiose utilization in Escherichia coli K12. Genetics. 1987 Mar;115(3):419–429. doi: 10.1093/genetics/115.3.419. [DOI] [PMC free article] [PubMed] [Google Scholar]
- Kricker M., Hall B. G. Directed evolution of cellobiose utilization in Escherichia coli K12. Mol Biol Evol. 1984 Feb;1(2):171–182. doi: 10.1093/oxfordjournals.molbev.a040310. [DOI] [PubMed] [Google Scholar]
- Kubota Y., Iuchi S., Fujisawa A., Tanaka S. Separation of four components of the phosphoenolpyruvate: glucose phosphotransferase system in Vibrio parahaemolyticus. Microbiol Immunol. 1979;23(3):131–146. doi: 10.1111/j.1348-0421.1979.tb00450.x. [DOI] [PubMed] [Google Scholar]
- Kukuruzinska M. A., Harrington W. F., Roseman S. Sugar transport by the bacterial phosphotransferase system. Studies on the molecular weight and association of enzyme I. J Biol Chem. 1982 Dec 10;257(23):14470–14476. [PubMed] [Google Scholar]
- Kundig W., Roseman S. Sugar transport. II. Characterization of constitutive membrane-bound enzymes II of the Escherichia coli phosphotransferase system. J Biol Chem. 1971 Mar 10;246(5):1407–1418. [PubMed] [Google Scholar]
- Kuroda M., de Waard S., Mizushima K., Tsuda M., Postma P., Tsuchiya T. Resistance of the melibiose carrier to inhibition by the phosphotransferase system due to substitutions of amino acid residues in the carrier of Salmonella typhimurium. J Biol Chem. 1992 Sep 15;267(26):18336–18341. [PubMed] [Google Scholar]
- Kühnau S., Reyes M., Sievertsen A., Shuman H. A., Boos W. The activities of the Escherichia coli MalK protein in maltose transport, regulation, and inducer exclusion can be separated by mutations. J Bacteriol. 1991 Apr;173(7):2180–2186. doi: 10.1128/jb.173.7.2180-2186.1991. [DOI] [PMC free article] [PubMed] [Google Scholar]
- Laoide B. M., Chambliss G. H., McConnell D. J. Bacillus licheniformis alpha-amylase gene, amyL, is subject to promoter-independent catabolite repression in Bacillus subtilis. J Bacteriol. 1989 May;171(5):2435–2442. doi: 10.1128/jb.171.5.2435-2442.1989. [DOI] [PMC free article] [PubMed] [Google Scholar]
- Lee C. A., Saier M. H., Jr Mannitol-specific enzyme II of the bacterial phosphotransferase system. III. The nucleotide sequence of the permease gene. J Biol Chem. 1983 Sep 10;258(17):10761–10767. [PubMed] [Google Scholar]
- Lengeler J. W., Mayer R. J., Schmid K. Phosphoenolpyruvate-dependent phosphotransferase system enzyme III and plasmid-encoded sucrose transport in Escherichia coli K-12. J Bacteriol. 1982 Jul;151(1):468–471. doi: 10.1128/jb.151.1.468-471.1982. [DOI] [PMC free article] [PubMed] [Google Scholar]
- Lengeler J. W., Titgemeyer F., Vogler A. P., Wöhrl B. M. Structures and homologies of carbohydrate: phosphotransferase system (PTS) proteins. Philos Trans R Soc Lond B Biol Sci. 1990 Jan 30;326(1236):489–504. doi: 10.1098/rstb.1990.0027. [DOI] [PubMed] [Google Scholar]
- Lengeler J. W., Vogler A. P. Molecular mechanisms of bacterial chemotaxis towards PTS-carbohydrates. FEMS Microbiol Rev. 1989 Jun;5(1-2):81–92. doi: 10.1016/0168-6445(89)90011-9. [DOI] [PubMed] [Google Scholar]
- Lengeler J., Auburger A. M., Mayer R., Pecher A. The phosphoenolpyruvate-dependent carbohydrate: phosphotransferase system enzymes II as chemoreceptors in chemotaxis of Escherichia coli K 12. Mol Gen Genet. 1981;183(1):163–170. doi: 10.1007/BF00270156. [DOI] [PubMed] [Google Scholar]
- Lengeler J. Characterisation of mutants of Escherichia coli K12, selected by resistance to streptozotocin. Mol Gen Genet. 1980;179(1):49–54. doi: 10.1007/BF00268445. [DOI] [PubMed] [Google Scholar]
- Lengeler J., Lin E. C. Reversal of the mannitol-sorbitol diauxie in Escherichia coli. J Bacteriol. 1972 Nov;112(2):840–848. doi: 10.1128/jb.112.2.840-848.1972. [DOI] [PMC free article] [PubMed] [Google Scholar]
- Lengeler J. Mutations affecting transport of the hexitols D-mannitol, D-glucitol, and galactitol in Escherichia coli K-12: isolation and mapping. J Bacteriol. 1975 Oct;124(1):26–38. doi: 10.1128/jb.124.1.26-38.1975. [DOI] [PMC free article] [PubMed] [Google Scholar]
- Lengeler J., Steinberger H. Analysis of regulatory mechanisms controlling the activity of the hexitol transport systems in Escherichia coli K12. Mol Gen Genet. 1978 Nov 16;167(1):75–82. doi: 10.1007/BF00270323. [DOI] [PubMed] [Google Scholar]
- Lengeler J., Steinberger H. Analysis of the regulatory mechanisms controlling the synthesis of the hexitol transport systems in Escherichia coli K12. Mol Gen Genet. 1978 Aug 17;164(2):163–169. doi: 10.1007/BF00267381. [DOI] [PubMed] [Google Scholar]
- Leonard J. E., Saier M. H., Jr Genetic dissection of catalytic activities of the Salmonella typhimurium mannitol enzyme II. J Bacteriol. 1981 Feb;145(2):1106–1109. doi: 10.1128/jb.145.2.1106-1109.1981. [DOI] [PMC free article] [PubMed] [Google Scholar]
- Leonard J. E., Saier M. H., Jr Mannitol-specific enzyme II of the bacterial phosphotransferase system. II. Reconstitution of vectorial transphosphorylation in phospholipid vesicles. J Biol Chem. 1983 Sep 10;258(17):10757–10760. [PubMed] [Google Scholar]
- LiCalsi C., Crocenzi T. S., Freire E., Roseman S. Sugar transport by the bacterial phosphotransferase system. Structural and thermodynamic domains of enzyme I of Salmonella typhimurium. J Biol Chem. 1991 Oct 15;266(29):19519–19527. [PubMed] [Google Scholar]
- Liao D. I., Kapadia G., Reddy P., Saier M. H., Jr, Reizer J., Herzberg O. Structure of the IIA domain of the glucose permease of Bacillus subtilis at 2.2-A resolution. Biochemistry. 1991 Oct 8;30(40):9583–9594. doi: 10.1021/bi00104a004. [DOI] [PubMed] [Google Scholar]
- Liberman E., Saffen D., Roseman S., Peterkofsky A. Inhibition of E. coli adenylate cyclase activity by inorganic orthophosphate is dependent on IIIglc of the phosphoenolpyruvate:glycose phosphotransferase system. Biochem Biophys Res Commun. 1986 Dec 30;141(3):1138–1144. doi: 10.1016/s0006-291x(86)80162-0. [DOI] [PubMed] [Google Scholar]
- Lin E. C. The genetics of bacterial transport systems. Annu Rev Genet. 1970;4:225–262. doi: 10.1146/annurev.ge.04.120170.001301. [DOI] [PubMed] [Google Scholar]
- Lis J. T., Schleif R. Different cyclic AMP requirements for induction of the arabinose and lactose operons of Escherichia coli. J Mol Biol. 1973 Sep 5;79(1):149–162. doi: 10.1016/0022-2836(73)90276-3. [DOI] [PubMed] [Google Scholar]
- Liu K. D., Roseman S. Kinetic characterization and regulation of phosphoenolpyruvate-dependent methyl alpha-D-glucopyranoside transport by Salmonella typhimurium membrane vesicles. Proc Natl Acad Sci U S A. 1983 Dec;80(23):7142–7145. doi: 10.1073/pnas.80.23.7142. [DOI] [PMC free article] [PubMed] [Google Scholar]
- Lodge J., Jacobson G. R. Starvation-induced stimulation of sugar uptake in Streptococcus mutans is due to an effect on the activities of preexisting proteins of the phosphotransferase system. Infect Immun. 1988 Oct;56(10):2594–2600. doi: 10.1128/iai.56.10.2594-2600.1988. [DOI] [PMC free article] [PubMed] [Google Scholar]
- Lolkema J. S., Dijkstra D. S., Robillard G. T. Mechanics of solute translocation catalyzed by enzyme IImtl of the phosphoenolpyruvate-dependent phosphotransferase system of Escherichia coli. Biochemistry. 1992 Jun 23;31(24):5514–5521. doi: 10.1021/bi00139a013. [DOI] [PubMed] [Google Scholar]
- Lolkema J. S., Dijkstra D. S., ten Hoeve-Duurkens R. H., Robillard G. T. Interaction between the cytoplasmic and membrane-bound domains of enzyme IImtl of the Escherichia coli phosphoenolpyruvate-dependent phosphotransferase system. Biochemistry. 1991 Jul 9;30(27):6721–6726. doi: 10.1021/bi00241a013. [DOI] [PubMed] [Google Scholar]
- Lolkema J. S., Dijkstra D. S., ten Hoeve-Duurkens R. H., Robillard G. T. The membrane-bound domain of the phosphotransferase enzyme IImtl of Escherichia coli constitutes a mannitol translocating unit. Biochemistry. 1990 Nov 27;29(47):10659–10663. doi: 10.1021/bi00499a012. [DOI] [PubMed] [Google Scholar]
- Lolkema J. S., Robillard G. T. Subunit structure and activity of the mannitol-specific enzyme II of the Escherichia coli phosphoenolpyruvate-dependent phosphotransferase system solubilized in detergent. Biochemistry. 1990 Oct 30;29(43):10120–10125. doi: 10.1021/bi00495a016. [DOI] [PubMed] [Google Scholar]
- Lolkema J. S., ten Hoeve-Duurkens R. H., Dijkstra D. S., Robillard G. T. Mechanistic coupling of transport and phosphorylation activity by enzyme IImtl of the Escherichia coli phosphoenolpyruvate-dependent phosphotransferase system. Biochemistry. 1991 Jul 9;30(27):6716–6721. doi: 10.1021/bi00241a012. [DOI] [PubMed] [Google Scholar]
- Lolkema J. S., ten Hoeve-Duurkens R. H., Robillard G. T. The phosphoenolpyruvate-dependent fructose-specific phosphotransferase system in Rhodopseudomonas sphaeroides. EIIFru possesses a Zn2+-binding site and a dithiol/disulfide redox centre. Eur J Biochem. 1986 Feb 3;154(3):651–656. doi: 10.1111/j.1432-1033.1986.tb09447.x. [DOI] [PubMed] [Google Scholar]
- Lolkema J. S., ten Hoeve-Duurkens R. H., Robillard G. T. The phosphoenolpyruvate-dependent fructose-specific phosphotransferase system in Rhodopseudomonas sphaeroides. Energetics of the phosphoryl group transfer from phosphoenolpyruvate to fructose. Eur J Biochem. 1986 Jan 15;154(2):387–393. doi: 10.1111/j.1432-1033.1986.tb09410.x. [DOI] [PubMed] [Google Scholar]
- Lolkema J. S., ten Hoeve-Duurkens R. H., Robillard G. T. The phosphoenolpyruvate-dependent fructose-specific phosphotransferase system in Rhodopseudomonas sphaeroides. Mechanism for transfer of the phosphoryl group from phosphoenolpyruvate to fructose. Eur J Biochem. 1985 Jun 18;149(3):625–631. doi: 10.1111/j.1432-1033.1985.tb08970.x. [DOI] [PubMed] [Google Scholar]
- London J., Hausman S. Z. Purification and characterization of the IIIXtl phospho-carrier protein of the phosphoenolpyruvate-dependent xylitol:phosphotransferase found in Lactobacillus casei C183. J Bacteriol. 1983 Nov;156(2):611–619. doi: 10.1128/jb.156.2.611-619.1983. [DOI] [PMC free article] [PubMed] [Google Scholar]
- Lukat G. S., McCleary W. R., Stock A. M., Stock J. B. Phosphorylation of bacterial response regulator proteins by low molecular weight phospho-donors. Proc Natl Acad Sci U S A. 1992 Jan 15;89(2):718–722. doi: 10.1073/pnas.89.2.718. [DOI] [PMC free article] [PubMed] [Google Scholar]
- Lévy S., De Reuse H., Danchin A. Antisense expression at the ptsH-ptsI locus of Escherichia coli. FEMS Microbiol Lett. 1989 Jan 1;48(1):35–38. doi: 10.1016/0378-1097(89)90142-0. [DOI] [PubMed] [Google Scholar]
- Lévy S., Zeng G. Q., Danchin A. Cyclic AMP synthesis in Escherichia coli strains bearing known deletions in the pts phosphotransferase operon. Gene. 1990 Jan 31;86(1):27–33. doi: 10.1016/0378-1119(90)90110-d. [DOI] [PubMed] [Google Scholar]
- Mahadevan S., Reynolds A. E., Wright A. Positive and negative regulation of the bgl operon in Escherichia coli. J Bacteriol. 1987 Jun;169(6):2570–2578. doi: 10.1128/jb.169.6.2570-2578.1987. [DOI] [PMC free article] [PubMed] [Google Scholar]
- Manayan R., Tenn G., Yee H. B., Desai J. D., Yamada M., Saier M. H., Jr Genetic analyses of the mannitol permease of Escherichia coli: isolation and characterization of a transport-deficient mutant which retains phosphorylation activity. J Bacteriol. 1988 Mar;170(3):1290–1296. doi: 10.1128/jb.170.3.1290-1296.1988. [DOI] [PMC free article] [PubMed] [Google Scholar]
- Manoil C., Beckwith J. A genetic approach to analyzing membrane protein topology. Science. 1986 Sep 26;233(4771):1403–1408. doi: 10.1126/science.3529391. [DOI] [PubMed] [Google Scholar]
- Markwell J., Shimamoto G. T., Bissett D. L., Anderson R. L. Pathway of galactitol catabolism in Klebsiella pneumoniae. Biochem Biophys Res Commun. 1976 Jul 12;71(1):221–227. doi: 10.1016/0006-291x(76)90271-0. [DOI] [PubMed] [Google Scholar]
- Marquet M., Creignou M. C., Dedonder R. The phosphoenolpyruvate : methyl-alpha-D-glucoside phosphotransferase system in Bacillus subtilis Marburg 168 : purification and identification of the phosphocarrier protein (HPr). Biochimie. 1976;58(4):435–441. doi: 10.1016/s0300-9084(76)80254-4. [DOI] [PubMed] [Google Scholar]
- Martin-Verstraete I., Débarbouillé M., Klier A., Rapoport G. Levanase operon of Bacillus subtilis includes a fructose-specific phosphotransferase system regulating the expression of the operon. J Mol Biol. 1990 Aug 5;214(3):657–671. doi: 10.1016/0022-2836(90)90284-S. [DOI] [PubMed] [Google Scholar]
- Maréchal L. R. Transport and metabolism of trehalose in Escherichia coli and Salmonella typhimurium. Arch Microbiol. 1984 Jan;137(1):70–73. doi: 10.1007/BF00425810. [DOI] [PubMed] [Google Scholar]
- Mason P. W., Carbone D. P., Cushman R. A., Waggoner A. S. The importance of inorganic phosphate in regulation of energy metabolism of Streptococcus lactis. J Biol Chem. 1981 Feb 25;256(4):1861–1866. [PubMed] [Google Scholar]
- Mattoo R. L., Waygood E. B. Determination of the levels of HPr and enzyme I of the phosphoenolpyruvate-sugar phosphotransferase system in Escherichia coli and Salmonella typhimurium. Can J Biochem Cell Biol. 1983 Jan;61(1):29–37. doi: 10.1139/o83-005. [DOI] [PubMed] [Google Scholar]
- McEntee K. Genetic analysis of the Escherichia coli K-12 srl region. J Bacteriol. 1977 Dec;132(3):904–911. doi: 10.1128/jb.132.3.904-911.1977. [DOI] [PMC free article] [PubMed] [Google Scholar]
- McKay L. L., Walter L. A., Sandine W. E., Elliker P. R. Involvement of phosphoenolpyruvate in lactose utilization by group N streptococci. J Bacteriol. 1969 Aug;99(2):603–610. doi: 10.1128/jb.99.2.603-610.1969. [DOI] [PMC free article] [PubMed] [Google Scholar]
- Meadow N. D., Coyle P., Komoryia A., Anfinsen C. B., Roseman S. Limited proteolysis of IIIGlc, a regulatory protein of the phosphoenolpyruvate:glycose phosphotransferase system, by membrane-associated enzymes from Salmonella typhimurium and Escherichia coli. J Biol Chem. 1986 Oct 15;261(29):13504–13509. [PubMed] [Google Scholar]
- Meadow N. D., Fox D. K., Roseman S. The bacterial phosphoenolpyruvate: glycose phosphotransferase system. Annu Rev Biochem. 1990;59:497–542. doi: 10.1146/annurev.bi.59.070190.002433. [DOI] [PubMed] [Google Scholar]
- Meadow N. D., Revuelta R., Chen V. N., Colwell R. R., Roseman S. Phosphoenolpyruvate:glycose phosphotransferase system in species of Vibrio, a widely distributed marine bacterial genus. J Bacteriol. 1987 Nov;169(11):4893–4900. doi: 10.1128/jb.169.11.4893-4900.1987. [DOI] [PMC free article] [PubMed] [Google Scholar]
- Meadow N. D., Roseman S. Sugar transport by the bacterial phosphotransferase system. Isolation and characterization of a glucose-specific phosphocarrier protein (IIIGlc) from Salmonella typhimurium. J Biol Chem. 1982 Dec 10;257(23):14526–14537. [PubMed] [Google Scholar]
- Meadow N. D., Rosenberg J. M., Pinkert H. M., Roseman S. Sugar transport by the bacterial phosphotransferase system. Evidence that crr is the structural gene for the Salmonella typhimurium glucose-specific phosphocarrier protein IIIGlc. J Biol Chem. 1982 Dec 10;257(23):14538–14542. [PubMed] [Google Scholar]
- Meins M., Jenö P., Müller D., Richter W. J., Rosenbusch J. P., Erni B. Cysteine phosphorylation of the glucose transporter of Escherichia coli. J Biol Chem. 1993 Jun 5;268(16):11604–11609. [PubMed] [Google Scholar]
- Meins M., Zanolari B., Rosenbusch J. P., Erni B. Glucose permease of Escherichia coli. Purification of the IIGlc subunit and functional characterization of its oligomeric forms. J Biol Chem. 1988 Sep 15;263(26):12986–12993. [PubMed] [Google Scholar]
- Melton T., Hartman P. E., Stratis J. P., Lee T. L., Davis A. T. Chemotaxis of Salmonella typhimurium to amino acids and some sugars. J Bacteriol. 1978 Feb;133(2):708–716. doi: 10.1128/jb.133.2.708-716.1978. [DOI] [PMC free article] [PubMed] [Google Scholar]
- Merrick M. J., Coppard J. R. Mutations in genes downstream of the rpoN gene (encoding sigma 54) of Klebsiella pneumoniae affect expression from sigma 54-dependent promoters. Mol Microbiol. 1989 Dec;3(12):1765–1775. doi: 10.1111/j.1365-2958.1989.tb00162.x. [DOI] [PubMed] [Google Scholar]
- Mimura C. S., Eisenberg L. B., Jacobson G. R. Resolution of the phosphotransferase enzymes of Streptococcus mutans: purification and preliminary characterization of a heat-stable phosphocarrier protein. Infect Immun. 1984 Jun;44(3):708–715. doi: 10.1128/iai.44.3.708-715.1984. [DOI] [PMC free article] [PubMed] [Google Scholar]
- Misko T. P., Mitchell W. J., Meadow N. D., Roseman S. Sugar transport by the bacterial phosphotransferase system. Reconstitution of inducer exclusion in Salmonella typhimurium membrane vesicles. J Biol Chem. 1987 Nov 25;262(33):16261–16266. [PubMed] [Google Scholar]
- Misset O., Blaauw M., Postma P. W., Robillard G. T. Bacterial phosphoenolpyruvate-dependent phosphotransferase system. Mechanism of the transmembrane sugar translocation and phosphorylation. Biochemistry. 1983 Dec 20;22(26):6163–6170. doi: 10.1021/bi00295a019. [DOI] [PubMed] [Google Scholar]
- Misset O., Brouwer M., Robillard G. T. Escherichia coli phosphoenolpyruvate-dependent phosphotransferase system. Evidence that the dimer is the active form of enzyme I. Biochemistry. 1980 Mar 4;19(5):883–890. doi: 10.1021/bi00546a009. [DOI] [PubMed] [Google Scholar]
- Mitchell W. J., Saffen D. W., Roseman S. Sugar transport by the bacterial phosphotransferase system. In vivo regulation of lactose transport in Escherichia coli by IIIGlc, a protein of the phosphoenolpyruvate:glycose phosphotransferase system. J Biol Chem. 1987 Nov 25;262(33):16254–16260. [PubMed] [Google Scholar]
- Mitchell W. J., Shaw J. E., Andrews L. Properties of the glucose phosphotransferase system of Clostridium acetobutylicum NCIB 8052. Appl Environ Microbiol. 1991 Sep;57(9):2534–2539. doi: 10.1128/aem.57.9.2534-2539.1991. [DOI] [PMC free article] [PubMed] [Google Scholar]
- Mock M., Crasnier M., Duflot E., Dumay V., Danchin A. Structural and functional relationships between Pasteurella multocida and enterobacterial adenylate cyclases. J Bacteriol. 1991 Oct;173(19):6265–6269. doi: 10.1128/jb.173.19.6265-6269.1991. [DOI] [PMC free article] [PubMed] [Google Scholar]
- Morris P. W., Binkley J. P., Henson J. M., Kuempel P. L. Cloning and location of the dgsA gene of Escherichia coli. J Bacteriol. 1985 Aug;163(2):785–786. doi: 10.1128/jb.163.2.785-786.1985. [DOI] [PMC free article] [PubMed] [Google Scholar]
- Morse M. L., Hill K. L., Egan J. B., Hengstenberg W. Metabolism of lactose by Staphylococcus aureus and its genetic basis. J Bacteriol. 1968 Jun;95(6):2270–2274. doi: 10.1128/jb.95.6.2270-2274.1968. [DOI] [PMC free article] [PubMed] [Google Scholar]
- Mueller E. G., Khandekar S. S., Knowles J. R., Jacobson G. R. Stereochemical course of the reactions catalyzed by the bacterial phosphoenolpyruvate:mannitol phosphotransferase system. Biochemistry. 1990 Jul 24;29(29):6892–6896. doi: 10.1021/bi00481a019. [DOI] [PubMed] [Google Scholar]
- Narindrasorasak S., Bridger W. A. Phosphoenolypyruvate synthetase of Escherichia coli: molecular weight, subunit composition, and identification of phosphohistidine in phosphoenzyme intermediate. J Biol Chem. 1977 May 25;252(10):3121–3127. [PubMed] [Google Scholar]
- Nelson S. O., Lengeler J., Postma P. W. Role of IIIGlc of the phosphoenolpyruvate-glucose phosphotransferase system in inducer exclusion in Escherichia coli. J Bacteriol. 1984 Oct;160(1):360–364. doi: 10.1128/jb.160.1.360-364.1984. [DOI] [PMC free article] [PubMed] [Google Scholar]
- Nelson S. O., Postma P. W. Interactions in vivo between IIIGlc of the phosphoenolpyruvate:sugar phosphotransferase system and the glycerol and maltose uptake systems of Salmonella typhimurium. Eur J Biochem. 1984 Feb 15;139(1):29–34. doi: 10.1111/j.1432-1033.1984.tb07971.x. [DOI] [PubMed] [Google Scholar]
- Nelson S. O., Scholte B. J., Postma P. W. Phosphoenolpyruvate:sugar phosphotransferase system-mediated regulation of carbohydrate metabolism in Salmonella typhimurium. J Bacteriol. 1982 May;150(2):604–615. doi: 10.1128/jb.150.2.604-615.1982. [DOI] [PMC free article] [PubMed] [Google Scholar]
- Nelson S. O., Schuitema A. R., Benne R., van der Ploeg L. H., Plijter J. S., Aan F., Postma P. W. Molecular cloning, sequencing, and expression of the crr gene: the structural gene for IIIGlc of the bacterial PEP:glucose phosphotransferase system. EMBO J. 1984 Jul;3(7):1587–1593. doi: 10.1002/j.1460-2075.1984.tb02015.x. [DOI] [PMC free article] [PubMed] [Google Scholar]
- Nelson S. O., Schuitema A. R., Postma P. W. The phosphoenolpyruvate:glucose phosphotransferase system of Salmonella typhimurium. The phosphorylated form of IIIGlc. Eur J Biochem. 1986 Jan 15;154(2):337–341. doi: 10.1111/j.1432-1033.1986.tb09402.x. [DOI] [PubMed] [Google Scholar]
- Nelson S. O., Wright J. K., Postma P. W. The mechanism of inducer exclusion. Direct interaction between purified III of the phosphoenolpyruvate:sugar phosphotransferase system and the lactose carrier of Escherichia coli. EMBO J. 1983;2(5):715–720. doi: 10.1002/j.1460-2075.1983.tb01490.x. [DOI] [PMC free article] [PubMed] [Google Scholar]
- Neyroz P., Brand L., Roseman S. Sugar transport by the bacterial phosphotransferase system. The intrinsic fluorescence of enzyme I. J Biol Chem. 1987 Nov 25;262(33):15900–15907. [PubMed] [Google Scholar]
- Nicholson W. L., Park Y. K., Henkin T. M., Won M., Weickert M. J., Gaskell J. A., Chambliss G. H. Catabolite repression-resistant mutations of the Bacillus subtilis alpha-amylase promoter affect transcription levels and are in an operator-like sequence. J Mol Biol. 1987 Dec 20;198(4):609–618. doi: 10.1016/0022-2836(87)90204-x. [DOI] [PubMed] [Google Scholar]
- Niersbach M., Kreuzaler F., Geerse R. H., Postma P. W., Hirsch H. J. Cloning and nucleotide sequence of the Escherichia coli K-12 ppsA gene, encoding PEP synthase. Mol Gen Genet. 1992 Jan;231(2):332–336. doi: 10.1007/BF00279808. [DOI] [PubMed] [Google Scholar]
- Niwano M., Taylor B. L. Novel sensory adaptation mechanism in bacterial chemotaxis to oxygen and phosphotransferase substrates. Proc Natl Acad Sci U S A. 1982 Jan;79(1):11–15. doi: 10.1073/pnas.79.1.11. [DOI] [PMC free article] [PubMed] [Google Scholar]
- Novotny M. J., Frederickson W. L., Waygood E. B., Saier M. H., Jr Allosteric regulation of glycerol kinase by enzyme IIIglc of the phosphotransferase system in Escherichia coli and Salmonella typhimurium. J Bacteriol. 1985 May;162(2):810–816. doi: 10.1128/jb.162.2.810-816.1985. [DOI] [PMC free article] [PubMed] [Google Scholar]
- Nuoffer C., Zanolari B., Erni B. Glucose permease of Escherichia coli. The effect of cysteine to serine mutations on the function, stability, and regulation of transport and phosphorylation. J Biol Chem. 1988 May 15;263(14):6647–6655. [PubMed] [Google Scholar]
- Orchard L. M., Kornberg H. L. Sequence similarities between the gene specifying 1-phosphofructokinase (fruK), genes specifying other kinases in Escherichia coli K12, and lacC of Staphylococcus aureus. Proc Biol Sci. 1990 Nov 22;242(1304):87–90. doi: 10.1098/rspb.1990.0108. [DOI] [PubMed] [Google Scholar]
- Oskouian B., Stewart G. C. Cloning and characterization of the repressor gene of the Staphylococcus aureus lactose operon. J Bacteriol. 1987 Dec;169(12):5459–5465. doi: 10.1128/jb.169.12.5459-5465.1987. [DOI] [PMC free article] [PubMed] [Google Scholar]
- Oskouian B., Stewart G. C. Repression and catabolite repression of the lactose operon of Staphylococcus aureus. J Bacteriol. 1990 Jul;172(7):3804–3812. doi: 10.1128/jb.172.7.3804-3812.1990. [DOI] [PMC free article] [PubMed] [Google Scholar]
- Osumi T., Saier M. H., Jr Regulation of lactose permease activity by the phosphoenolpyruvate:sugar phosphotransferase system: evidence for direct binding of the glucose-specific enzyme III to the lactose permease. Proc Natl Acad Sci U S A. 1982 Mar;79(5):1457–1461. doi: 10.1073/pnas.79.5.1457. [DOI] [PMC free article] [PubMed] [Google Scholar]
- Parker L. L., Hall B. G. A fourth Escherichia coli gene system with the potential to evolve beta-glucoside utilization. Genetics. 1988 Jul;119(3):485–490. doi: 10.1093/genetics/119.3.485. [DOI] [PMC free article] [PubMed] [Google Scholar]
- Parker L. L., Hall B. G. Characterization and nucleotide sequence of the cryptic cel operon of Escherichia coli K12. Genetics. 1990 Mar;124(3):455–471. doi: 10.1093/genetics/124.3.455. [DOI] [PMC free article] [PubMed] [Google Scholar]
- Parker L. L., Hall B. G. Mechanisms of activation of the cryptic cel operon of Escherichia coli K12. Genetics. 1990 Mar;124(3):473–482. doi: 10.1093/genetics/124.3.473. [DOI] [PMC free article] [PubMed] [Google Scholar]
- Parkinson J. S., Kofoid E. C. Communication modules in bacterial signaling proteins. Annu Rev Genet. 1992;26:71–112. doi: 10.1146/annurev.ge.26.120192.000443. [DOI] [PubMed] [Google Scholar]
- Parra F., Jones-Mortimer M. C., Kornberg H. L. Phosphotransferase-mediated regulation of carbohydrate utilization in Escherichia coli K12: the nature of the iex (crr) and gsr (tgs) mutations. J Gen Microbiol. 1983 Feb;129(2):337–348. doi: 10.1099/00221287-129-2-337. [DOI] [PubMed] [Google Scholar]
- Pas H. H., Ellory J. C., Robillard G. T. Bacterial phosphoenolpyruvate-dependent phosphotransferase system: association state of membrane-bound mannitol-specific enzyme II demonstrated by inactivation. Biochemistry. 1987 Oct 20;26(21):6689–6696. doi: 10.1021/bi00395a019. [DOI] [PubMed] [Google Scholar]
- Pas H. H., Meyer G. H., Kruizinga W. H., Tamminga K. S., van Weeghel R. P., Robillard G. T. 31phospho-NMR demonstration of phosphocysteine as a catalytic intermediate on the Escherichia coli phosphotransferase system EIIMtl. J Biol Chem. 1991 Apr 15;266(11):6690–6692. [PubMed] [Google Scholar]
- Pas H. H., Robillard G. T. Enzyme IIMtl of the Escherichia coli phosphoenolpyruvate-dependent phosphotransferase system: identification of the activity-linked cysteine on the mannitol carrier. Biochemistry. 1988 Jul 26;27(15):5515–5519. doi: 10.1021/bi00415a019. [DOI] [PubMed] [Google Scholar]
- Pas H. H., Robillard G. T. S-phosphocysteine and phosphohistidine are intermediates in the phosphoenolpyruvate-dependent mannitol transport catalyzed by Escherichia coli EIIMtl. Biochemistry. 1988 Aug 9;27(16):5835–5839. doi: 10.1021/bi00416a002. [DOI] [PubMed] [Google Scholar]
- Pas H. H., ten Hoeve-Duurkens R. H., Robillard G. T. Bacterial phosphoenolpyruvate-dependent phosphotransferase system: mannitol-specific EII contains two phosphoryl binding sites per monomer and one high-affinity mannitol binding site per dimer. Biochemistry. 1988 Jul 26;27(15):5520–5525. doi: 10.1021/bi00415a020. [DOI] [PubMed] [Google Scholar]
- Pastan I., Perlman R. L. Repression of beta-galactosidase synthesis by glucose in phosphotransferase mutants of Escherichia coli. Repression in the absence of glucose phosphorylation. J Biol Chem. 1969 Nov 10;244(21):5836–5842. [PubMed] [Google Scholar]
- Pelton J. G., Torchia D. A., Meadow N. D., Roseman S. Structural comparison of phosphorylated and unphosphorylated forms of IIIGlc, a signal-transducing protein from Escherichia coli, using three-dimensional NMR techniques. Biochemistry. 1992 Jun 9;31(22):5215–5224. doi: 10.1021/bi00137a017. [DOI] [PubMed] [Google Scholar]
- Pelton J. G., Torchia D. A., Meadow N. D., Wong C. Y., Roseman S. 1H, 15N, and 13C NMR signal assignments of IIIGlc, a signal-transducing protein of Escherichia coli, using three-dimensional triple-resonance techniques. Biochemistry. 1991 Oct 15;30(41):10043–10057. doi: 10.1021/bi00105a032. [DOI] [PubMed] [Google Scholar]
- Pelton J. G., Torchia D. A., Meadow N. D., Wong C. Y., Roseman S. Secondary structure of the phosphocarrier protein IIIGlc, a signal-transducing protein from Escherichia coli, determined by heteronuclear three-dimensional NMR spectroscopy. Proc Natl Acad Sci U S A. 1991 Apr 15;88(8):3479–3483. doi: 10.1073/pnas.88.8.3479. [DOI] [PMC free article] [PubMed] [Google Scholar]
- Perham R. N. Domains, motifs, and linkers in 2-oxo acid dehydrogenase multienzyme complexes: a paradigm in the design of a multifunctional protein. Biochemistry. 1991 Sep 3;30(35):8501–8512. doi: 10.1021/bi00099a001. [DOI] [PubMed] [Google Scholar]
- Peri K. G., Goldie H., Waygood E. B. Cloning and characterization of the N-acetylglucosamine operon of Escherichia coli. Biochem Cell Biol. 1990 Jan;68(1):123–137. doi: 10.1139/o90-017. [DOI] [PubMed] [Google Scholar]
- Peri K. G., Waygood E. B. Sequence of cloned enzyme IIN-acetylglucosamine of the phosphoenolpyruvate:N-acetylglucosamine phosphotransferase system of Escherichia coli. Biochemistry. 1988 Aug 9;27(16):6054–6061. doi: 10.1021/bi00416a034. [DOI] [PubMed] [Google Scholar]
- Peterkofsky A., Gazdar C. Escherichia coli adenylate cyclase complex: regulation by the proton electrochemical gradient. Proc Natl Acad Sci U S A. 1979 Mar;76(3):1099–1103. doi: 10.1073/pnas.76.3.1099. [DOI] [PMC free article] [PubMed] [Google Scholar]
- Peterkofsky A., Gazdar C. Interaction of enzyme I of the phosphoenolpyruvate:sugar phosphotransferase system with adenylate cyclase of Escherichia coli. Proc Natl Acad Sci U S A. 1975 Aug;72(8):2920–2924. doi: 10.1073/pnas.72.8.2920. [DOI] [PMC free article] [PubMed] [Google Scholar]
- Peterkofsky A., Svenson I., Amin N. Regulation of Escherichia coli adenylate cyclase activity by the phosphoenolpyruvate:sugar phosphotransferase system. FEMS Microbiol Rev. 1989 Jun;5(1-2):103–108. doi: 10.1016/0168-6445(89)90013-2. [DOI] [PubMed] [Google Scholar]
- Plumbridge J. A. Induction of the nag regulon of Escherichia coli by N-acetylglucosamine and glucosamine: role of the cyclic AMP-catabolite activator protein complex in expression of the regulon. J Bacteriol. 1990 May;172(5):2728–2735. doi: 10.1128/jb.172.5.2728-2735.1990. [DOI] [PMC free article] [PubMed] [Google Scholar]
- Plumbridge J. A. Repression and induction of the nag regulon of Escherichia coli K-12: the roles of nagC and nagA in maintenance of the uninduced state. Mol Microbiol. 1991 Aug;5(8):2053–2062. doi: 10.1111/j.1365-2958.1991.tb00828.x. [DOI] [PubMed] [Google Scholar]
- Plumbridge J. A. Sequence of the nagBACD operon in Escherichia coli K12 and pattern of transcription within the nag regulon. Mol Microbiol. 1989 Apr;3(4):505–515. doi: 10.1111/j.1365-2958.1989.tb00197.x. [DOI] [PubMed] [Google Scholar]
- Plumbridge J., Kolb A. CAP and Nag repressor binding to the regulatory regions of the nagE-B and manX genes of Escherichia coli. J Mol Biol. 1991 Feb 20;217(4):661–679. doi: 10.1016/0022-2836(91)90524-a. [DOI] [PubMed] [Google Scholar]
- Pocalyko D. J., Carroll L. J., Martin B. M., Babbitt P. C., Dunaway-Mariano D. Analysis of sequence homologies in plant and bacterial pyruvate phosphate dikinase, enzyme I of the bacterial phosphoenolpyruvate: sugar phosphotransferase system and other PEP-utilizing enzymes. Identification of potential catalytic and regulatory motifs. Biochemistry. 1990 Dec 4;29(48):10757–10765. doi: 10.1021/bi00500a006. [DOI] [PubMed] [Google Scholar]
- Poolman B., Modderman R., Reizer J. Lactose transport system of Streptococcus thermophilus. The role of histidine residues. J Biol Chem. 1992 May 5;267(13):9150–9157. [PubMed] [Google Scholar]
- Poolman B., Royer T. J., Mainzer S. E., Schmidt B. F. Lactose transport system of Streptococcus thermophilus: a hybrid protein with homology to the melibiose carrier and enzyme III of phosphoenolpyruvate-dependent phosphotransferase systems. J Bacteriol. 1989 Jan;171(1):244–253. doi: 10.1128/jb.171.1.244-253.1989. [DOI] [PMC free article] [PubMed] [Google Scholar]
- Portlock S. H., Lee Y., Tomich J. M., Tamm L. K. Insertion and folding of the amino-terminal amphiphilic signal sequences of the mannitol and glucitol permeases of Escherichia coli. J Biol Chem. 1992 Jun 5;267(16):11017–11022. [PubMed] [Google Scholar]
- Postma P. W., Broekhuizen C. P., Geerse R. H. The role of the PEP: carbohydrate phosphotransferase system in the regulation of bacterial metabolism. FEMS Microbiol Rev. 1989 Jun;5(1-2):69–80. doi: 10.1016/0168-6445(89)90010-7. [DOI] [PubMed] [Google Scholar]
- Postma P. W. Defective enzyme II-BGlc of the phosphoenolpyruvate:sugar phosphotransferase system leading to uncoupling of transport and phosphorylation in Salmonella typhimurium. J Bacteriol. 1981 Aug;147(2):382–389. doi: 10.1128/jb.147.2.382-389.1981. [DOI] [PMC free article] [PubMed] [Google Scholar]
- Postma P. W., Epstein W., Schuitema A. R., Nelson S. O. Interaction between IIIGlc of the phosphoenolpyruvate:sugar phosphotransferase system and glycerol kinase of Salmonella typhimurium. J Bacteriol. 1984 Apr;158(1):351–353. doi: 10.1128/jb.158.1.351-353.1984. [DOI] [PMC free article] [PubMed] [Google Scholar]
- Postma P. W. Involvement of the phosphotransferase system in galactose transport in Salmonella typhimurium. FEBS Lett. 1976 Jan 1;61(1):49–53. doi: 10.1016/0014-5793(76)80169-x. [DOI] [PubMed] [Google Scholar]
- Postma P. W., Keizer H. G., Koolwijk P. Transport of trehalose in Salmonella typhimurium. J Bacteriol. 1986 Dec;168(3):1107–1111. doi: 10.1128/jb.168.3.1107-1111.1986. [DOI] [PMC free article] [PubMed] [Google Scholar]
- Postma P. W., Lengeler J. W. Phosphoenolpyruvate:carbohydrate phosphotransferase system of bacteria. Microbiol Rev. 1985 Sep;49(3):232–269. doi: 10.1128/mr.49.3.232-269.1985. [DOI] [PMC free article] [PubMed] [Google Scholar]
- Postma P. W., Stock J. B. Enzymes II of the phosphotransferase system do not catalyze sugar transport in the absence of phosphorylation. J Bacteriol. 1980 Feb;141(2):476–484. doi: 10.1128/jb.141.2.476-484.1980. [DOI] [PMC free article] [PubMed] [Google Scholar]
- Potter K., Chaloner-Larsson G., Yamazaki H. Abnormally high rate of cyclic AMP excretion from an Escherichia coli mutant deficient in cyclic AMP receptor protein. Biochem Biophys Res Commun. 1974 Mar 25;57(2):379–385. doi: 10.1016/0006-291x(74)90941-3. [DOI] [PubMed] [Google Scholar]
- Powers D. A., Roseman S. The primary structure of Salmonella typhimurium HPr, a phosphocarrier protein of the phosphoenolpyruvate:glycose phosphotransferase system. A correction. J Biol Chem. 1984 Dec 25;259(24):15212–15214. [PubMed] [Google Scholar]
- Prasad I., Schaefler S. Regulation of the beta-glucoside system in Escherchia coli K-12. J Bacteriol. 1974 Nov;120(2):638–650. doi: 10.1128/jb.120.2.638-650.1974. [DOI] [PMC free article] [PubMed] [Google Scholar]
- Presper K. A., Wong C. Y., Liu L., Meadow N. D., Roseman S. Site-directed mutagenesis of the phosphocarrier protein. IIIGlc, a major signal-transducing protein in Escherichia coli. Proc Natl Acad Sci U S A. 1989 Jun;86(11):4052–4055. doi: 10.1073/pnas.86.11.4052. [DOI] [PMC free article] [PubMed] [Google Scholar]
- Pries A., Priefert H., Krüger N., Steinbüchel A. Identification and characterization of two Alcaligenes eutrophus gene loci relevant to the poly(beta-hydroxybutyric acid)-leaky phenotype which exhibit homology to ptsH and ptsI of Escherichia coli. J Bacteriol. 1991 Sep;173(18):5843–5853. doi: 10.1128/jb.173.18.5843-5853.1991. [DOI] [PMC free article] [PubMed] [Google Scholar]
- Prior T. I., Kornberg H. L. Nucleotide sequence of fruA, the gene specifying enzyme IIfru of the phosphoenolpyruvate-dependent sugar phosphotransferase system in Escherichia coli K12. J Gen Microbiol. 1988 Oct;134(10):2757–2768. doi: 10.1099/00221287-134-10-2757. [DOI] [PubMed] [Google Scholar]
- Reddy P., Meadow N., Roseman S., Peterkofsky A. Reconstitution of regulatory properties of adenylate cyclase in Escherichia coli extracts. Proc Natl Acad Sci U S A. 1985 Dec;82(24):8300–8304. doi: 10.1073/pnas.82.24.8300. [DOI] [PMC free article] [PubMed] [Google Scholar]
- Reddy P., Miller D., Peterkofsky A. Stimulation of Escherichia coli adenylate cyclase activity by elongation factor Tu, a GTP-binding protein essential for protein synthesis. J Biol Chem. 1986 Sep 5;261(25):11448–11451. [PubMed] [Google Scholar]
- Reiche B., Frank R., Deutscher J., Meyer N., Hengstenberg W. Staphylococcal phosphoenolpyruvate-dependent phosphotransferase system: purification and characterization of the mannitol-specific enzyme IIImtl of Staphylococcus aureus and Staphylococcus carnosus and homology with the enzyme IImtl of Escherichia coli. Biochemistry. 1988 Aug 23;27(17):6512–6516. doi: 10.1021/bi00417a047. [DOI] [PubMed] [Google Scholar]
- Reider E., Wagner E. F., Schweiger M. Control of phosphoenolpyruvate-dependent phosphotransferase-mediated sugar transport in Escherichia coli by energization of the cell membrane. Proc Natl Acad Sci U S A. 1979 Nov;76(11):5529–5533. doi: 10.1073/pnas.76.11.5529. [DOI] [PMC free article] [PubMed] [Google Scholar]
- Reidl J., Boos W. The malX malY operon of Escherichia coli encodes a novel enzyme II of the phosphotransferase system recognizing glucose and maltose and an enzyme abolishing the endogenous induction of the maltose system. J Bacteriol. 1991 Aug;173(15):4862–4876. doi: 10.1128/jb.173.15.4862-4876.1991. [DOI] [PMC free article] [PubMed] [Google Scholar]
- Reiner A. M. Xylitol and D-arabitol toxicities due to derepressed fructose, galactitol, and sorbitol phosphotransferases of Escherichia coli. J Bacteriol. 1977 Oct;132(1):166–173. doi: 10.1128/jb.132.1.166-173.1977. [DOI] [PMC free article] [PubMed] [Google Scholar]
- Reizer A., Pao G. M., Saier M. H., Jr Evolutionary relationships among the permease proteins of the bacterial phosphoenolpyruvate: sugar phosphotransferase system. Construction of phylogenetic trees and possible relatedness to proteins of eukaryotic mitochondria. J Mol Evol. 1991 Aug;33(2):179–193. doi: 10.1007/BF02193633. [DOI] [PubMed] [Google Scholar]
- Reizer J., Deutscher J., Saier M. H., Jr Metabolite-sensitive, ATP-dependent, protein kinase-catalyzed phosphorylation of HPr, a phosphocarrier protein of the phosphotransferase system in gram-positive bacteria. Biochimie. 1989 Sep-Oct;71(9-10):989–996. doi: 10.1016/0300-9084(89)90102-8. [DOI] [PubMed] [Google Scholar]
- Reizer J., Novotny M. J., Hengstenberg W., Saier M. H., Jr Properties of ATP-dependent protein kinase from Streptococcus pyogenes that phosphorylates a seryl residue in HPr, a phosphocarrier protein of the phosphotransferase system. J Bacteriol. 1984 Oct;160(1):333–340. doi: 10.1128/jb.160.1.333-340.1984. [DOI] [PMC free article] [PubMed] [Google Scholar]
- Reizer J., Novotny M. J., Panos C., Saier M. H., Jr Mechanism of inducer expulsion in Streptococcus pyogenes: a two-step process activated by ATP. J Bacteriol. 1983 Oct;156(1):354–361. doi: 10.1128/jb.156.1.354-361.1983. [DOI] [PMC free article] [PubMed] [Google Scholar]
- Reizer J., Novotny M. J., Stuiver I., Saier M. H., Jr Regulation of glycerol uptake by the phosphoenolpyruvate-sugar phosphotransferase system in Bacillus subtilis. J Bacteriol. 1984 Jul;159(1):243–250. doi: 10.1128/jb.159.1.243-250.1984. [DOI] [PMC free article] [PubMed] [Google Scholar]
- Reizer J., Panos C. Regulation of beta-galactoside phosphate accumulation in Streptococcus pyogenes by an expulsion mechanism. Proc Natl Acad Sci U S A. 1980 Sep;77(9):5497–5501. doi: 10.1073/pnas.77.9.5497. [DOI] [PMC free article] [PubMed] [Google Scholar]
- Reizer J., Peterkofsky A., Romano A. H. Evidence for the presence of heat-stable protein (HPr) and ATP-dependent HPr kinase in heterofermentative lactobacilli lacking phosphoenolpyruvate:glycose phosphotransferase activity. Proc Natl Acad Sci U S A. 1988 Apr;85(7):2041–2045. doi: 10.1073/pnas.85.7.2041. [DOI] [PMC free article] [PubMed] [Google Scholar]
- Reizer J., Reizer A., Saier M. H., Jr, Jacobson G. R. A proposed link between nitrogen and carbon metabolism involving protein phosphorylation in bacteria. Protein Sci. 1992 Jun;1(6):722–726. doi: 10.1002/pro.5560010604. [DOI] [PMC free article] [PubMed] [Google Scholar]
- Reizer J., Reizer A., Saier M. H., Jr The cellobiose permease of Escherichia coli consists of three proteins and is homologous to the lactose permease of Staphylococcus aureus. Res Microbiol. 1990 Nov-Dec;141(9):1061–1067. doi: 10.1016/0923-2508(90)90079-6. [DOI] [PubMed] [Google Scholar]
- Reizer J., Saier M. H., Jr, Deutscher J., Grenier F., Thompson J., Hengstenberg W. The phosphoenolpyruvate:sugar phosphotransferase system in gram-positive bacteria: properties, mechanism, and regulation. Crit Rev Microbiol. 1988;15(4):297–338. doi: 10.3109/10408418809104461. [DOI] [PubMed] [Google Scholar]
- Reizer J., Saier M. H., Jr Involvement of lactose enzyme II of the phosphotransferase system in rapid expulsion of free galactosides from Streptococcus pyogenes. J Bacteriol. 1983 Oct;156(1):236–242. doi: 10.1128/jb.156.1.236-242.1983. [DOI] [PMC free article] [PubMed] [Google Scholar]
- Reizer J., Sutrina S. L., Saier M. H., Stewart G. C., Peterkofsky A., Reddy P. Mechanistic and physiological consequences of HPr(ser) phosphorylation on the activities of the phosphoenolpyruvate:sugar phosphotransferase system in gram-positive bacteria: studies with site-specific mutants of HPr. EMBO J. 1989 Jul;8(7):2111–2120. doi: 10.1002/j.1460-2075.1989.tb03620.x. [DOI] [PMC free article] [PubMed] [Google Scholar]
- Reizer J., Sutrina S. L., Wu L. F., Deutscher J., Reddy P., Saier M. H., Jr Functional interactions between proteins of the phosphoenolpyruvate:sugar phosphotransferase systems of Bacillus subtilis and Escherichia coli. J Biol Chem. 1992 May 5;267(13):9158–9169. [PubMed] [Google Scholar]
- Rephaeli A. W., Saier M. H., Jr Kinetic analyses of the sugar phosphate:sugar transphosphorylation reaction catalyzed by the glucose enzyme II complex of the bacterial phosphotransferase system. J Biol Chem. 1978 Nov 10;253(21):7595–7597. [PubMed] [Google Scholar]
- Rephaeli A. W., Saier M. H., Jr Regulation of genes coding for enzyme constituents of the bacterial phosphotransferase system. J Bacteriol. 1980 Feb;141(2):658–663. doi: 10.1128/jb.141.2.658-663.1980. [DOI] [PMC free article] [PubMed] [Google Scholar]
- Reynolds A. E., Felton J., Wright A. Insertion of DNA activates the cryptic bgl operon in E. coli K12. Nature. 1981 Oct 22;293(5834):625–629. doi: 10.1038/293625a0. [DOI] [PubMed] [Google Scholar]
- Robillard G. T., Blaauw M. Enzyme II of the Escherichia coli phosphoenolpyruvate-dependent phosphotransferase system: protein-protein and protein-phospholipid interactions. Biochemistry. 1987 Sep 8;26(18):5796–5803. doi: 10.1021/bi00392a032. [DOI] [PubMed] [Google Scholar]
- Robillard G. T., Konings W. N. Physical mechanism for regulation of phosphoenolpyruvate-dependent glucose transport activity in Escherichia coli. Biochemistry. 1981 Aug 18;20(17):5025–5032. doi: 10.1021/bi00520a032. [DOI] [PubMed] [Google Scholar]
- Robillard G. T., Lolkema J. S. Enzymes II of the phosphoenolpyruvate-dependent sugar transport systems: a review of their structure and mechanism of sugar transport. Biochim Biophys Acta. 1988 Oct 11;947(3):493–519. doi: 10.1016/0304-4157(88)90005-6. [DOI] [PubMed] [Google Scholar]
- Roehl R. A., Vinopal R. T. Genetic locus, distant from ptsM, affecting enzyme IIA/IIB function in Escherichia coli K-12. J Bacteriol. 1980 Apr;142(1):120–130. doi: 10.1128/jb.142.1.120-130.1980. [DOI] [PMC free article] [PubMed] [Google Scholar]
- Roehl R. A., Vinopal R. T. Lack of glucose phosphotransferase function in phosphofructokinase mutants of Escherichia coli. J Bacteriol. 1976 May;126(2):852–860. doi: 10.1128/jb.126.2.852-860.1976. [DOI] [PMC free article] [PubMed] [Google Scholar]
- Rogers M. J., Ohgi T., Plumbridge J., Söll D. Nucleotide sequences of the Escherichia coli nagE and nagB genes: the structural genes for the N-acetylglucosamine transport protein of the bacterial phosphoenolpyruvate: sugar phosphotransferase system and for glucosamine-6-phosphate deaminase. Gene. 1988;62(2):197–207. doi: 10.1016/0378-1119(88)90558-6. [DOI] [PubMed] [Google Scholar]
- Romano A. H., Brino G., Peterkofsky A., Reizer J. Regulation of beta-galactoside transport and accumulation in heterofermentative lactic acid bacteria. J Bacteriol. 1987 Dec;169(12):5589–5596. doi: 10.1128/jb.169.12.5589-5596.1987. [DOI] [PMC free article] [PubMed] [Google Scholar]
- Romano A. H., Saier M. H., Jr, Harriott O. T., Reizer J. Physiological studies on regulation of glycerol utilization by the phosphoenolpyruvate:sugar phosphotransferase system in Enterococcus faecalis. J Bacteriol. 1990 Dec;172(12):6741–6748. doi: 10.1128/jb.172.12.6741-6748.1990. [DOI] [PMC free article] [PubMed] [Google Scholar]
- Roossien F. F., Blaauw M., Robillard G. T. Kinetics and subunit interaction of the mannitol-specific enzyme II of the Escherichia coli phosphoenolpyruvate-dependent phosphotransferase system. Biochemistry. 1984 Oct 9;23(21):4934–4939. doi: 10.1021/bi00316a017. [DOI] [PubMed] [Google Scholar]
- Roossien F. F., Robillard G. T. Mannitol-specific carrier protein from the Escherichia coli phosphoenolpyruvate-dependent phosphotransferase system can be extracted as a dimer from the membrane. Biochemistry. 1984 Nov 20;23(24):5682–5685. doi: 10.1021/bi00319a003. [DOI] [PubMed] [Google Scholar]
- Roossien F. F., van Es-Spiekman W., Robillard G. T. Dimeric enzyme IImtl of the E. coli phosphoenolpyruvate-dependent phosphotransferase system. Cross-linking studies with bifunctional sulfhydryl reagents. FEBS Lett. 1986 Feb 17;196(2):284–290. doi: 10.1016/0014-5793(86)80264-2. [DOI] [PubMed] [Google Scholar]
- Roseman S. Sialic acid, serendipity, and sugar transport: discovery of the bacterial phosphotransferase system. FEMS Microbiol Rev. 1989 Jun;5(1-2):3–11. doi: 10.1016/0168-6445(89)90003-x. [DOI] [PubMed] [Google Scholar]
- Rosenberg H., Hardy C. M. Conversion of D-mannitol to D-ribose: a newly discovered pathway in Escherichia coli. J Bacteriol. 1984 Apr;158(1):69–72. doi: 10.1128/jb.158.1.69-72.1984. [DOI] [PMC free article] [PubMed] [Google Scholar]
- Rosey E. L., Oskouian B., Stewart G. C. Lactose metabolism by Staphylococcus aureus: characterization of lacABCD, the structural genes of the tagatose 6-phosphate pathway. J Bacteriol. 1991 Oct;173(19):5992–5998. doi: 10.1128/jb.173.19.5992-5998.1991. [DOI] [PMC free article] [PubMed] [Google Scholar]
- Rosey E. L., Stewart G. C. Nucleotide and deduced amino acid sequences of the lacR, lacABCD, and lacFE genes encoding the repressor, tagatose 6-phosphate gene cluster, and sugar-specific phosphotransferase system components of the lactose operon of Streptococcus mutans. J Bacteriol. 1992 Oct;174(19):6159–6170. doi: 10.1128/jb.174.19.6159-6170.1992. [DOI] [PMC free article] [PubMed] [Google Scholar]
- Ruijter G. J., Postma P. W., van Dam K. Adaptation of Salmonella typhimurium mutants containing uncoupled enzyme IIGlc to glucose-limited conditions. J Bacteriol. 1990 Sep;172(9):4783–4789. doi: 10.1128/jb.172.9.4783-4789.1990. [DOI] [PMC free article] [PubMed] [Google Scholar]
- Ruijter G. J., Postma P. W., van Dam K. Energetics of glucose uptake in a Salmonella typhimurium mutant containing uncoupled enzyme IIGlc. Arch Microbiol. 1991;155(3):234–237. doi: 10.1007/BF00252206. [DOI] [PubMed] [Google Scholar]
- Ruijter G. J., van Meurs G., Verwey M. A., Postma P. W., van Dam K. Analysis of mutations that uncouple transport from phosphorylation in enzyme IIGlc of the Escherichia coli phosphoenolpyruvate-dependent phosphotransferase system. J Bacteriol. 1992 May;174(9):2843–2850. doi: 10.1128/jb.174.9.2843-2850.1992. [DOI] [PMC free article] [PubMed] [Google Scholar]
- Ruyter G. J., Postma P. W., van Dam K. Control of glucose metabolism by enzyme IIGlc of the phosphoenolpyruvate-dependent phosphotransferase system in Escherichia coli. J Bacteriol. 1991 Oct;173(19):6184–6191. doi: 10.1128/jb.173.19.6184-6191.1991. [DOI] [PMC free article] [PubMed] [Google Scholar]
- Rygus T., Hillen W. Catabolite repression of the xyl operon in Bacillus megaterium. J Bacteriol. 1992 May;174(9):3049–3055. doi: 10.1128/jb.174.9.3049-3055.1992. [DOI] [PMC free article] [PubMed] [Google Scholar]
- Saier M. H., Jr, Novotny M. J., Comeau-Fuhrman D., Osumi T., Desai J. D. Cooperative binding of the sugar substrates and allosteric regulatory protein (enzyme IIIGlc of the phosphotransferase system) to the lactose and melibiose permeases in Escherichia coli and Salmonella typhimurium. J Bacteriol. 1983 Sep;155(3):1351–1357. doi: 10.1128/jb.155.3.1351-1357.1983. [DOI] [PMC free article] [PubMed] [Google Scholar]
- Saier M. H., Jr Protein phosphorylation and allosteric control of inducer exclusion and catabolite repression by the bacterial phosphoenolpyruvate: sugar phosphotransferase system. Microbiol Rev. 1989 Mar;53(1):109–120. doi: 10.1128/mr.53.1.109-120.1989. [DOI] [PMC free article] [PubMed] [Google Scholar]
- Saier M. H., Jr, Reizer J. Proposed uniform nomenclature for the proteins and protein domains of the bacterial phosphoenolpyruvate: sugar phosphotransferase system. J Bacteriol. 1992 Mar;174(5):1433–1438. doi: 10.1128/jb.174.5.1433-1438.1992. [DOI] [PMC free article] [PubMed] [Google Scholar]
- Saier M. H., Jr, Roseman S. Sugar transport. The crr mutation: its effect on repression of enzyme synthesis. J Biol Chem. 1976 Nov 10;251(21):6598–6605. [PubMed] [Google Scholar]
- Saier M. H., Jr, Schmidt M. R., Lin P. Phosphoryl exchange reaction catalyzed by enzyme I of the bacterial phosphoenolpyruvate: sugar phosphotransferase system. Kinetic characterization. J Biol Chem. 1980 Sep 25;255(18):8579–8584. [PubMed] [Google Scholar]
- Saier M. H., Jr, Simoni R. D. Regulation of carbohydrate uptake in gram-positive bacteria. J Biol Chem. 1976 Feb 10;251(3):893–894. [PubMed] [Google Scholar]
- Saier M. H., Jr, Straud H., Massman L. S., Judice J. J., Newman M. J., Feucht B. U. Permease-specific mutations in Salmonella typhimurium and Escherichia coli that release the glycerol, maltose, melibiose, and lactose transport systems from regulation by the phosphoenolpyruvate:sugar phosphotransferase system. J Bacteriol. 1978 Mar;133(3):1358–1367. doi: 10.1128/jb.133.3.1358-1367.1978. [DOI] [PMC free article] [PubMed] [Google Scholar]
- Saier M. H., Jr, Yamada M., Erni B., Suda K., Lengeler J., Ebner R., Argos P., Rak B., Schnetz K., Lee C. A. Sugar permeases of the bacterial phosphoenolpyruvate-dependent phosphotransferase system: sequence comparisons. FASEB J. 1988 Mar 1;2(3):199–208. doi: 10.1096/fasebj.2.3.2832233. [DOI] [PubMed] [Google Scholar]
- Saier M. H., Roseman S. Inducer exclusion and repression of enzyme synthesis in mutants of Salmonella typhimurium defective in enzyme I of the phosphoenolpyruvate: sugar phosphotransferase system. J Biol Chem. 1972 Feb 10;247(3):972–975. [PubMed] [Google Scholar]
- Sarno M. V., Tenn L. G., Desai A., Chin A. M., Grenier F. C., Saier M. H., Jr Genetic evidence for glucitol-specific enzyme III, an essential phosphocarrier protein of the Salmonella typhimurium glucitol phosphotransferase system. J Bacteriol. 1984 Mar;157(3):953–955. doi: 10.1128/jb.157.3.953-955.1984. [DOI] [PMC free article] [PubMed] [Google Scholar]
- Sato Y., Poy F., Jacobson G. R., Kuramitsu H. K. Characterization and sequence analysis of the scrA gene encoding enzyme IIScr of the Streptococcus mutans phosphoenolpyruvate-dependent sucrose phosphotransferase system. J Bacteriol. 1989 Jan;171(1):263–271. doi: 10.1128/jb.171.1.263-271.1989. [DOI] [PMC free article] [PubMed] [Google Scholar]
- Scarborough G. A. Binding energy, conformational change, and the mechanism of transmembrane solute movements. Microbiol Rev. 1985 Sep;49(3):214–231. doi: 10.1128/mr.49.3.214-231.1985. [DOI] [PMC free article] [PubMed] [Google Scholar]
- Schaefler S. Inducible system for the utilization of beta-glucosides in Escherichia coli. I. Active transport and utilization of beta-glucosides. J Bacteriol. 1967 Jan;93(1):254–263. doi: 10.1128/jb.93.1.254-263.1967. [DOI] [PMC free article] [PubMed] [Google Scholar]
- Schmid K., Ebner R., Altenbuchner J., Schmitt R., Lengeler J. W. Plasmid-mediated sucrose metabolism in Escherichia coli K12: mapping of the scr genes of pUR400. Mol Microbiol. 1988 Jan;2(1):1–8. doi: 10.1111/j.1365-2958.1988.tb00001.x. [DOI] [PubMed] [Google Scholar]
- Schmid K., Ebner R., Jahreis K., Lengeler J. W., Titgemeyer F. A sugar-specific porin, ScrY, is involved in sucrose uptake in enteric bacteria. Mol Microbiol. 1991 Apr;5(4):941–950. doi: 10.1111/j.1365-2958.1991.tb00769.x. [DOI] [PubMed] [Google Scholar]
- Schmid K., Schupfner M., Schmitt R. Plasmid-mediated uptake and metabolism of sucrose by Escherichia coli K-12. J Bacteriol. 1982 Jul;151(1):68–76. doi: 10.1128/jb.151.1.68-76.1982. [DOI] [PMC free article] [PubMed] [Google Scholar]
- Schnetz K., Rak B. Beta-glucoside permease represses the bgl operon of Escherichia coli by phosphorylation of the antiterminator protein and also interacts with glucose-specific enzyme III, the key element in catabolite control. Proc Natl Acad Sci U S A. 1990 Jul;87(13):5074–5078. doi: 10.1073/pnas.87.13.5074. [DOI] [PMC free article] [PubMed] [Google Scholar]
- Schnetz K., Rak B. Regulation of the bgl operon of Escherichia coli by transcriptional antitermination. EMBO J. 1988 Oct;7(10):3271–3277. doi: 10.1002/j.1460-2075.1988.tb03194.x. [DOI] [PMC free article] [PubMed] [Google Scholar]
- Schnetz K., Sutrina S. L., Saier M. H., Jr, Rak B. Identification of catalytic residues in the beta-glucoside permease of Escherichia coli by site-specific mutagenesis and demonstration of interdomain cross-reactivity between the beta-glucoside and glucose systems. J Biol Chem. 1990 Aug 15;265(23):13464–13471. [PubMed] [Google Scholar]
- Schnetz K., Toloczyki C., Rak B. Beta-glucoside (bgl) operon of Escherichia coli K-12: nucleotide sequence, genetic organization, and possible evolutionary relationship to regulatory components of two Bacillus subtilis genes. J Bacteriol. 1987 Jun;169(6):2579–2590. doi: 10.1128/jb.169.6.2579-2590.1987. [DOI] [PMC free article] [PubMed] [Google Scholar]
- Schnierow B. J., Yamada M., Saier M. H., Jr Partial nucleotide sequence of the pts operon in Salmonella typhimurium: comparative analyses in five bacterial genera. Mol Microbiol. 1989 Jan;3(1):113–118. doi: 10.1111/j.1365-2958.1989.tb00110.x. [DOI] [PubMed] [Google Scholar]
- Scholte B. J., Postma P. W. Competition between two pathways for sugar uptake by the phosphoenolpyruvate-dependent sugar phosphotransferase system in Salmonella typhimurium. Eur J Biochem. 1981;114(1):51–58. doi: 10.1111/j.1432-1033.1981.tb06171.x. [DOI] [PubMed] [Google Scholar]
- Scholte B. J., Schuitema A. R., Postma P. W. Characterization of factor IIIGLc in catabolite repression-resistant (crr) mutants of Salmonella typhimurium. J Bacteriol. 1982 Feb;149(2):576–586. doi: 10.1128/jb.149.2.576-586.1982. [DOI] [PMC free article] [PubMed] [Google Scholar]
- Scholte B. J., Schuitema A. R., Postma P. W. Isolation of IIIGlc of the phosphoenolpyruvate-dependent glucose phosphotransferase system of Salmonella typhimurium. J Bacteriol. 1981 Oct;148(1):257–264. doi: 10.1128/jb.148.1.257-264.1981. [DOI] [PMC free article] [PubMed] [Google Scholar]
- Schrecker Otto, Hengstenberg Wolfgang. Purification of the lactose specific factor III of the staphylococcal PEP dependent phosphotransferase system. FEBS Lett. 1971 Mar 16;13(4):209–212. doi: 10.1016/0014-5793(71)80537-9. [DOI] [PubMed] [Google Scholar]
- Sharma S., Georges F., Delbaere L. T., Lee J. S., Klevit R. E., Waygood E. B. Epitope mapping by mutagenesis distinguishes between the two tertiary structures of the histidine-containing protein HPr. Proc Natl Acad Sci U S A. 1991 Jun 1;88(11):4877–4881. doi: 10.1073/pnas.88.11.4877. [DOI] [PMC free article] [PubMed] [Google Scholar]
- Shimotsu H., Henner D. J. Modulation of Bacillus subtilis levansucrase gene expression by sucrose and regulation of the steady-state mRNA level by sacU and sacQ genes. J Bacteriol. 1986 Oct;168(1):380–388. doi: 10.1128/jb.168.1.380-388.1986. [DOI] [PMC free article] [PubMed] [Google Scholar]
- Shioi J. I., Galloway R. J., Niwano M., Chinnock R. E., Taylor B. L. Requirement of ATP in bacterial chemotaxis. J Biol Chem. 1982 Jul 25;257(14):7969–7975. [PubMed] [Google Scholar]
- Shuman H. A. The use of gene fusions of study bacterial transport proteins. J Membr Biol. 1981;61(1):1–11. doi: 10.1007/BF01870747. [DOI] [PubMed] [Google Scholar]
- Simoni R. D., Hays J. B., Nakazawa T., Roseman S. Sugar transport. VI. Phosphoryl transfer in the lactose phosphotransferase system of Staphylococcus aureus. J Biol Chem. 1973 Feb 10;248(3):957–965. [PubMed] [Google Scholar]
- Simoni R. D., Nakazawa T., Hays J. B., Roseman S. Sugar transport. IV. Isolation and characterization of the lactose phosphotransferase system in Staphylococcus aureus. J Biol Chem. 1973 Feb 10;248(3):932–940. [PubMed] [Google Scholar]
- Simoni R. D., Roseman S., Saier M. H., Jr Sugar transport. Properties of mutant bacteria defective in proteins of the phosphoenolpyruvate: sugar phosphotransferase system. J Biol Chem. 1976 Nov 10;251(21):6584–6597. [PubMed] [Google Scholar]
- Simoni R. D., Roseman S. Sugar transport. VII. Lactose transport in Staphylococcus aureus. J Biol Chem. 1973 Feb 10;248(3):966–974. [PubMed] [Google Scholar]
- Singh S. P., Bishop C. J., Vink R., Rogers P. J. Regulation of the glucose phosphotransferase system in Brochothrix thermosphacta by membrane energization. J Bacteriol. 1985 Oct;164(1):367–378. doi: 10.1128/jb.164.1.367-378.1985. [DOI] [PMC free article] [PubMed] [Google Scholar]
- Sizemore C., Wieland B., Götz F., Hillen W. Regulation of Staphylococcus xylosus xylose utilization genes at the molecular level. J Bacteriol. 1992 May;174(9):3042–3048. doi: 10.1128/jb.174.9.3042-3048.1992. [DOI] [PMC free article] [PubMed] [Google Scholar]
- Solomon E., Lin E. C. Mutations affecting the dissimilation of mannitol by Escherichia coli K-12. J Bacteriol. 1972 Aug;111(2):566–574. doi: 10.1128/jb.111.2.566-574.1972. [DOI] [PMC free article] [PubMed] [Google Scholar]
- Solomon E., Miyal K., Lin E. C. Membrane translocation of mannitol in Escherichia coli without phosphorylation. J Bacteriol. 1973 May;114(2):723–728. doi: 10.1128/jb.114.2.723-728.1973. [DOI] [PMC free article] [PubMed] [Google Scholar]
- Sprenger G. A., Lengeler J. W. Analysis of sucrose catabolism in Klebsiella pneumoniae and in Scr+ derivatives of Escherichia coli K12. J Gen Microbiol. 1988 Jun;134(6):1635–1644. doi: 10.1099/00221287-134-6-1635. [DOI] [PubMed] [Google Scholar]
- Sprenger G. A., Lengeler J. W. L-Sorbose metabolism in Klebsiella pneumoniae and Sor+ derivatives of Escherichia coli K-12 and chemotaxis toward sorbose. J Bacteriol. 1984 Jan;157(1):39–45. doi: 10.1128/jb.157.1.39-45.1984. [DOI] [PMC free article] [PubMed] [Google Scholar]
- Sprenger G. A., Lengeler J. W. Mapping of the sor genes for L-sorbose degradation in the chromosome of Klebsiella pneumoniae. Mol Gen Genet. 1987 Sep;209(2):352–359. doi: 10.1007/BF00329665. [DOI] [PubMed] [Google Scholar]
- Stein J. M., Kornberg H. L., Martin B. R. Effects of GTP,GDP[beta S] and glucose on adenylate cyclase activity of E. coli B. FEBS Lett. 1985 Mar 25;182(2):429–434. doi: 10.1016/0014-5793(85)80348-3. [DOI] [PubMed] [Google Scholar]
- Steinmetz M., Le Coq D., Aymerich S., Gonzy-Tréboul G., Gay P. The DNA sequence of the gene for the secreted Bacillus subtilis enzyme levansucrase and its genetic control sites. Mol Gen Genet. 1985;200(2):220–228. doi: 10.1007/BF00425427. [DOI] [PubMed] [Google Scholar]
- Steinmetz M., Le Coq D., Aymerich S. Induction of saccharolytic enzymes by sucrose in Bacillus subtilis: evidence for two partially interchangeable regulatory pathways. J Bacteriol. 1989 Mar;171(3):1519–1523. doi: 10.1128/jb.171.3.1519-1523.1989. [DOI] [PMC free article] [PubMed] [Google Scholar]
- Stephan M. M., Jacobson G. R. Membrane disposition of the Escherichia coli mannitol permease: identification of membrane-bound and cytoplasmic domains. Biochemistry. 1986 Dec 16;25(25):8230–8234. doi: 10.1021/bi00373a016. [DOI] [PubMed] [Google Scholar]
- Stephan M. M., Jacobson G. R. Subunit interactions of the Escherichia coli mannitol permease: correlation with enzymic activities. Biochemistry. 1986 Jul 15;25(14):4046–4051. doi: 10.1021/bi00362a009. [DOI] [PubMed] [Google Scholar]
- Stephan M. M., Khandekar S. S., Jacobson G. R. Hydrophilic C-terminal domain of the Escherichia coli mannitol permease: phosphorylation, functional independence, and evidence for intersubunit phosphotransfer. Biochemistry. 1989 Sep 19;28(19):7941–7946. doi: 10.1021/bi00445a058. [DOI] [PubMed] [Google Scholar]
- Stock J. B., Ninfa A. J., Stock A. M. Protein phosphorylation and regulation of adaptive responses in bacteria. Microbiol Rev. 1989 Dec;53(4):450–490. doi: 10.1128/mr.53.4.450-490.1989. [DOI] [PMC free article] [PubMed] [Google Scholar]
- Stock J. B., Waygood E. B., Meadow N. D., Postma P. W., Roseman S. Sugar transport by the bacterial phosphotransferase system. The glucose receptors of the Salmonella typhimurium phosphotransferase system. J Biol Chem. 1982 Dec 10;257(23):14543–14552. [PubMed] [Google Scholar]
- Stone M. J., Fairbrother W. J., Palmer A. G., 3rd, Reizer J., Saier M. H., Jr, Wright P. E. Backbone dynamics of the Bacillus subtilis glucose permease IIA domain determined from 15N NMR relaxation measurements. Biochemistry. 1992 May 12;31(18):4394–4406. doi: 10.1021/bi00133a003. [DOI] [PubMed] [Google Scholar]
- Stüber K., Deutscher J., Sobek H. M., Hengstenberg W., Beyreuther K. Amino acid sequence of the amphiphilic phosphocarrier protein factor IIILac of the lactose-specific phosphotransferase system of Staphylococcus. Biochemistry. 1985 Feb 26;24(5):1164–1168. doi: 10.1021/bi00326a016. [DOI] [PubMed] [Google Scholar]
- Sugiyama J. E., Mahmoodian S., Jacobson G. R. Membrane topology analysis of Escherichia coli mannitol permease by using a nested-deletion method to create mtlA-phoA fusions. Proc Natl Acad Sci U S A. 1991 Nov 1;88(21):9603–9607. doi: 10.1073/pnas.88.21.9603. [DOI] [PMC free article] [PubMed] [Google Scholar]
- Sutrina S. L., Chin A. M., Esch F., Saier M. H., Jr Purification and characterization of the fructose-inducible HPr-like protein, FPr, and the fructose-specific enzyme III of the phosphoenolpyruvate: sugar phosphotransferase system of Salmonella typhimurium. J Biol Chem. 1988 Apr 15;263(11):5061–5069. [PubMed] [Google Scholar]
- Sutrina S. L., Reddy P., Saier M. H., Jr, Reizer J. The glucose permease of Bacillus subtilis is a single polypeptide chain that functions to energize the sucrose permease. J Biol Chem. 1990 Oct 25;265(30):18581–18589. [PubMed] [Google Scholar]
- Sutrina S. L., Reizer J., Saier M. H., Jr Inducer expulsion in Streptococcus pyogenes: properties and mechanism of the efflux reaction. J Bacteriol. 1988 Apr;170(4):1874–1877. doi: 10.1128/jb.170.4.1874-1877.1988. [DOI] [PMC free article] [PubMed] [Google Scholar]
- Sutrina S. L., Waygood E. B., Grenier F. C., Saier M. H., Jr HPr/HPr-P phosphoryl exchange reaction catalyzed by the mannitol specific enzyme II of the bacterial phosphotransferase system. J Biol Chem. 1987 Feb 25;262(6):2636–2641. [PubMed] [Google Scholar]
- Takahashi M., Blazy B., Baudras A., Hillen W. Ligand-modulated binding of a gene regulatory protein to DNA. Quantitative analysis of cyclic-AMP induced binding of CRP from Escherichia coli to non-specific and specific DNA targets. J Mol Biol. 1989 Jun 20;207(4):783–796. doi: 10.1016/0022-2836(89)90244-1. [DOI] [PubMed] [Google Scholar]
- Tangney M., Buchanan C. J., Priest F. G., Mitchell W. J. Maltose uptake and its regulation in Bacillus subtilis. FEMS Microbiol Lett. 1992 Oct 1;76(1-2):191–196. doi: 10.1016/0378-1097(92)90385-2. [DOI] [PubMed] [Google Scholar]
- Thibault L., Vadeboncoeur C. Phosphoenolpyruvate-sugar phosphotransferase transport system of Streptococcus mutans: purification of HPr and enzyme I and determination of their intracellular concentrations by rocket immunoelectrophoresis. Infect Immun. 1985 Dec;50(3):817–825. doi: 10.1128/iai.50.3.817-825.1985. [DOI] [PMC free article] [PubMed] [Google Scholar]
- Thompson J., Chassy B. M. Intracellular hexose-6-phosphate:phosphohydrolase from Streptococcus lactis: purification, properties, and function. J Bacteriol. 1983 Oct;156(1):70–80. doi: 10.1128/jb.156.1.70-80.1983. [DOI] [PMC free article] [PubMed] [Google Scholar]
- Thompson J., Nguyen N. Y., Sackett D. L., Donkersloot J. A. Transposon-encoded sucrose metabolism in Lactococcus lactis. Purification of sucrose-6-phosphate hydrolase and genetic linkage to N5-(L-1-carboxyethyl)-L-ornithine synthase in strain K1. J Biol Chem. 1991 Aug 5;266(22):14573–14579. [PubMed] [Google Scholar]
- Thompson J., Sackett D. L., Donkersloot J. A. Purification and properties of fructokinase I from Lactococcus lactis. Localization of scrK on the sucrose-nisin transposon Tn5306. J Biol Chem. 1991 Nov 25;266(33):22626–22633. [PubMed] [Google Scholar]
- Thompson J., Torchia D. A. Use of 31P nuclear magnetic resonance spectroscopy and 14C fluorography in studies of glycolysis and regulation of pyruvate kinase in Streptococcus lactis. J Bacteriol. 1984 Jun;158(3):791–800. doi: 10.1128/jb.158.3.791-800.1984. [DOI] [PMC free article] [PubMed] [Google Scholar]
- Thompson J., Turner K. W., Thomas T. D. Catabolite inhibition and sequential metabolism of sugars by Streptococcus lactis. J Bacteriol. 1978 Mar;133(3):1163–1174. doi: 10.1128/jb.133.3.1163-1174.1978. [DOI] [PMC free article] [PubMed] [Google Scholar]
- Titgemeyer F., Eisermann R., Hengstenberg W., Lengeler J. W. The nucleotide sequence of ptsH gene from Klebsiella pneumoniae. Nucleic Acids Res. 1990 Apr 11;18(7):1898–1898. doi: 10.1093/nar/18.7.1898. [DOI] [PMC free article] [PubMed] [Google Scholar]
- Tribhuwan R. C., Johnson M. S., Taylor B. L. Evidence against direct involvement of cyclic GMP or cyclic AMP in bacterial chemotactic signaling. J Bacteriol. 1986 Nov;168(2):624–630. doi: 10.1128/jb.168.2.624-630.1986. [DOI] [PMC free article] [PubMed] [Google Scholar]
- Ugurbil K., Rottenberg H., Glynn P., Shulman R. G. 31P nuclear magnetic resonance studies of bioenergetics and glycolysis in anaerobic Escherichia coli cells. Proc Natl Acad Sci U S A. 1978 May;75(5):2244–2248. doi: 10.1073/pnas.75.5.2244. [DOI] [PMC free article] [PubMed] [Google Scholar]
- Ullah A. H., Cirillo V. P. Mycoplasma phosphoenolpyruvate-dependent sugar phosphotransferase system: purification and characterization of the phosphocarrier protein. J Bacteriol. 1976 Sep;127(3):1298–1306. doi: 10.1128/jb.127.3.1298-1306.1976. [DOI] [PMC free article] [PubMed] [Google Scholar]
- Vadeboncoeur C., Brochu D., Reizer J. Quantitative determination of the intracellular concentration of the various forms of HPr, a phosphocarrier protein of the phosphoenolpyruvate: sugar phosphotransferase system in growing cells of oral streptococci. Anal Biochem. 1991 Jul;196(1):24–30. doi: 10.1016/0003-2697(91)90112-7. [DOI] [PubMed] [Google Scholar]
- Vadeboncoeur C., Gauthier L. The phosphoenolpyruvate: sugar phosphotransferase system of Streptococcus salivarius. Identification of a IIIman protein. Can J Microbiol. 1987 Feb;33(2):118–122. doi: 10.1139/m87-020. [DOI] [PubMed] [Google Scholar]
- Vadeboncoeur C., Proulx M. Lactose transport in Streptococcus mutans: isolation and characterization of factor IIIlac, a specific protein component of the phosphoenolpyruvate-lactose phosphotransferase system. Infect Immun. 1984 Oct;46(1):213–219. doi: 10.1128/iai.46.1.213-219.1984. [DOI] [PMC free article] [PubMed] [Google Scholar]
- Vadeboncoeur C., Proulx M., Trahan L. Purification of proteins similar to HPr and enzyme I from the oral bacterium Streptococcus salivarius. Biochemical and immunochemical properties. Can J Microbiol. 1983 Dec;29(12):1694–1705. doi: 10.1139/m83-260. [DOI] [PubMed] [Google Scholar]
- Van Dijk A. A., Scheek R. M., Dijkstra K., Wolters G. K., Robillard G. T. Characterization of the protonation and hydrogen bonding state of the histidine residues in IIAmtl, a domain of the phosphoenolpyruvate-dependent mannitol-specific transport protein. Biochemistry. 1992 Sep 22;31(37):9063–9072. doi: 10.1021/bi00152a050. [DOI] [PubMed] [Google Scholar]
- Vogler A. P., Broekhuizen C. P., Schuitema A., Lengeler J. W., Postma P. W. Suppression of IIIGlc-defects by enzymes IINag and IIBgl of the PEP:carbohydrate phosphotransferase system. Mol Microbiol. 1988 Nov;2(6):719–726. doi: 10.1111/j.1365-2958.1988.tb00082.x. [DOI] [PubMed] [Google Scholar]
- Vogler A. P., Lengeler J. W. Analysis of the nag regulon from Escherichia coli K12 and Klebsiella pneumoniae and of its regulation. Mol Gen Genet. 1989 Oct;219(1-2):97–105. doi: 10.1007/BF00261163. [DOI] [PubMed] [Google Scholar]
- Vogler A. P., Lengeler J. W. Comparison of the sequences of the nagE operons from Klebsiella pneumoniae and Escherichia coli K12: enhanced variability of the enzyme IIN-acetylglucosamine in regions connecting functional domains. Mol Gen Genet. 1991 Nov;230(1-2):270–276. doi: 10.1007/BF00290677. [DOI] [PubMed] [Google Scholar]
- Vogler A. P., Lengeler J. W. Complementation of a truncated membrane-bound Enzyme IINag from Klebsiella pneumoniae with a soluble Enzyme III in Escherichia coli K12. Mol Gen Genet. 1988 Jul;213(1):175–178. doi: 10.1007/BF00333417. [DOI] [PubMed] [Google Scholar]
- Vogler A. P., Lengeler J. W. Indirect role of adenylate cyclase and cyclic AMP in chemotaxis to phosphotransferase system carbohydrates in Escherichia coli K-12. J Bacteriol. 1987 Feb;169(2):593–599. doi: 10.1128/jb.169.2.593-599.1987. [DOI] [PMC free article] [PubMed] [Google Scholar]
- Vogler A. P., Trentmann S., Lengeler J. W. Alternative route for biosynthesis of amino sugars in Escherichia coli K-12 mutants by means of a catabolic isomerase. J Bacteriol. 1989 Dec;171(12):6586–6592. doi: 10.1128/jb.171.12.6586-6592.1989. [DOI] [PMC free article] [PubMed] [Google Scholar]
- Waygood E. B. Enzyme I of the phosphoenolpyruvate: sugar phosphotransferase system has two sites of phosphorylation per dimer. Biochemistry. 1986 Jul 15;25(14):4085–4090. doi: 10.1021/bi00362a015. [DOI] [PubMed] [Google Scholar]
- Waygood E. B., Mattoo R. L., Erickson E., Vadeboncoeur C. Phosphoproteins and the phosphoenolpyruvate:sugar phosphotransferase system of Streptococcus salivarius. Detection of two different ATP-dependent phosphorylations of the phosphocarrier protein HPr. Can J Microbiol. 1986 Apr;32(4):310–318. doi: 10.1139/m86-062. [DOI] [PubMed] [Google Scholar]
- Waygood E. B., Mattoo R. L., Peri K. G. Phosphoproteins and the phosphoenolpyruvate: sugar phosphotransferase system in Salmonella typhimurium and Escherichia coli: evidence for IIImannose, IIIfructose, IIIglucitol, and the phosphorylation of enzyme IImannitol and enzyme IIN-acetylglucosamine. J Cell Biochem. 1984;25(3):139–159. doi: 10.1002/jcb.240250304. [DOI] [PubMed] [Google Scholar]
- Waygood E. B., Meadow N. D., Roseman S. Modified assay procedures for the phosphotransferase system in enteric bacteria. Anal Biochem. 1979 May;95(1):293–304. doi: 10.1016/0003-2697(79)90219-7. [DOI] [PubMed] [Google Scholar]
- Waygood E. B. Resolution of the phosphoenolpyruvate: fructose phosphotransferase system of Escherichia coli into two components: enzyme IIfructose and fructose-induced HPr-like protein (FPr). Can J Biochem. 1980 Oct;58(10):1144–1146. doi: 10.1139/o80-153. [DOI] [PubMed] [Google Scholar]
- Waygood E. B., Steeves T. Enzyme I of the phosphoenolpyruvate: sugar phosphotransferase system of Escherichia coli. Purification to homogeneity and some properties. Can J Biochem. 1980 Jan;58(1):40–48. doi: 10.1139/o80-006. [DOI] [PubMed] [Google Scholar]
- Wehmeier U., Sprenger G. A., Lengeler J. W. The use of lambda plac-Mu hybrid phages in Klebsiella pneumoniae and the isolation of stable Hfr strains. Mol Gen Genet. 1989 Feb;215(3):529–536. doi: 10.1007/BF00427052. [DOI] [PubMed] [Google Scholar]
- Weickert M. J., Adhya S. A family of bacterial regulators homologous to Gal and Lac repressors. J Biol Chem. 1992 Aug 5;267(22):15869–15874. [PubMed] [Google Scholar]
- Weickert M. J., Chambliss G. H. Site-directed mutagenesis of a catabolite repression operator sequence in Bacillus subtilis. Proc Natl Acad Sci U S A. 1990 Aug;87(16):6238–6242. doi: 10.1073/pnas.87.16.6238. [DOI] [PMC free article] [PubMed] [Google Scholar]
- Weigel N., Kukuruzinska M. A., Nakazawa A., Waygood E. B., Roseman S. Sugar transport by the bacterial phosphotransferase system. Phosphoryl transfer reactions catalyzed by enzyme I of Salmonella typhimurium. J Biol Chem. 1982 Dec 10;257(23):14477–14491. [PubMed] [Google Scholar]
- Weigel N., Powers D. A., Roseman S. Sugar transport by the bacterial phosphotransferase system. Primary structure and active site of a general phosphocarrier protein (HPr) from Salmonella typhimurium. J Biol Chem. 1982 Dec 10;257(23):14499–14509. [PubMed] [Google Scholar]
- Weigel N., Waygood E. B., Kukuruzinska M. A., Nakazawa A., Roseman S. Sugar transport by the bacterial phosphotransferase system. Isolation and characterization of enzyme I from Salmonella typhimurium. J Biol Chem. 1982 Dec 10;257(23):14461–14469. [PubMed] [Google Scholar]
- Weng Q. P., Elder J., Jacobson G. R. Site-specific mutagenesis of residues in the Escherichia coli mannitol permease that have been suggested to be important for its phosphorylation and chemoreception functions. J Biol Chem. 1992 Sep 25;267(27):19529–19535. [PubMed] [Google Scholar]
- White D. W., Jacobson G. R. Molecular cloning of the C-terminal domain of Escherichia coli D-mannitol permease: expression, phosphorylation, and complementation with C-terminal permease deletion proteins. J Bacteriol. 1990 Mar;172(3):1509–1515. doi: 10.1128/jb.172.3.1509-1515.1990. [DOI] [PMC free article] [PubMed] [Google Scholar]
- White R. J. The role of the phosphoenolpyruvate phosphotransferase system in the transport of N-acetyl-D-glucosamine by Escherichia coli. Biochem J. 1970 Jun;118(1):89–92. doi: 10.1042/bj1180089. [DOI] [PMC free article] [PubMed] [Google Scholar]
- Williams N., Fox D. K., Shea C., Roseman S. Pel, the protein that permits lambda DNA penetration of Escherichia coli, is encoded by a gene in ptsM and is required for mannose utilization by the phosphotransferase system. Proc Natl Acad Sci U S A. 1986 Dec;83(23):8934–8938. doi: 10.1073/pnas.83.23.8934. [DOI] [PMC free article] [PubMed] [Google Scholar]
- Wilson T. H., Yunker P. L., Hansen C. L. Lactose transport mutants of Escherichia coli resistant to inhibition by the phosphotransferase system. Biochim Biophys Acta. 1990 Nov 2;1029(1):113–116. doi: 10.1016/0005-2736(90)90443-r. [DOI] [PubMed] [Google Scholar]
- Winkler H. H. Efflux and the steady state in alpha-methylglucoside transport in Escherichia coli. J Bacteriol. 1971 May;106(2):362–368. doi: 10.1128/jb.106.2.362-368.1971. [DOI] [PMC free article] [PubMed] [Google Scholar]
- Winkler H. H., Wilson T. H. Inhibition of beta-galactoside transport by substrates of the glucose transport system in Escherichia coli. Biochim Biophys Acta. 1967;135(5):1030–1051. doi: 10.1016/0005-2736(67)90073-9. [DOI] [PubMed] [Google Scholar]
- Wittekind M., Reizer J., Deutscher J., Saier M. H., Klevit R. E. Common structural changes accompany the functional inactivation of HPr by seryl phosphorylation or by serine to aspartate substitution. Biochemistry. 1989 Dec 26;28(26):9908–9912. doi: 10.1021/bi00452a005. [DOI] [PubMed] [Google Scholar]
- Wittekind M., Reizer J., Klevit R. E. Sequence-specific 1H NMR resonance assignments of Bacillus subtilis HPr: use of spectra obtained from mutants to resolve spectral overlap. Biochemistry. 1990 Aug 7;29(31):7191–7200. doi: 10.1021/bi00483a006. [DOI] [PubMed] [Google Scholar]
- Wolfe A. J., Conley M. P., Berg H. C. Acetyladenylate plays a role in controlling the direction of flagellar rotation. Proc Natl Acad Sci U S A. 1988 Sep;85(18):6711–6715. doi: 10.1073/pnas.85.18.6711. [DOI] [PMC free article] [PubMed] [Google Scholar]
- Woodward M. J., Charles H. P. Genes for l-sorbose utilization in Escherichia coli. J Gen Microbiol. 1982 Sep;128(9):1969–1980. doi: 10.1099/00221287-128-9-1969. [DOI] [PubMed] [Google Scholar]
- Wootton J. C., Drummond M. H. The Q-linker: a class of interdomain sequences found in bacterial multidomain regulatory proteins. Protein Eng. 1989 May;2(7):535–543. doi: 10.1093/protein/2.7.535. [DOI] [PubMed] [Google Scholar]
- Worthylake D., Meadow N. D., Roseman S., Liao D. I., Herzberg O., Remington S. J. Three-dimensional structure of the Escherichia coli phosphocarrier protein IIIglc. Proc Natl Acad Sci U S A. 1991 Dec 1;88(23):10382–10386. doi: 10.1073/pnas.88.23.10382. [DOI] [PMC free article] [PubMed] [Google Scholar]
- Wu L. F., Saier M. H., Jr Nucleotide sequence of the fruA gene, encoding the fructose permease of the Rhodobacter capsulatus phosphotransferase system, and analyses of the deduced protein sequence. J Bacteriol. 1990 Dec;172(12):7167–7178. doi: 10.1128/jb.172.12.7167-7178.1990. [DOI] [PMC free article] [PubMed] [Google Scholar]
- Wu L. F., Tomich J. M., Saier M. H., Jr Structure and evolution of a multidomain multiphosphoryl transfer protein. Nucleotide sequence of the fruB(HI) gene in Rhodobacter capsulatus and comparisons with homologous genes from other organisms. J Mol Biol. 1990 Jun 20;213(4):687–703. doi: 10.1016/S0022-2836(05)80256-6. [DOI] [PubMed] [Google Scholar]
- Wöhrl B. M., Lengeler J. W. Cloning and physical mapping of the sor genes for L-sorbose transport and metabolism from Klebsiella pneumoniae. Mol Microbiol. 1990 Sep;4(9):1557–1565. doi: 10.1111/j.1365-2958.1990.tb02067.x. [DOI] [PubMed] [Google Scholar]
- Wöhrl B. M., Wehmeier U. F., Lengeler J. W. Positive and negative regulation of expression of the L-sorbose (sor) operon by SorC in Klebsiella pneumoniae. Mol Gen Genet. 1990 Nov;224(2):193–200. doi: 10.1007/BF00271552. [DOI] [PubMed] [Google Scholar]
- Yamada M., Feucht B. U., Saier M. H., Jr Regulation of gluconeogenesis by the glucitol enzyme III of the phosphotransferase system in Escherichia coli. J Bacteriol. 1987 Dec;169(12):5416–5422. doi: 10.1128/jb.169.12.5416-5422.1987. [DOI] [PMC free article] [PubMed] [Google Scholar]
- Yamada M., Saier M. H., Jr Glucitol-specific enzymes of the phosphotransferase system in Escherichia coli. Nucleotide sequence of the gut operon. J Biol Chem. 1987 Apr 25;262(12):5455–5463. [PubMed] [Google Scholar]
- Yamada M., Saier M. H., Jr Physical and genetic characterization of the glucitol operon in Escherichia coli. J Bacteriol. 1987 Jul;169(7):2990–2994. doi: 10.1128/jb.169.7.2990-2994.1987. [DOI] [PMC free article] [PubMed] [Google Scholar]
- Yamada M., Saier M. H., Jr Positive and negative regulators for glucitol (gut) operon expression in Escherichia coli. J Mol Biol. 1988 Oct 5;203(3):569–583. doi: 10.1016/0022-2836(88)90193-3. [DOI] [PubMed] [Google Scholar]
- Yamada Y., Chang Y. Y., Daniels G. A., Wu L. F., Tomich J. M., Yamada M., Saier M. H., Jr Insertion of the mannitol permease into the membrane of Escherichia coli. Possible involvement of an N-terminal amphiphilic sequence. J Biol Chem. 1991 Sep 25;266(27):17863–17871. [PubMed] [Google Scholar]
- Yang J. K., Bloom R. W., Epstein W. Catabolite and transient repression in Escherichia coli do not require enzyme I of the phosphotransferase system. J Bacteriol. 1979 Apr;138(1):275–279. doi: 10.1128/jb.138.1.275-279.1979. [DOI] [PMC free article] [PubMed] [Google Scholar]
- Zagorec M., Postma P. W. Cloning and nucleotide sequence of the ptsG gene of Bacillus subtilis. Mol Gen Genet. 1992 Aug;234(2):325–328. doi: 10.1007/BF00283853. [DOI] [PubMed] [Google Scholar]
- Zeng G. Q., De Reuse H., Danchin A. Mutational analysis of the enzyme IIIGlc of the phosphoenolpyruvate phosphotransferase system in Escherichia coli. Res Microbiol. 1992 Mar-Apr;143(3):251–261. doi: 10.1016/0923-2508(92)90017-i. [DOI] [PubMed] [Google Scholar]
- Zukowski M. M., Miller L., Cosgwell P., Chen K., Aymerich S., Steinmetz M. Nucleotide sequence of the sacS locus of Bacillus subtilis reveals the presence of two regulatory genes. Gene. 1990 May 31;90(1):153–155. doi: 10.1016/0378-1119(90)90453-x. [DOI] [PubMed] [Google Scholar]
- de Boer M., Broekhuizen C. P., Postma P. W. Regulation of glycerol kinase by enzyme IIIGlc of the phosphoenolpyruvate:carbohydrate phosphotransferase system. J Bacteriol. 1986 Jul;167(1):393–395. doi: 10.1128/jb.167.1.393-395.1986. [DOI] [PMC free article] [PubMed] [Google Scholar]
- de Crécy-Lagard V., Bouvet O. M., Lejeune P., Danchin A. Fructose catabolism in Xanthomonas campestris pv. campestris. Sequence of the PTS operon, characterization of the fructose-specific enzymes. J Biol Chem. 1991 Sep 25;266(27):18154–18161. [PubMed] [Google Scholar]
- de Crécy-Lagard V., Lejeune P., Bouvet O. M., Danchin A. Identification of two fructose transport and phosphorylation pathways in Xanthomonas campestris pv. campestris. Mol Gen Genet. 1991 Jul;227(3):465–472. doi: 10.1007/BF00273939. [DOI] [PubMed] [Google Scholar]
- de Vos W. M., Boerrigter I., van Rooyen R. J., Reiche B., Hengstenberg W. Characterization of the lactose-specific enzymes of the phosphotransferase system in Lactococcus lactis. J Biol Chem. 1990 Dec 25;265(36):22554–22560. [PubMed] [Google Scholar]
- den Blaauwen J. L., Postma P. W. Regulation of cyclic AMP synthesis by enzyme IIIGlc of the phosphoenolpyruvate:sugar phosphotransferase system in crp strains of Salmonella typhimurium. J Bacteriol. 1985 Oct;164(1):477–478. doi: 10.1128/jb.164.1.477-478.1985. [DOI] [PMC free article] [PubMed] [Google Scholar]
- el Hassouni M., Henrissat B., Chippaux M., Barras F. Nucleotide sequences of the arb genes, which control beta-glucoside utilization in Erwinia chrysanthemi: comparison with the Escherichia coli bgl operon and evidence for a new beta-glycohydrolase family including enzymes from eubacteria, archeabacteria, and humans. J Bacteriol. 1992 Feb;174(3):765–777. doi: 10.1128/jb.174.3.765-777.1992. [DOI] [PMC free article] [PubMed] [Google Scholar]
- el-Kabbani O. A., Waygood E. B., Delbaere L. T. Tertiary structure of histidine-containing protein of the phosphoenolpyruvate:sugar phosphotransferase system of Escherichia coli. J Biol Chem. 1987 Sep 25;262(27):12926–12929. [PubMed] [Google Scholar]
- van Dijk A. A., Eisermann R., Hengstenberg W., Robillard G. T. Exchange of phosphoryl groups between HPr molecules of the phosphoenolpyruvate-dependent phosphotransferase system is an autocatalytic process. Biochemistry. 1991 Mar 19;30(11):2876–2882. doi: 10.1021/bi00225a021. [DOI] [PubMed] [Google Scholar]
- van Dijk A. A., de Lange L. C., Bachovchin W. W., Robillard G. T. Effect of phosphorylation on hydrogen-bonding interactions of the active site histidine of the phosphocarrier protein HPr of the phosphoenolpyruvate-dependent phosphotransferase system determined by 15N NMR spectroscopy. Biochemistry. 1990 Sep 4;29(35):8164–8171. doi: 10.1021/bi00487a026. [DOI] [PubMed] [Google Scholar]
- van Nuland N. A., van Dijk A. A., Dijkstra K., van Hoesel F. H., Scheek R. M., Robillard G. T. Three-dimensional 15N-1H-1H and 15N-13C-1H nuclear-magnetic resonance studies of HPr a central component of the phosphoenolpyruvate-dependent phosphotransferase system from Escherichia coli. Assignment of backbone resonances. Eur J Biochem. 1992 Feb 1;203(3):483–491. doi: 10.1111/j.1432-1033.1992.tb16573.x. [DOI] [PubMed] [Google Scholar]
- van Rooijen R. J., de Vos W. M. Molecular cloning, transcriptional analysis, and nucleotide sequence of lacR, a gene encoding the repressor of the lactose phosphotransferase system of Lactococcus lactis. J Biol Chem. 1990 Oct 25;265(30):18499–18503. [PubMed] [Google Scholar]
- van Rooijen R. J., van Schalkwijk S., de Vos W. M. Molecular cloning, characterization, and nucleotide sequence of the tagatose 6-phosphate pathway gene cluster of the lactose operon of Lactococcus lactis. J Biol Chem. 1991 Apr 15;266(11):7176–7181. [PubMed] [Google Scholar]
- van Weeghel R. P., Keck W., Robillard G. T. Regulated high-level expression of the mannitol permease of the phosphoenolpyruvate-dependent sugar phosphotransferase system in Escherichia coli. Proc Natl Acad Sci U S A. 1990 Apr;87(7):2613–2617. doi: 10.1073/pnas.87.7.2613. [DOI] [PMC free article] [PubMed] [Google Scholar]
- van Weeghel R. P., Meyer G. H., Keck W., Robillard G. T. Phosphoenolpyruvate-dependent mannitol phosphotransferase system of Escherichia coli: overexpression, purification, and characterization of the enzymatically active C-terminal domain of enzyme IImtl equivalent to enzyme IIImtl. Biochemistry. 1991 Feb 19;30(7):1774–1779. doi: 10.1021/bi00221a007. [DOI] [PubMed] [Google Scholar]
- van Weeghel R. P., Meyer G., Pas H. H., Keck W., Robillard G. T. Cytoplasmic phosphorylating domain of the mannitol-specific transport protein of the phosphoenolpyruvate-dependent phosphotransferase system in Escherichia coli: overexpression, purification, and functional complementation with the mannitol binding domain. Biochemistry. 1991 Oct 1;30(39):9478–9485. doi: 10.1021/bi00103a013. [DOI] [PubMed] [Google Scholar]
- van Weeghel R. P., van der Hoek Y. Y., Pas H. H., Elferink M., Keck W., Robillard G. T. Details of mannitol transport in Escherichia coli elucidated by site-specific mutagenesis and complementation of phosphorylation site mutants of the phosphoenolpyruvate-dependent mannitol-specific phosphotransferase system. Biochemistry. 1991 Feb 19;30(7):1768–1773. doi: 10.1021/bi00221a006. [DOI] [PubMed] [Google Scholar]