Full text
PDF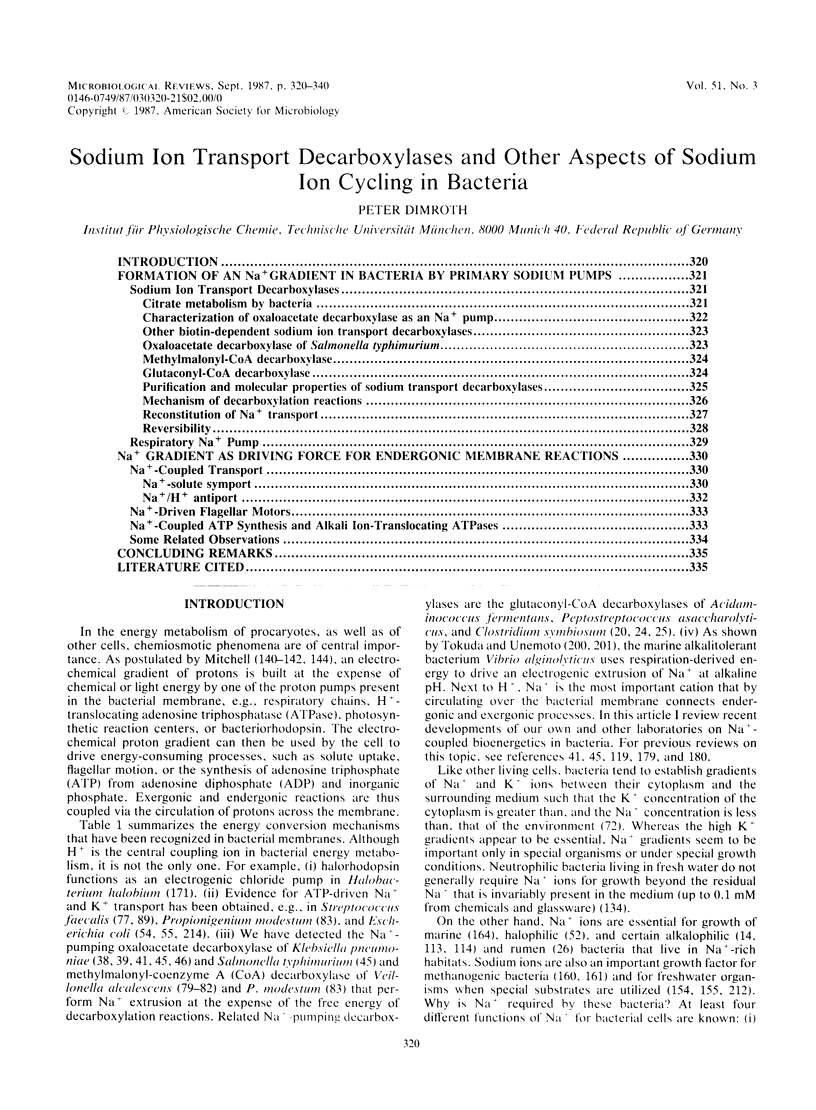
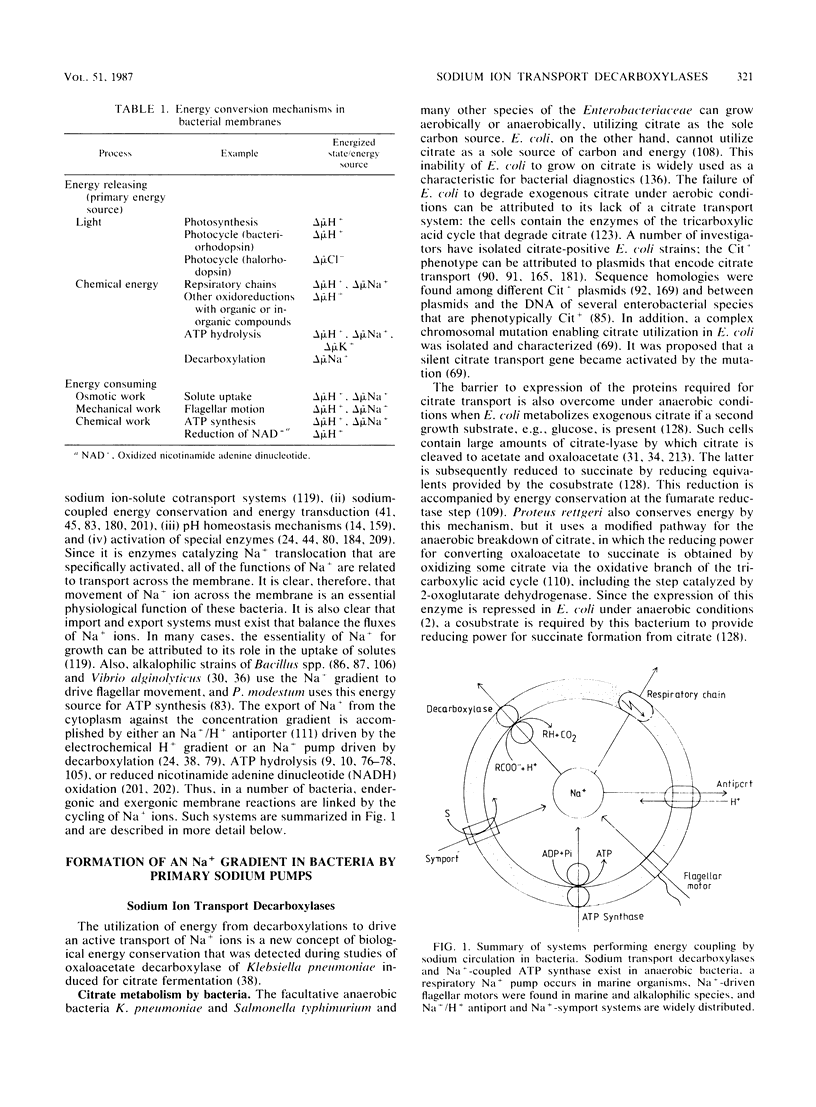
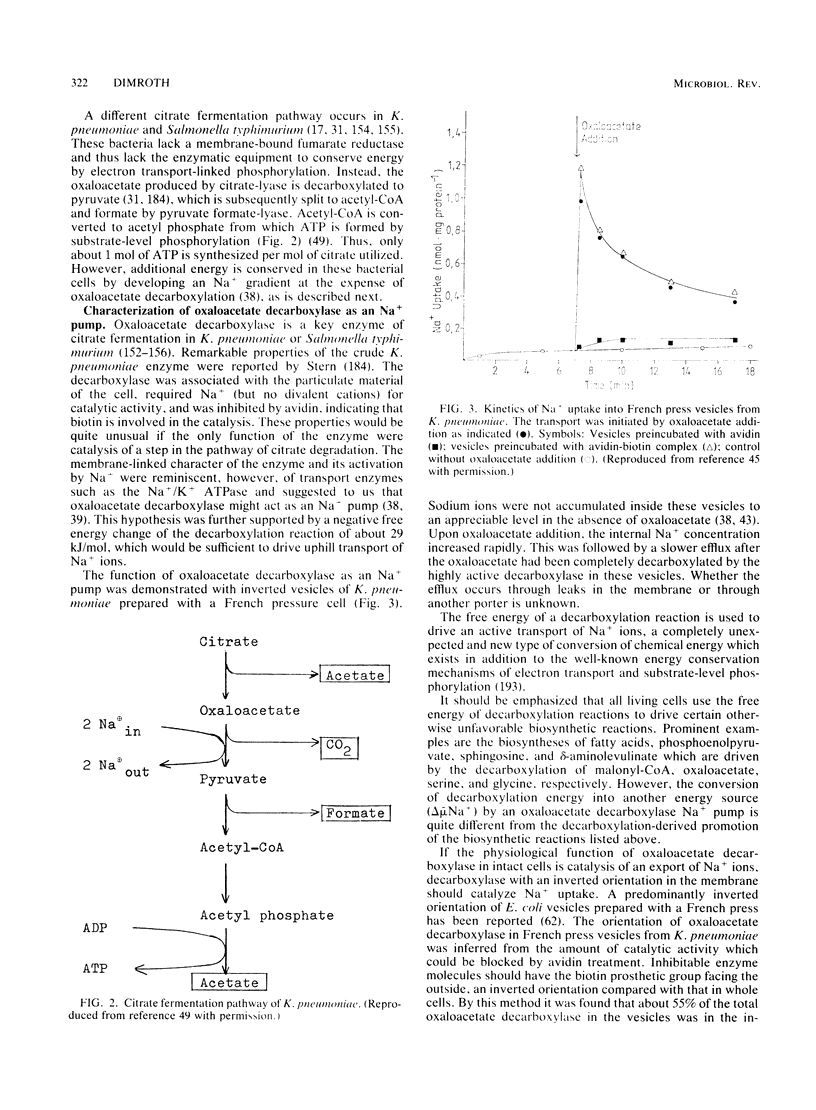
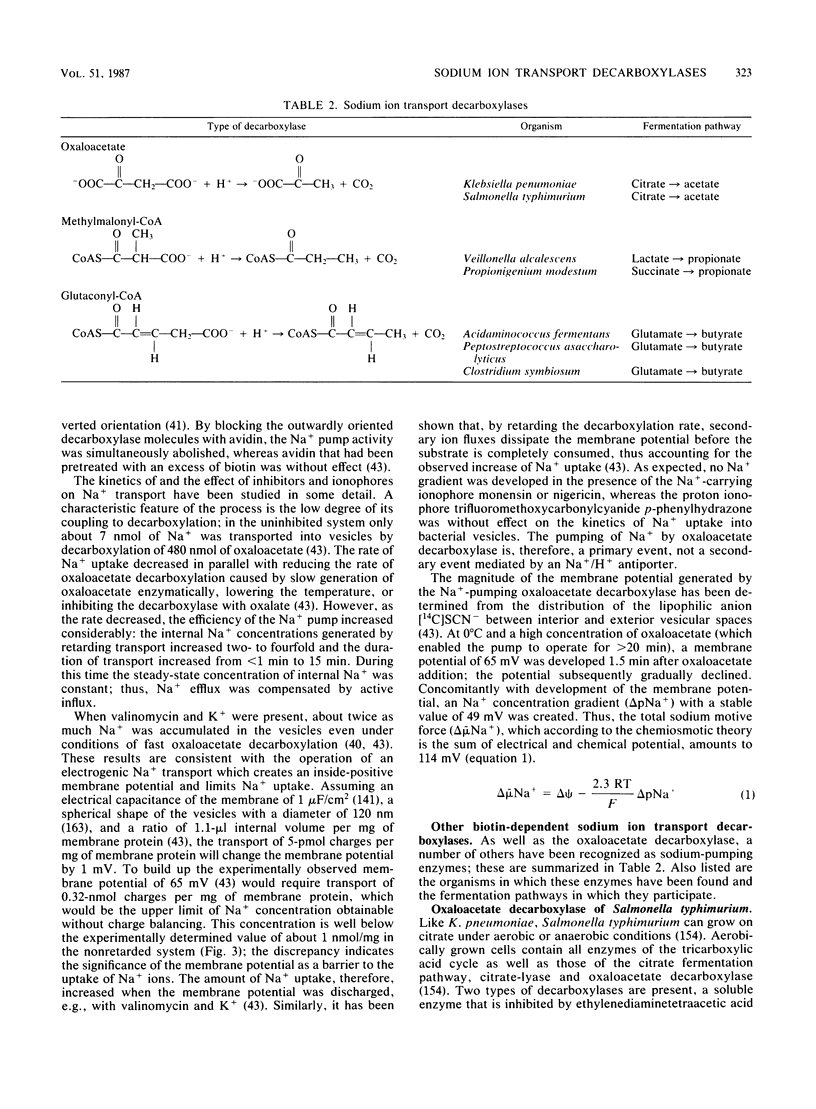
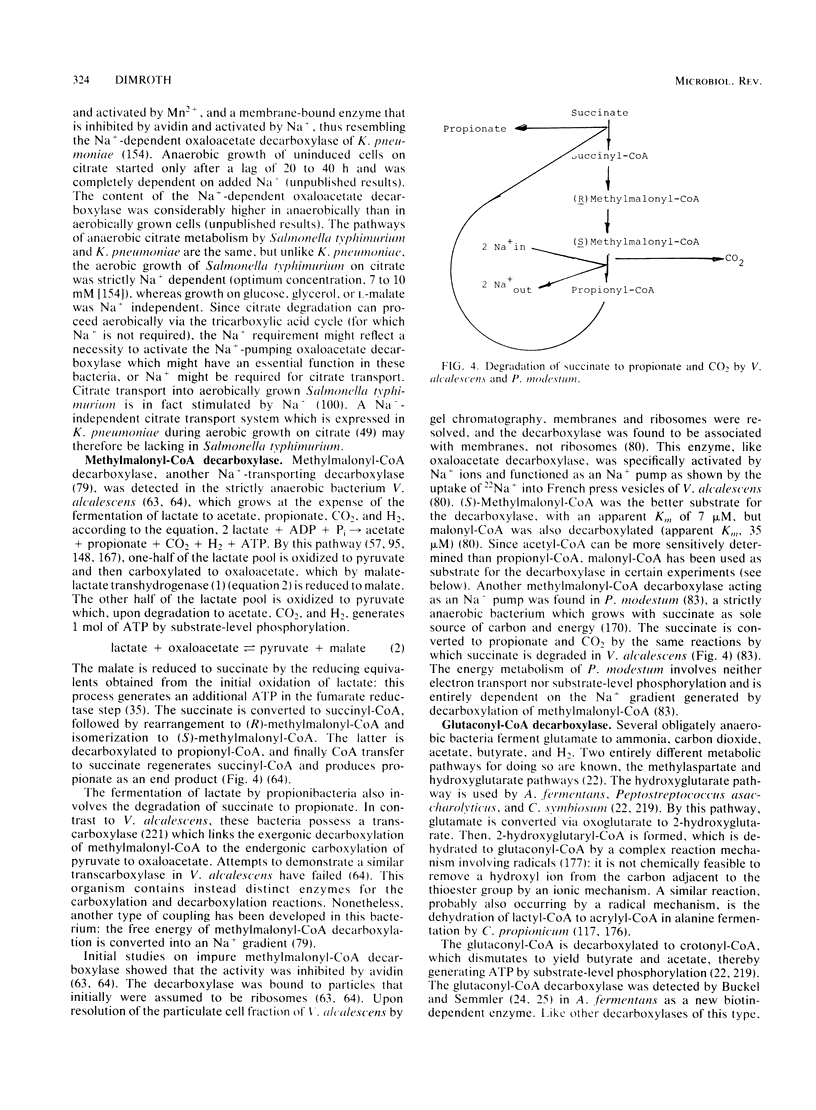
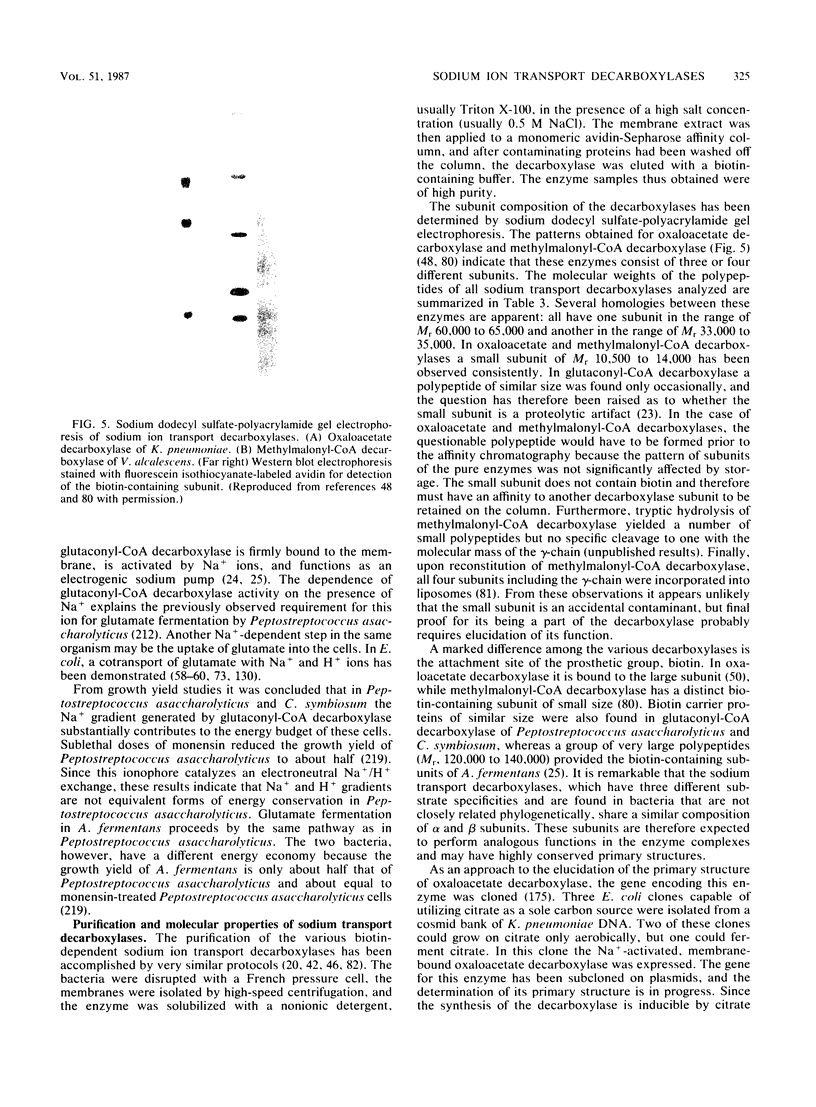
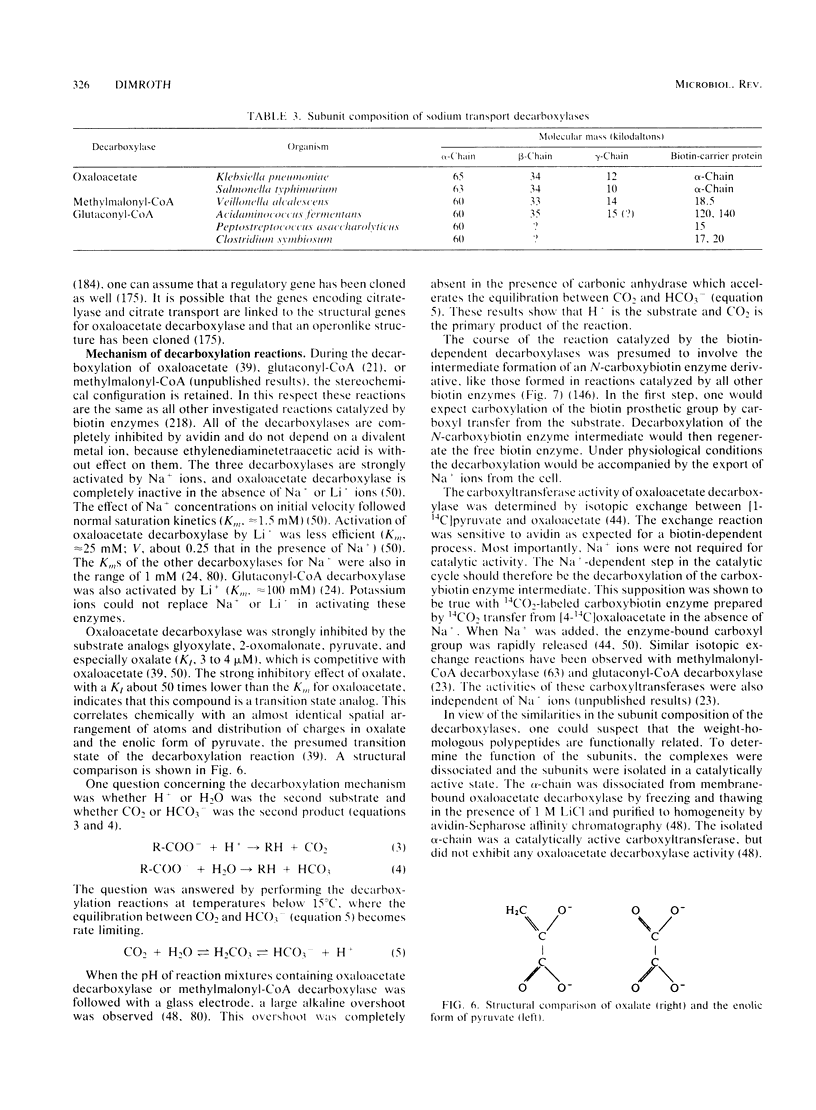
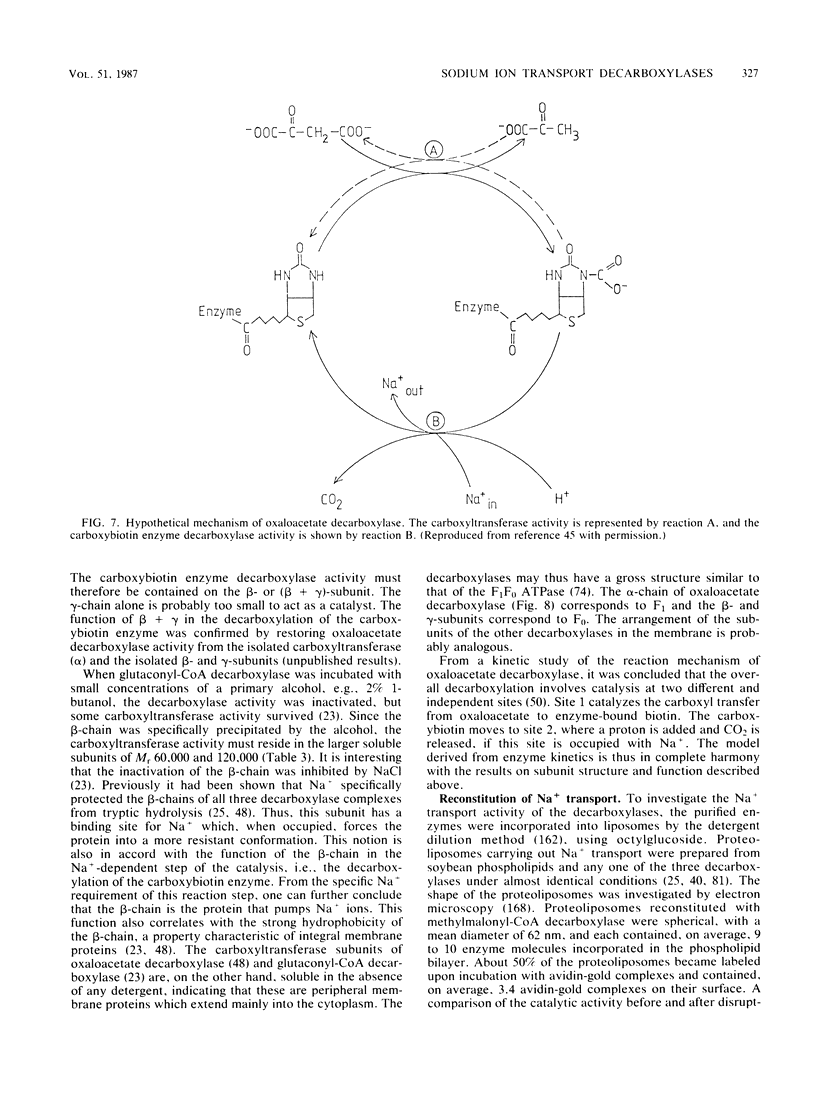
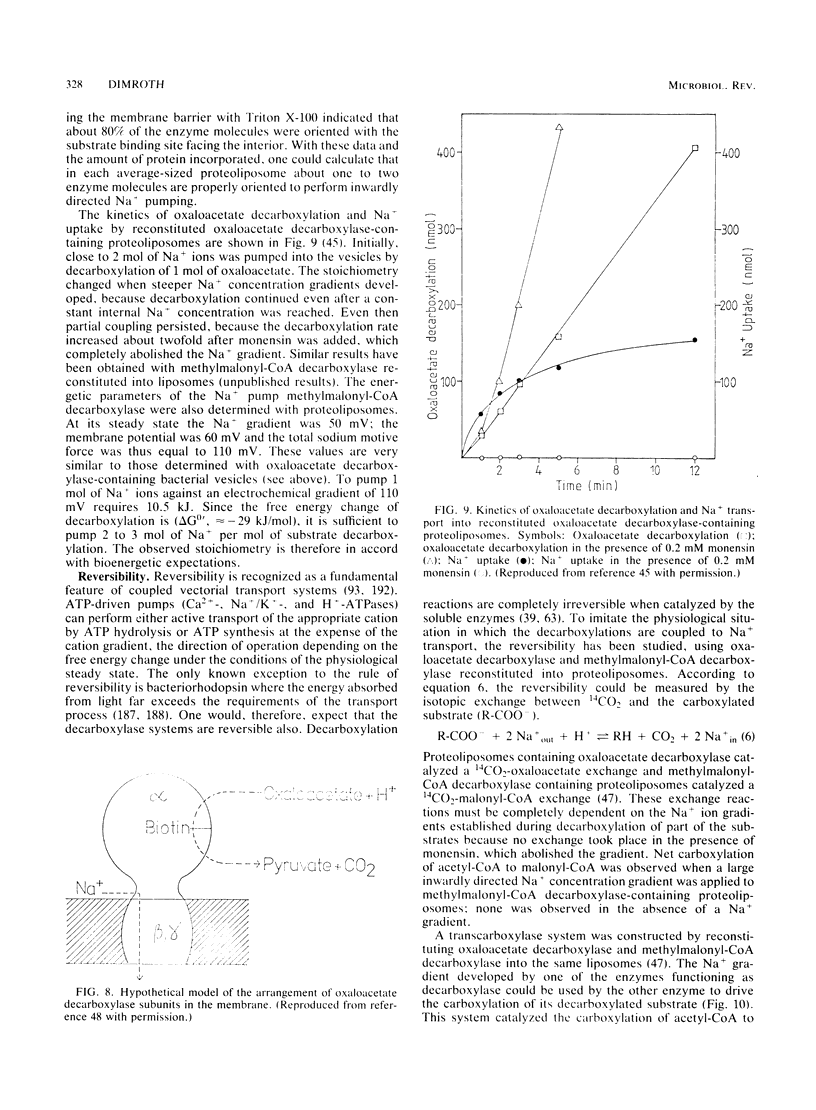
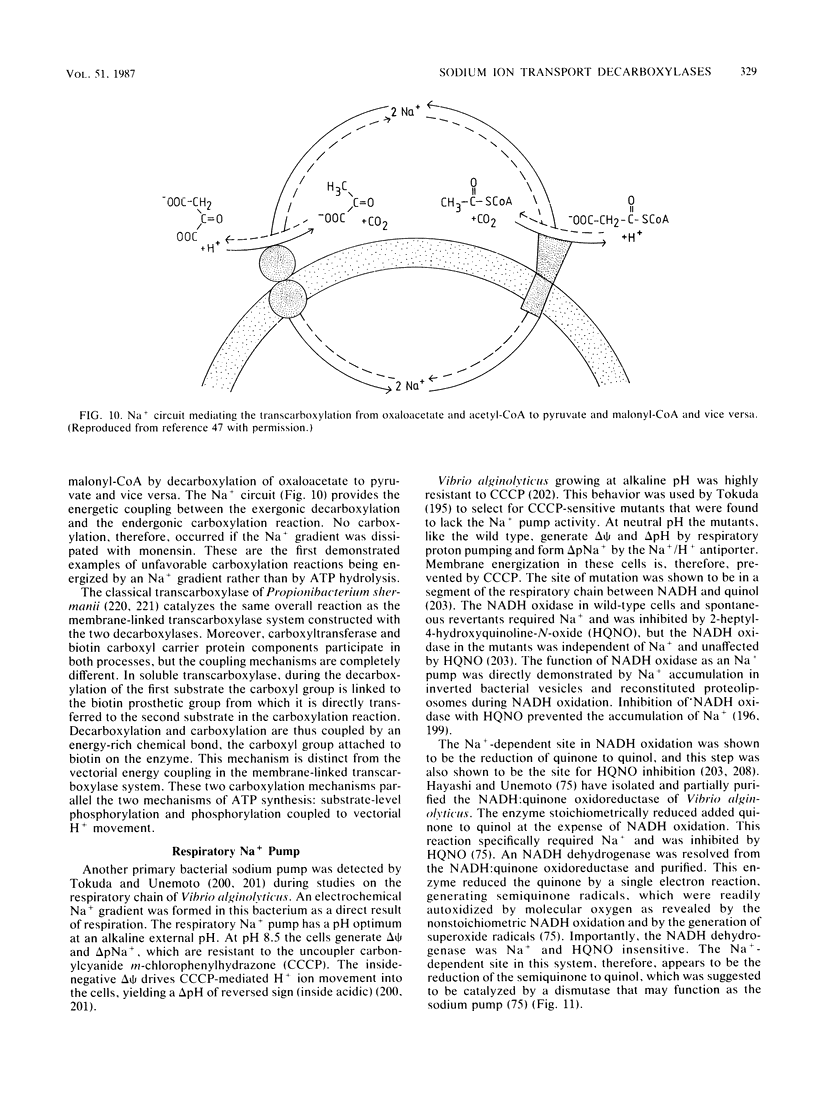
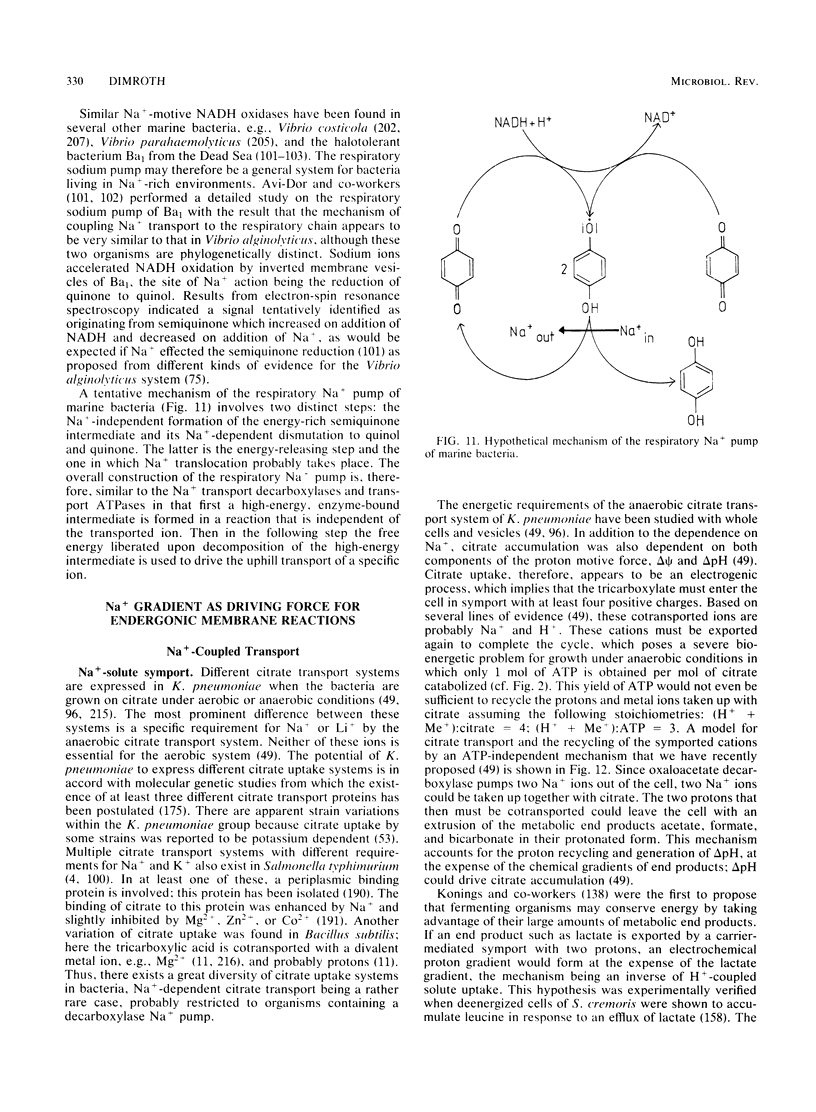
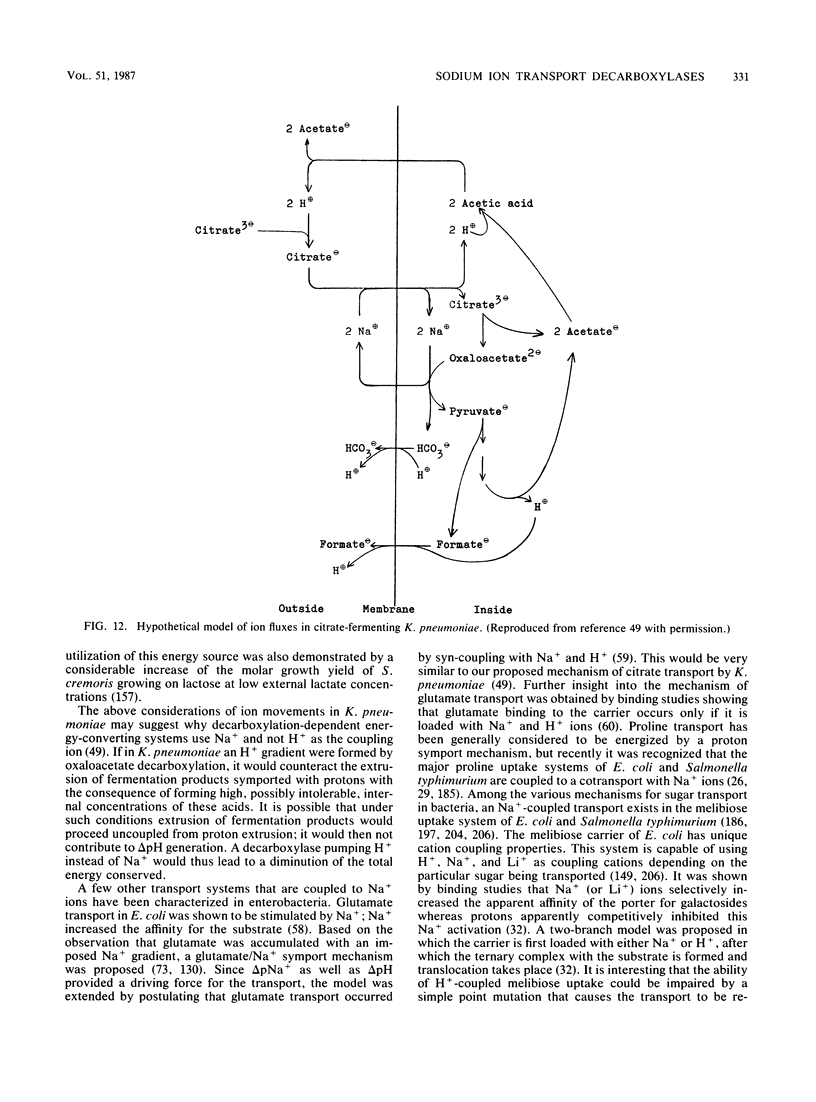
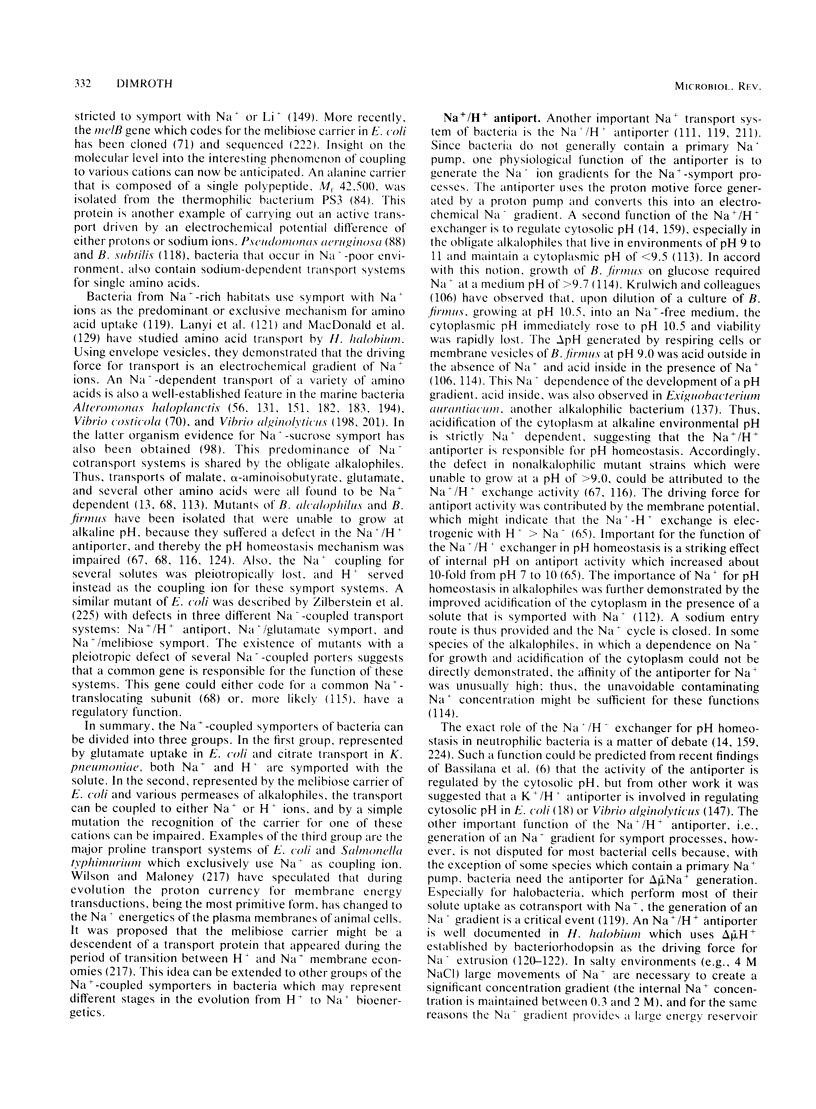
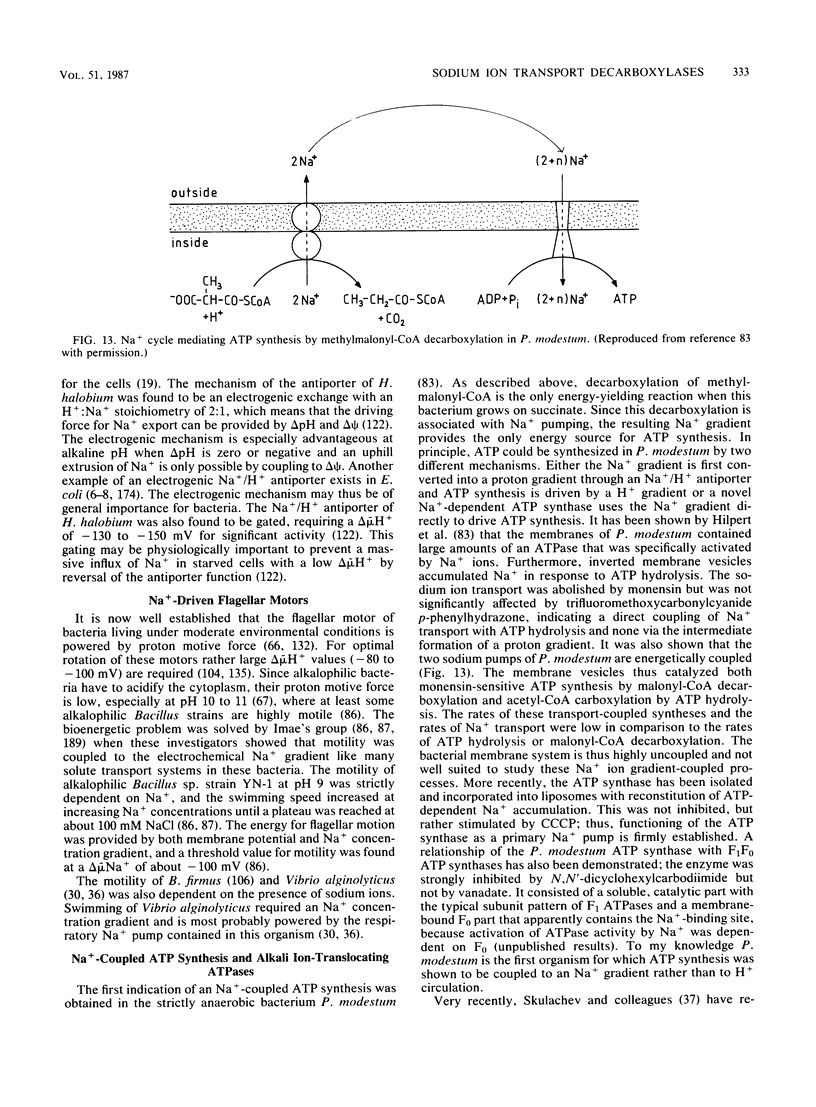
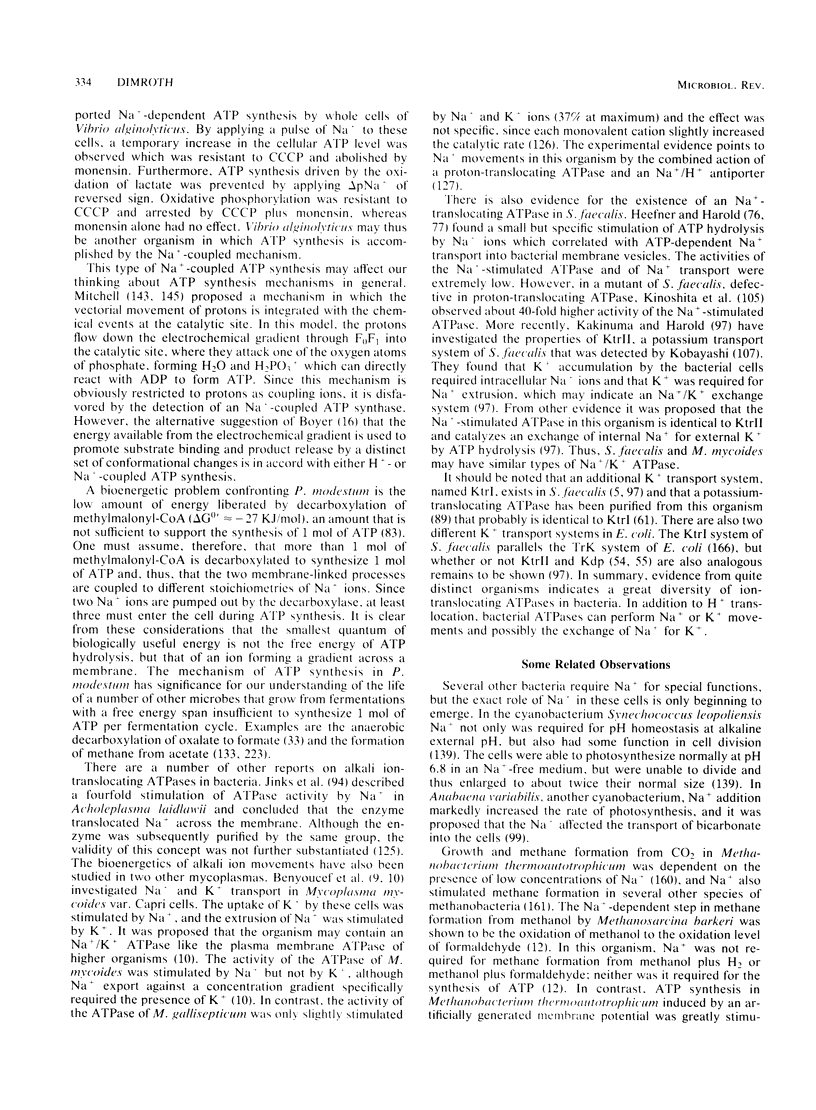
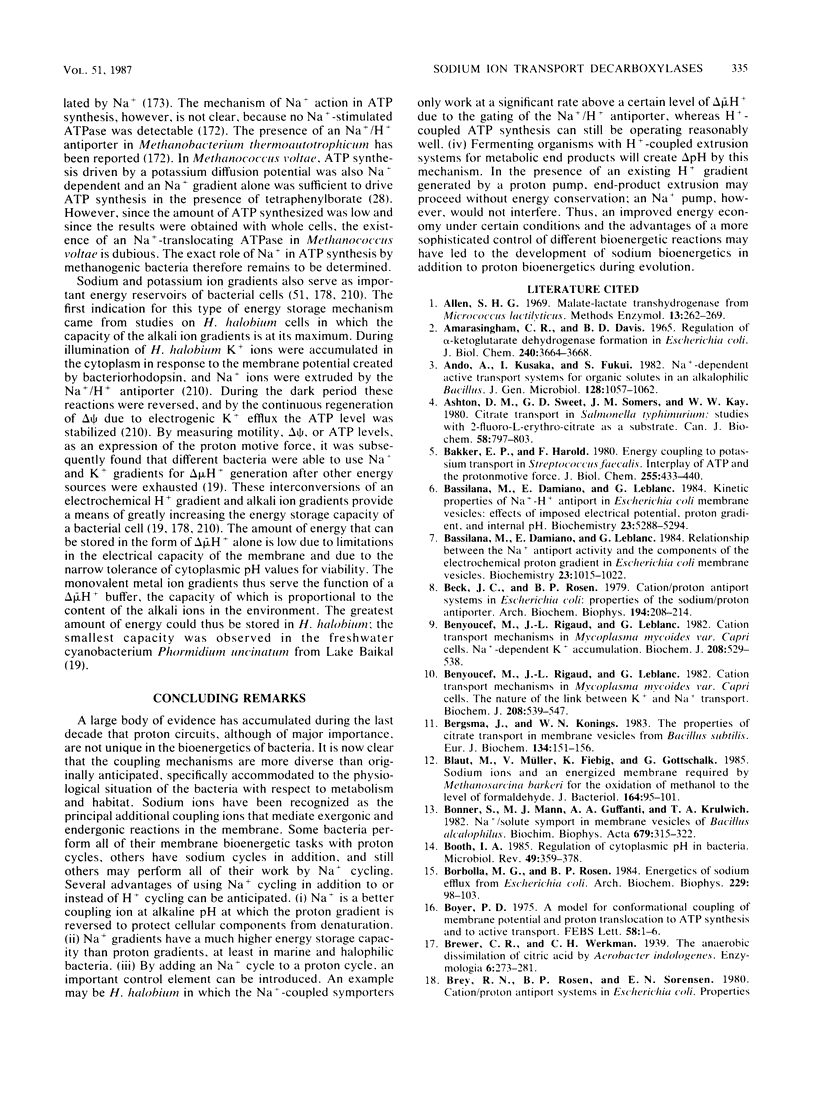
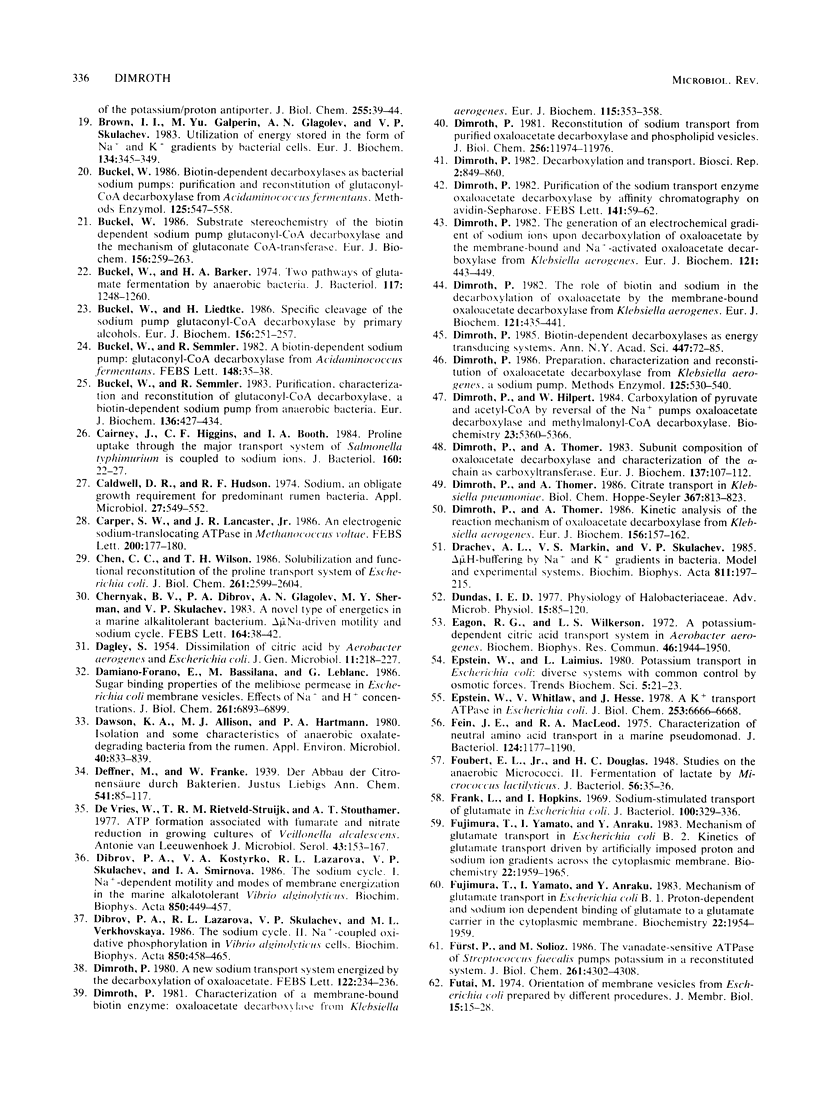
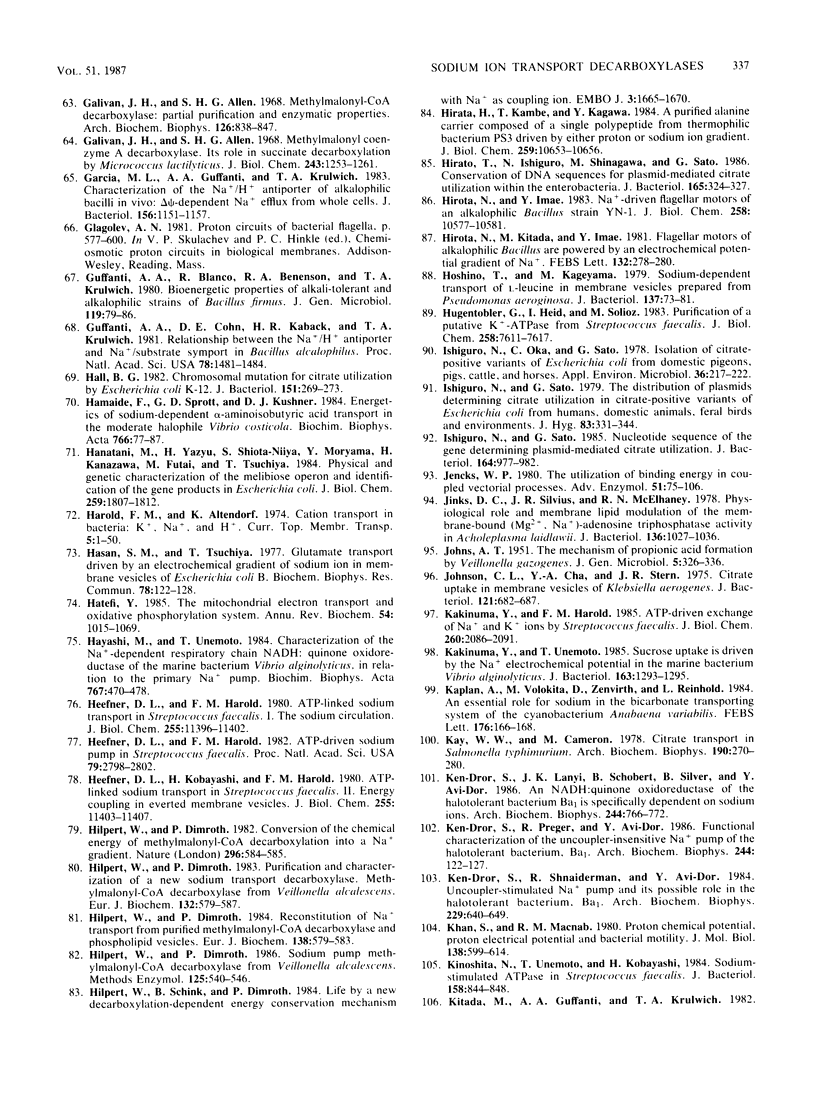
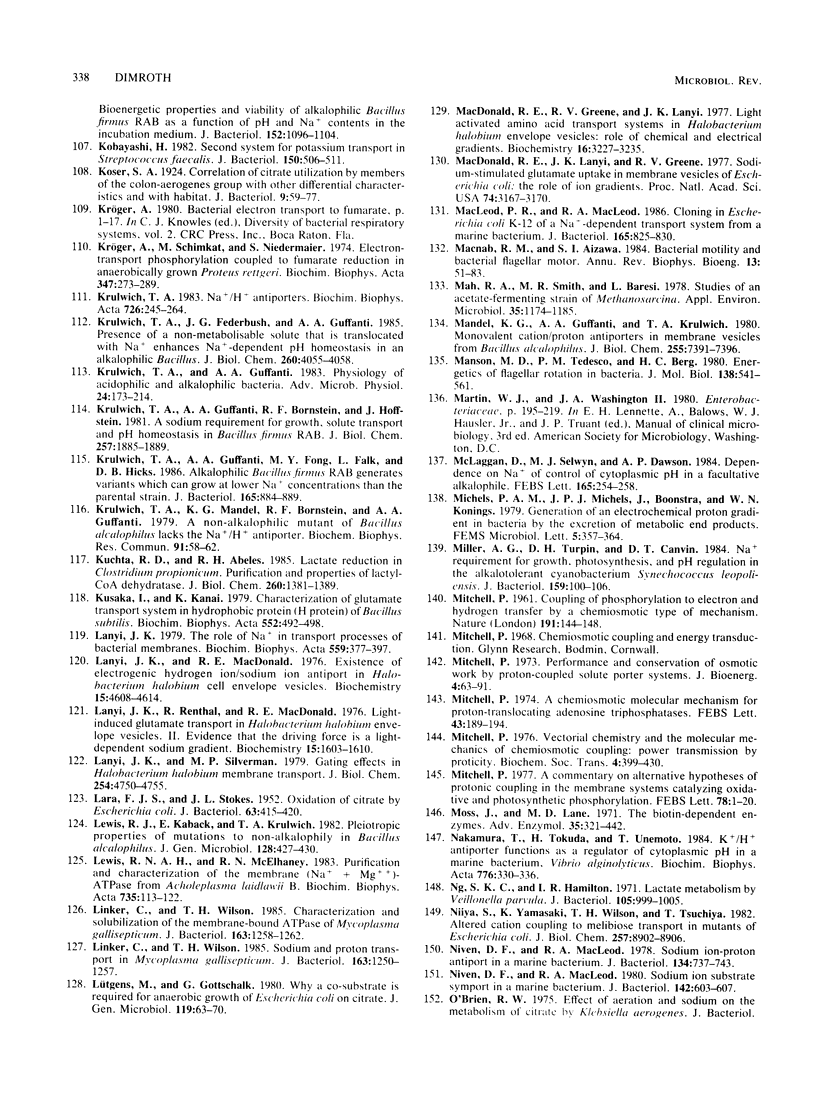
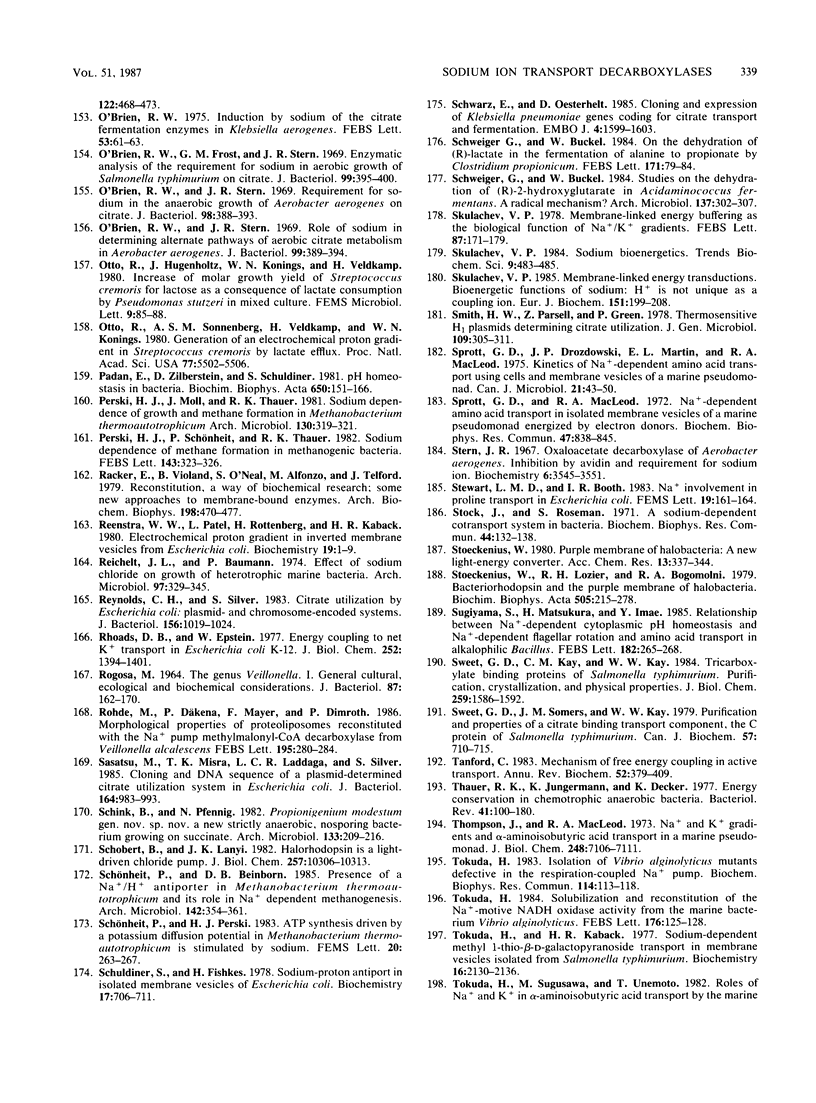
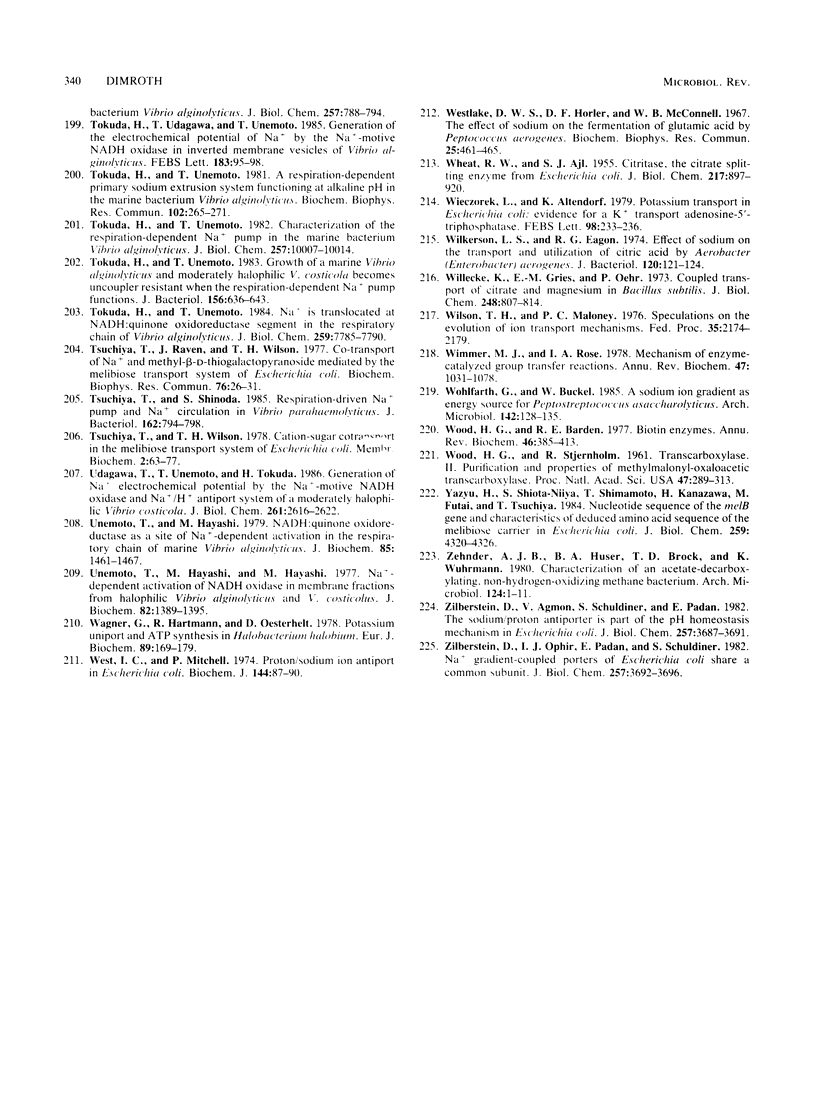
Images in this article
Selected References
These references are in PubMed. This may not be the complete list of references from this article.
- Amarasingham C. R., Davis B. D. Regulation of alpha-ketoglutarate dehydrogenase formation in Escherichia coli. J Biol Chem. 1965 Sep;240(9):3664–3668. [PubMed] [Google Scholar]
- Ashton D. M., Sweet G. D., Somers J. M., Kay W. W. Citrate transport in Salmonella typhimurium: studies with 2-fluoro-L-erythro-citrate as a substrate. Can J Biochem. 1980 Oct;58(10):797–803. doi: 10.1139/o80-111. [DOI] [PubMed] [Google Scholar]
- Bakker E. P., Harold F. M. Energy coupling to potassium transport in Streptococcus faecalis. Interplay of ATP and the protonmotive force. J Biol Chem. 1980 Jan 25;255(2):433–440. [PubMed] [Google Scholar]
- Bassilana M., Damiano E., Leblanc G. Relationships between the Na+-H+ antiport activity and the components of the electrochemical proton gradient in Escherichia coli membrane vesicles. Biochemistry. 1984 Feb 28;23(5):1015–1022. doi: 10.1021/bi00300a033. [DOI] [PubMed] [Google Scholar]
- Beck J. C., Rosen B. P. Cation/proton antiport systems in escherichia coli: properties of the sodium/proton antiporter. Arch Biochem Biophys. 1979 Apr 15;194(1):208–214. doi: 10.1016/0003-9861(79)90611-8. [DOI] [PubMed] [Google Scholar]
- Benyoucef M., Rigaud J. L., Leblanc G. Cation transport mechanisms in Mycoplasma mycoides var. Capri cells. Na+-dependent K+ accumulation. Biochem J. 1982 Dec 15;208(3):529–538. doi: 10.1042/bj2080529. [DOI] [PMC free article] [PubMed] [Google Scholar]
- Benyoucef M., Rigaud J. L., Leblanc G. Cation transport mechanisms in Mycoplasma mycoides var. Capri cells. The nature of the link between K+ and Na+ transport. Biochem J. 1982 Dec 15;208(3):539–547. doi: 10.1042/bj2080539. [DOI] [PMC free article] [PubMed] [Google Scholar]
- Bergsma J., Konings W. N. The properties of citrate transport in membrane vesicles from Bacillus subtilis. Eur J Biochem. 1983 Jul 15;134(1):151–156. doi: 10.1111/j.1432-1033.1983.tb07545.x. [DOI] [PubMed] [Google Scholar]
- Blaut M., Müller V., Fiebig K., Gottschalk G. Sodium ions and an energized membrane required by Methanosarcina barkeri for the oxidation of methanol to the level of formaldehyde. J Bacteriol. 1985 Oct;164(1):95–101. doi: 10.1128/jb.164.1.95-101.1985. [DOI] [PMC free article] [PubMed] [Google Scholar]
- Booth I. R. Regulation of cytoplasmic pH in bacteria. Microbiol Rev. 1985 Dec;49(4):359–378. doi: 10.1128/mr.49.4.359-378.1985. [DOI] [PMC free article] [PubMed] [Google Scholar]
- Borbolla M. G., Rosen B. P. Energetics of sodium efflux from Escherichia coli. Arch Biochem Biophys. 1984 Feb 15;229(1):98–103. doi: 10.1016/0003-9861(84)90134-6. [DOI] [PubMed] [Google Scholar]
- Boyer P. D. A model for conformational coupling of membrane potential and proton translocation to ATP synthesis and to active transport. FEBS Lett. 1975 Oct 15;58(1):1–6. doi: 10.1016/0014-5793(75)80212-2. [DOI] [PubMed] [Google Scholar]
- Brown I. I., Galperin MYu, Glagolev A. N., Skulachev V. P. Utilization of energy stored in the form of Na+ and K+ ion gradients by bacterial cells. Eur J Biochem. 1983 Aug 1;134(2):345–349. doi: 10.1111/j.1432-1033.1983.tb07573.x. [DOI] [PubMed] [Google Scholar]
- Buckel W., Barker H. A. Two pathways of glutamate fermentation by anaerobic bacteria. J Bacteriol. 1974 Mar;117(3):1248–1260. doi: 10.1128/jb.117.3.1248-1260.1974. [DOI] [PMC free article] [PubMed] [Google Scholar]
- Buckel W., Liedtke H. The sodium pump glutaconyl-CoA decarboxylase from Acidaminococcus fermentans. Specific cleavage by n-alkanols. Eur J Biochem. 1986 Apr 15;156(2):251–257. doi: 10.1111/j.1432-1033.1986.tb09575.x. [DOI] [PubMed] [Google Scholar]
- Buckel W., Semmler R. Purification, characterisation and reconstitution of glutaconyl-CoA decarboxylase, a biotin-dependent sodium pump from anaerobic bacteria. Eur J Biochem. 1983 Nov 2;136(2):427–434. doi: 10.1111/j.1432-1033.1983.tb07760.x. [DOI] [PubMed] [Google Scholar]
- Buckel W. Substrate stereochemistry of the biotin-dependent sodium pump glutaconyl-CoA decarboxylase from Acidaminococcus fermentans. Eur J Biochem. 1986 Apr 15;156(2):259–263. doi: 10.1111/j.1432-1033.1986.tb09576.x. [DOI] [PubMed] [Google Scholar]
- Cairney J., Higgins C. F., Booth I. R. Proline uptake through the major transport system of Salmonella typhimurium is coupled to sodium ions. J Bacteriol. 1984 Oct;160(1):22–27. doi: 10.1128/jb.160.1.22-27.1984. [DOI] [PMC free article] [PubMed] [Google Scholar]
- Caldwell D. R., Hudson R. F. Sodium, an obligate growth requirement for predominant rumen bacteria. Appl Microbiol. 1974 Mar;27(3):549–552. doi: 10.1128/am.27.3.549-552.1974. [DOI] [PMC free article] [PubMed] [Google Scholar]
- Chen C. C., Wilson T. H. Solubilization and functional reconstitution of the proline transport system of Escherichia coli. J Biol Chem. 1986 Feb 25;261(6):2599–2604. [PubMed] [Google Scholar]
- DAGLEY S. Dissimilation of citric acid by Aerobacter aerogenes and Escherichia coli. J Gen Microbiol. 1954 Oct;11(2):218–227. doi: 10.1099/00221287-11-2-218. [DOI] [PubMed] [Google Scholar]
- Damiano-Forano E., Bassilana M., Leblanc G. Sugar binding properties of the melibiose permease in Escherichia coli membrane vesicles. Effects of Na+ and H+ concentrations. J Biol Chem. 1986 May 25;261(15):6893–6899. [PubMed] [Google Scholar]
- Dawson K. A., Allison M. J., Hartman P. A. Isolation and some characteristics of anaerobic oxalate-degrading bacteria from the rumen. Appl Environ Microbiol. 1980 Oct;40(4):833–839. doi: 10.1128/aem.40.4.833-839.1980. [DOI] [PMC free article] [PubMed] [Google Scholar]
- Dibrov P. A., Kostryko V. A., Lazarova R. L., Skulachev V. P., Smirnova I. A. The sodium cycle. I. Na+-dependent motility and modes of membrane energization in the marine alkalotolerant vibrio Alginolyticus. Biochim Biophys Acta. 1986 Jul 23;850(3):449–457. doi: 10.1016/0005-2728(86)90113-1. [DOI] [PubMed] [Google Scholar]
- Dibrov P. A., Lazarova R. L., Skulachev V. P., Verkhovskaya M. L. The sodium cycle. II. Na+-coupled oxidative phosphorylation in Vibrio alginolyticus cells. Biochim Biophys Acta. 1986 Jul 23;850(3):458–465. doi: 10.1016/0005-2728(86)90114-3. [DOI] [PubMed] [Google Scholar]
- Dimroth P. A new sodium-transport system energized by the decarboxylation of oxaloacetate. FEBS Lett. 1980 Dec 29;122(2):234–236. doi: 10.1016/0014-5793(80)80446-7. [DOI] [PubMed] [Google Scholar]
- Dimroth P. Biotin-dependent decarboxylases as energy transducing systems. Ann N Y Acad Sci. 1985;447:72–85. doi: 10.1111/j.1749-6632.1985.tb18426.x. [DOI] [PubMed] [Google Scholar]
- Dimroth P. Characterization of a membrane-bound biotin-containing enzyme: oxaloacetate decarboxylase from Klebsiella aerogenes. Eur J Biochem. 1981 Apr;115(2):353–358. doi: 10.1111/j.1432-1033.1981.tb05245.x. [DOI] [PubMed] [Google Scholar]
- Dimroth P. Decarboxylation and transport. Biosci Rep. 1982 Nov;2(11):849–860. doi: 10.1007/BF01114890. [DOI] [PubMed] [Google Scholar]
- Dimroth P. Preparation, characterization, and reconstitution of oxaloacetate decarboxylase from Klebsiella aerogenes, a sodium pump. Methods Enzymol. 1986;125:530–540. doi: 10.1016/s0076-6879(86)25042-9. [DOI] [PubMed] [Google Scholar]
- Dimroth P. Reconstitution of sodium transport from purified oxaloacetate decarboxylase and phospholipid vesicles. J Biol Chem. 1981 Dec 10;256(23):11974–11976. [PubMed] [Google Scholar]
- Dimroth P. The generation of an electrochemical gradient of sodium ions upon decarboxylation of oxaloacetate by the membrane-bound and Na+-activated oxaloacetate decarboxylase from Klebsiella aerogenes. Eur J Biochem. 1982 Jan;121(2):443–449. doi: 10.1111/j.1432-1033.1982.tb05807.x. [DOI] [PubMed] [Google Scholar]
- Dimroth P. The role of biotin and sodium in the decarboxylation of oxaloacetate by the membrane-bound oxaloacetate decarboxylase from Klebsiella aerogenes. Eur J Biochem. 1982 Jan;121(2):435–441. doi: 10.1111/j.1432-1033.1982.tb05806.x. [DOI] [PubMed] [Google Scholar]
- Dimroth P., Thomer A. Citrate transport in Klebsiella pneumoniae. Biol Chem Hoppe Seyler. 1986 Aug;367(8):813–823. doi: 10.1515/bchm3.1986.367.2.813. [DOI] [PubMed] [Google Scholar]
- Dimroth P., Thomer A. Kinetic analysis of the reaction mechanism of oxaloacetate decarboxylase from Klebsiella aerogenes. Eur J Biochem. 1986 Apr 1;156(1):157–162. doi: 10.1111/j.1432-1033.1986.tb09561.x. [DOI] [PubMed] [Google Scholar]
- Dimroth P., Thomer A. Subunit composition of oxaloacetate decarboxylase and characterization of the alpha chain as carboxyltransferase. Eur J Biochem. 1983 Dec 1;137(1-2):107–112. doi: 10.1111/j.1432-1033.1983.tb07802.x. [DOI] [PubMed] [Google Scholar]
- Drachev A. L., Markin V. S., Skulachev V. P. delta-mu H-buffering by Na+ and K+ gradients in bacteria. Model and experimental systems. Biochim Biophys Acta. 1985 Jun 3;811(2):197–215. doi: 10.1016/0304-4173(85)90018-7. [DOI] [PubMed] [Google Scholar]
- Dundas I. E. Physiology of halobacteriaceae. Adv Microb Physiol. 1977;15:85–120. doi: 10.1016/s0065-2911(08)60315-x. [DOI] [PubMed] [Google Scholar]
- Eagon R. G., Wilkerson L. S. A potassium-dependent citric acid transport system in Aerobacter aerogenes. Biochem Biophys Res Commun. 1972 Mar 10;46(5):1944–1950. doi: 10.1016/0006-291x(72)90074-5. [DOI] [PubMed] [Google Scholar]
- Epstein W., Whitelaw V., Hesse J. A K+ transport ATPase in Escherichia coli. J Biol Chem. 1978 Oct 10;253(19):6666–6668. [PubMed] [Google Scholar]
- Fein J. E., MacLeod R. A. Characterization of neutral amino acid transport in a marine pseudomonad. J Bacteriol. 1975 Dec;124(3):1177–1190. doi: 10.1128/jb.124.3.1177-1190.1975. [DOI] [PMC free article] [PubMed] [Google Scholar]
- Foubert E. L., Douglas H. C. Studies on the Anaerobic Micrococci: II. The Fermentation of Lactate by Micrococcus lactilyticus. J Bacteriol. 1948 Jul;56(1):35–36. [PMC free article] [PubMed] [Google Scholar]
- Frank L., Hopkins I. Sodium-stimulated transport of glutamate in Escherichia coli. J Bacteriol. 1969 Oct;100(1):329–336. doi: 10.1128/jb.100.1.329-336.1969. [DOI] [PMC free article] [PubMed] [Google Scholar]
- Fujimura T., Yamato I., Anraku Y. Mechanism of glutamate transport in Escherichia coli B. 1. Proton-dependent and sodium ion dependent binding of glutamate to a glutamate carrier in the cytoplasmic membrane. Biochemistry. 1983 Apr 12;22(8):1954–1959. doi: 10.1021/bi00277a033. [DOI] [PubMed] [Google Scholar]
- Fujimura T., Yamato I., Anraku Y. Mechanism of glutamate transport in Escherichia coli B. 2. Kinetics of glutamate transport driven by artificially imposed proton and sodium ion gradients across the cytoplasmic membrane. Biochemistry. 1983 Apr 12;22(8):1959–1965. doi: 10.1021/bi00277a034. [DOI] [PubMed] [Google Scholar]
- Futai M. Orientation of membrane vesicles from Escherichia coli prepared by different procedures. J Membr Biol. 1974;15(1):15–28. doi: 10.1007/BF01870079. [DOI] [PubMed] [Google Scholar]
- Fürst P., Solioz M. The vanadate-sensitive ATPase of Streptococcus faecalis pumps potassium in a reconstituted system. J Biol Chem. 1986 Mar 25;261(9):4302–4308. [PubMed] [Google Scholar]
- Galivan J. H., Allen S. H. Methylmalonyl coenzyme A decarboxylase. Its role in succinate decarboxylation by Micrococcus lactilyticus. J Biol Chem. 1968 Mar 25;243(6):1253–1261. [PubMed] [Google Scholar]
- Galivan J. H., Allen S. H. Methylmalonyl-CoA decarboxylase: partial purification and enzymatic properties. Arch Biochem Biophys. 1968 Sep 10;126(3):838–847. doi: 10.1016/0003-9861(68)90477-3. [DOI] [PubMed] [Google Scholar]
- Garcia M. L., Guffanti A. A., Krulwich T. A. Characterization of the Na+/H+ antiporter of alkalophilic bacilli in vivo: delta psi-dependent 22Na+ efflux from whole cells. J Bacteriol. 1983 Dec;156(3):1151–1157. doi: 10.1128/jb.156.3.1151-1157.1983. [DOI] [PMC free article] [PubMed] [Google Scholar]
- Guffanti A. A., Cohn D. E., Kaback H. R., Krulwich T. A. Relationship between the Na+/H+ antiporter and Na+/substrate symport in Bacillus alcalophilus. Proc Natl Acad Sci U S A. 1981 Mar;78(3):1481–1484. doi: 10.1073/pnas.78.3.1481. [DOI] [PMC free article] [PubMed] [Google Scholar]
- Hall B. G. Chromosomal mutation for citrate utilization by Escherichia coli K-12. J Bacteriol. 1982 Jul;151(1):269–273. doi: 10.1128/jb.151.1.269-273.1982. [DOI] [PMC free article] [PubMed] [Google Scholar]
- Hamaide F., Sprott G. D., Kushner D. J. Energetics of sodium-dependent alpha-aminoisobutyric acid transport in the moderate halophile Vibrio costicola. Biochim Biophys Acta. 1984 Jul 27;766(1):77–87. doi: 10.1016/0005-2728(84)90219-6. [DOI] [PubMed] [Google Scholar]
- Hanatani M., Yazyu H., Shiota-Niiya S., Moriyama Y., Kanazawa H., Futai M., Tsuchiya T. Physical and genetic characterization of the melibiose operon and identification of the gene products in Escherichia coli. J Biol Chem. 1984 Feb 10;259(3):1807–1812. [PubMed] [Google Scholar]
- Hasan S. M., Tsuchiya T. Glutamate transport driven by an electrochemical gradient of sodium ion in membrane vesicles of Escherichia coli B. Biochem Biophys Res Commun. 1977 Sep 9;78(1):122–128. doi: 10.1016/0006-291x(77)91229-3. [DOI] [PubMed] [Google Scholar]
- Hatefi Y. The mitochondrial electron transport and oxidative phosphorylation system. Annu Rev Biochem. 1985;54:1015–1069. doi: 10.1146/annurev.bi.54.070185.005055. [DOI] [PubMed] [Google Scholar]
- Heefner D. L., Harold F. M. ATP-driven sodium pump in Streptococcus faecalis. Proc Natl Acad Sci U S A. 1982 May;79(9):2798–2802. doi: 10.1073/pnas.79.9.2798. [DOI] [PMC free article] [PubMed] [Google Scholar]
- Heefner D. L., Harold F. M. ATP-linked sodium transport in Streptococcus faecalis. I. The sodium circulation. J Biol Chem. 1980 Dec 10;255(23):11396–11402. [PubMed] [Google Scholar]
- Heefner D. L., Kobayashi H., Harold F. M. ATP-linked sodium transport in Streptococcus faecalis. II. Energy coupling in everted membrane vesicles. J Biol Chem. 1980 Dec 10;255(23):11403–11407. [PubMed] [Google Scholar]
- Hilpert W., Dimroth P. Conversion of the chemical energy of methylmalonyl-CoA decarboxylation into a Na+ gradient. Nature. 1982 Apr 8;296(5857):584–585. doi: 10.1038/296584a0. [DOI] [PubMed] [Google Scholar]
- Hilpert W., Dimroth P. Purification and characterization of a new sodium-transport decarboxylase. Methylmalonyl-CoA decarboxylase from Veillonella alcalescens. Eur J Biochem. 1983 May 16;132(3):579–587. doi: 10.1111/j.1432-1033.1983.tb07403.x. [DOI] [PubMed] [Google Scholar]
- Hilpert W., Dimroth P. Reconstitution of Na+ transport from purified methylmalonyl-CoA decarboxylase and phospholipid vesicles. Eur J Biochem. 1984 Feb 1;138(3):579–583. doi: 10.1111/j.1432-1033.1984.tb07953.x. [DOI] [PubMed] [Google Scholar]
- Hilpert W., Schink B., Dimroth P. Life by a new decarboxylation-dependent energy conservation mechanism with Na as coupling ion. EMBO J. 1984 Aug;3(8):1665–1670. doi: 10.1002/j.1460-2075.1984.tb02030.x. [DOI] [PMC free article] [PubMed] [Google Scholar]
- Hirata H., Kambe T., Kagawa Y. A purified alanine carrier composed of a single polypeptide from thermophilic bacterium PS3 driven by either proton or sodium ion gradient. J Biol Chem. 1984 Sep 10;259(17):10653–10656. [PubMed] [Google Scholar]
- Hirato T., Ishiguro N., Shinagawa M., Sato G. Conservation of DNA sequences for plasmid-mediated citrate utilization within the enterobacteria. J Bacteriol. 1986 Jan;165(1):324–327. doi: 10.1128/jb.165.1.324-327.1986. [DOI] [PMC free article] [PubMed] [Google Scholar]
- Hirota N., Imae Y. Na+-driven flagellar motors of an alkalophilic Bacillus strain YN-1. J Biol Chem. 1983 Sep 10;258(17):10577–10581. [PubMed] [Google Scholar]
- Hoshino T., Kageyama M. Sodium-dependent transport of L-leucine in membrane vesicles prepared from Pseudomonas aeruginosa. J Bacteriol. 1979 Jan;137(1):73–81. doi: 10.1128/jb.137.1.73-81.1979. [DOI] [PMC free article] [PubMed] [Google Scholar]
- Hugentobler G., Heid I., Solioz M. Purification of a putative K+-ATPase from Streptococcus faecalis. J Biol Chem. 1983 Jun 25;258(12):7611–7617. [PubMed] [Google Scholar]
- Ishiguro N., Oka C., Sato G. Isolation of citrate-positive variants of Escherichia coli from domestic pigeons, pigs, cattle, and horses. Appl Environ Microbiol. 1978 Aug;36(2):217–222. doi: 10.1128/aem.36.2.217-222.1978. [DOI] [PMC free article] [PubMed] [Google Scholar]
- Ishiguro N., Sato G. Nucleotide sequence of the gene determining plasmid-mediated citrate utilization. J Bacteriol. 1985 Dec;164(3):977–982. doi: 10.1128/jb.164.3.977-982.1985. [DOI] [PMC free article] [PubMed] [Google Scholar]
- Ishiguro N., Sato G. The distribution of plasmids determining citrate utilization in citrate-positive variants of Escherichia coli from humans, domestic animals, feral birds and environments. J Hyg (Lond) 1979 Oct;83(2):331–344. doi: 10.1017/s0022172400026127. [DOI] [PMC free article] [PubMed] [Google Scholar]
- JOHNS A. T. The mechanism of propionic acid formation by Veillonella gazogenes. J Gen Microbiol. 1951 May;5(2):326–336. doi: 10.1099/00221287-5-2-326. [DOI] [PubMed] [Google Scholar]
- Jencks W. P. The utilization of binding energy in coupled vectorial processes. Adv Enzymol Relat Areas Mol Biol. 1980;51:75–106. doi: 10.1002/9780470122969.ch2. [DOI] [PubMed] [Google Scholar]
- Jinks D. C., Silvius J. R., McElhaney R. N. Physiological role and membrane lipid modulation of the membrane-bound (Mg2+, na+)-adenosine triphosphatase activity in Acholeplasma laidlawii. J Bacteriol. 1978 Dec;136(3):1027–1036. doi: 10.1128/jb.136.3.1027-1036.1978. [DOI] [PMC free article] [PubMed] [Google Scholar]
- Johnson C. L., Cha Y. A., Stern J. R. Citrate uptake in membrane vesicles of Klebsiella aerogenes. J Bacteriol. 1975 Feb;121(2):682–687. doi: 10.1128/jb.121.2.682-687.1975. [DOI] [PMC free article] [PubMed] [Google Scholar]
- Kakinuma Y., Harold F. M. ATP-driven exchange of Na+ and K+ ions by Streptococcus faecalis. J Biol Chem. 1985 Feb 25;260(4):2086–2091. [PubMed] [Google Scholar]
- Kakinuma Y., Unemoto T. Sucrose uptake is driven by the Na+ electrochemical potential in the marine bacterium Vibrio alginolyticus. J Bacteriol. 1985 Sep;163(3):1293–1295. doi: 10.1128/jb.163.3.1293-1295.1985. [DOI] [PMC free article] [PubMed] [Google Scholar]
- Kay W. W., Cameron M. Citrate transport in Salmonella typhimurium. Arch Biochem Biophys. 1978 Sep;190(1):270–280. doi: 10.1016/0003-9861(78)90276-x. [DOI] [PubMed] [Google Scholar]
- Ken-Dror S., Lanyi J. K., Schobert B., Silver B., Avi-Dor Y. An NADH:quinone oxidoreductase of the halotolerant bacterium Ba1 is specifically dependent on sodium ions. Arch Biochem Biophys. 1986 Feb 1;244(2):766–772. doi: 10.1016/0003-9861(86)90645-4. [DOI] [PubMed] [Google Scholar]
- Ken-Dror S., Preger R., Avi-Dor Y. Functional characterization of the uncoupler-insensitive Na+ pump of the halotolerant bacterium, Ba1. Arch Biochem Biophys. 1986 Jan;244(1):122–127. doi: 10.1016/0003-9861(86)90100-1. [DOI] [PubMed] [Google Scholar]
- Ken-Dror S., Shnaiderman R., Avi-Dor Y. Uncoupler-stimulated Na+ pump and its possible role in the halotolerant bacterium, Ba. Arch Biochem Biophys. 1984 Mar;229(2):640–649. doi: 10.1016/0003-9861(84)90197-8. [DOI] [PubMed] [Google Scholar]
- Khan S., Macnab R. M. Proton chemical potential, proton electrical potential and bacterial motility. J Mol Biol. 1980 Apr 15;138(3):599–614. doi: 10.1016/s0022-2836(80)80019-2. [DOI] [PubMed] [Google Scholar]
- Kinoshita N., Unemoto T., Kobayashi H. Sodium-stimulated ATPase in Streptococcus faecalis. J Bacteriol. 1984 Jun;158(3):844–848. doi: 10.1128/jb.158.3.844-848.1984. [DOI] [PMC free article] [PubMed] [Google Scholar]
- Kobayashi H. Second system for potassium transport in Streptococcus faecalis. J Bacteriol. 1982 May;150(2):506–511. doi: 10.1128/jb.150.2.506-511.1982. [DOI] [PMC free article] [PubMed] [Google Scholar]
- Koser S. A. CORRELATION OF CITRATE UTILIZATION BY MEMBERS OF THE COLON-AEROGENES GROUP WITH OTHER DIFFERENTIAL CHARACTERISTICS AND WITH HABITAT. J Bacteriol. 1924 Jan;9(1):59–77. doi: 10.1128/jb.9.1.59-77.1924. [DOI] [PMC free article] [PubMed] [Google Scholar]
- Krulwich T. A., Federbush J. G., Guffanti A. A. Presence of a nonmetabolizable solute that is translocated with Na+ enhances Na+-dependent pH homeostasis in an alkalophilic Bacillus. J Biol Chem. 1985 Apr 10;260(7):4055–4058. [PubMed] [Google Scholar]
- Krulwich T. A., Guffanti A. A., Bornstein R. F., Hoffstein J. A sodium requirement for growth, solute transport, and pH homeostasis in Bacillus firmus RAB. J Biol Chem. 1982 Feb 25;257(4):1885–1889. [PubMed] [Google Scholar]
- Krulwich T. A., Guffanti A. A., Fong M. Y., Falk L., Hicks D. B. Alkalophilic Bacillus firmus RAB generates variants which can grow at lower Na+ concentrations than the parental strain. J Bacteriol. 1986 Mar;165(3):884–889. doi: 10.1128/jb.165.3.884-889.1986. [DOI] [PMC free article] [PubMed] [Google Scholar]
- Krulwich T. A., Guffanti A. A. Physiology of acidophilic and alkalophilic bacteria. Adv Microb Physiol. 1983;24:173–214. doi: 10.1016/s0065-2911(08)60386-0. [DOI] [PubMed] [Google Scholar]
- Krulwich T. A., Mandel K. G., Bornstein R. F., Guffanti A. A. A non-alkalophilic mutant of Bacillus alcalophilus lacks the Na+/H+ antiporter. Biochem Biophys Res Commun. 1979 Nov 14;91(1):58–62. doi: 10.1016/0006-291x(79)90582-5. [DOI] [PubMed] [Google Scholar]
- Krulwich T. A. Na+/H+ antiporters. Biochim Biophys Acta. 1983 Dec 30;726(4):245–264. doi: 10.1016/0304-4173(83)90011-3. [DOI] [PubMed] [Google Scholar]
- Kröger A. Electron-transport phosphorylation coupled to fumarate reduction in anaerobically grown Proteus rettgeri. Biochim Biophys Acta. 1974 May 22;347(2):273–289. doi: 10.1016/0005-2728(74)90051-6. [DOI] [PubMed] [Google Scholar]
- Kusaka I., Kanai K. Characterization of glutamate transport system in hydrophobic protein (H protein) of Bacillus subtilis. Biochim Biophys Acta. 1979 Apr 19;552(3):492–498. doi: 10.1016/0005-2736(79)90193-7. [DOI] [PubMed] [Google Scholar]
- LARA F. J. S., STOKES J. L. Oxidation of citrate by Escherichia coli. J Bacteriol. 1952 Mar;63(3):415–420. doi: 10.1128/jb.63.3.415-420.1952. [DOI] [PMC free article] [PubMed] [Google Scholar]
- Lanyi J. K., MacDonald R. E. Existence of electrogenic hydrogen ion/sodium ion antiport in Halobacterium halobium cell envelope vesicles. Biochemistry. 1976 Oct 19;15(21):4608–4614. doi: 10.1021/bi00666a010. [DOI] [PubMed] [Google Scholar]
- Lanyi J. K., Renthal R., MacDonald R. E. Light-induced glutamate transport in Halobacterium halobium envelope vesicles. II. Evidence that the driving force is a light-dependent sodium gradient. Biochemistry. 1976 Apr 20;15(8):1603–1610. doi: 10.1021/bi00653a002. [DOI] [PubMed] [Google Scholar]
- Lanyi J. K., Silverman M. P. Gating effects in Halobacterium halobium membrane transport. J Biol Chem. 1979 Jun 10;254(11):4750–4755. [PubMed] [Google Scholar]
- Lanyi J. K. The role of Na+ in transport processes of bacterial membranes. Biochim Biophys Acta. 1979 Dec 20;559(4):377–397. doi: 10.1016/0304-4157(79)90011-x. [DOI] [PubMed] [Google Scholar]
- Lewis R. N., McElhaney R. N. Purification and characterization of the membrane (Na+ + Mg2+)-ATPase from Acholeplasma laidlawii B. Biochim Biophys Acta. 1983 Oct 26;735(1):113–122. doi: 10.1016/0005-2736(83)90266-3. [DOI] [PubMed] [Google Scholar]
- Linker C., Wilson T. H. Characterization and solubilization of the membrane-bound ATPase of Mycoplasma gallisepticum. J Bacteriol. 1985 Sep;163(3):1258–1262. doi: 10.1128/jb.163.3.1258-1262.1985. [DOI] [PMC free article] [PubMed] [Google Scholar]
- Linker C., Wilson T. H. Sodium and proton transport in Mycoplasma gallisepticum. J Bacteriol. 1985 Sep;163(3):1250–1257. doi: 10.1128/jb.163.3.1250-1257.1985. [DOI] [PMC free article] [PubMed] [Google Scholar]
- Lütgens M., Gottschalk G. Why a co-substrate is required for anaerobic growth of Escherichia coli on citrate. J Gen Microbiol. 1980 Jul;119(1):63–70. doi: 10.1099/00221287-119-1-63. [DOI] [PubMed] [Google Scholar]
- MITCHELL P. Coupling of phosphorylation to electron and hydrogen transfer by a chemi-osmotic type of mechanism. Nature. 1961 Jul 8;191:144–148. doi: 10.1038/191144a0. [DOI] [PubMed] [Google Scholar]
- MacDonald R. E., Greene R. V., Lanyi J. K. Light-activated amino acid transport systems in Halobacterium halobium envelope vesicles: role of chemical and electrical gradients. Biochemistry. 1977 Jul 12;16(14):3227–3235. doi: 10.1021/bi00633a029. [DOI] [PubMed] [Google Scholar]
- MacDonald R. E., Lanyi J. K., Greene R. V. Sodium-stimulated glutamate uptake in membrane vesicles of Escherichia coli: the role of ion gradients. Proc Natl Acad Sci U S A. 1977 Aug;74(8):3167–3170. doi: 10.1073/pnas.74.8.3167. [DOI] [PMC free article] [PubMed] [Google Scholar]
- MacLeod P. R., MacLeod R. A. Cloning in Escherichia coli K-12 of a Na+-dependent transport system from a marine bacterium. J Bacteriol. 1986 Mar;165(3):825–830. doi: 10.1128/jb.165.3.825-830.1986. [DOI] [PMC free article] [PubMed] [Google Scholar]
- Macnab R. M., Aizawa S. Bacterial motility and the bacterial flagellar motor. Annu Rev Biophys Bioeng. 1984;13:51–83. doi: 10.1146/annurev.bb.13.060184.000411. [DOI] [PubMed] [Google Scholar]
- Mah R. A., Smith M. R., Baresi L. Studies on an acetate-fermenting strain of Methanosarcina. Appl Environ Microbiol. 1978 Jun;35(6):1174–1184. doi: 10.1128/aem.35.6.1174-1184.1978. [DOI] [PMC free article] [PubMed] [Google Scholar]
- Mandel K. G., Guffanti A. A., Krulwich T. A. Monovalent cation/proton antiporters in membrane vesicles from Bacillus alcalophilus. J Biol Chem. 1980 Aug 10;255(15):7391–7396. [PubMed] [Google Scholar]
- Manson M. D., Tedesco P. M., Berg H. C. Energetics of flagellar rotation in bacteria. J Mol Biol. 1980 Apr 15;138(3):541–561. doi: 10.1016/s0022-2836(80)80017-9. [DOI] [PubMed] [Google Scholar]
- Miller A. G., Turpin D. H., Canvin D. T. Na+ requirement for growth, photosynthesis, and pH regulation in the alkalotolerant cyanobacterium Synechococcus leopoliensis. J Bacteriol. 1984 Jul;159(1):100–106. doi: 10.1128/jb.159.1.100-106.1984. [DOI] [PMC free article] [PubMed] [Google Scholar]
- Mitchell P. A chemiosmotic molecular mechanism for proton-translocating adenosine triphosphatases. FEBS Lett. 1974 Jul 15;43(2):189–194. doi: 10.1016/0014-5793(74)80997-x. [DOI] [PubMed] [Google Scholar]
- Mitchell P. A commentary on alternative hypotheses of protonic coupling in the membrane systems catalysing oxidative and photosynthetic phosphorylation. FEBS Lett. 1977;78(1):1–20. doi: 10.1016/0014-5793(77)80263-9. [DOI] [PubMed] [Google Scholar]
- Mitchell P. Performance and conservation of osmotic work by proton-coupled solute porter systems. J Bioenerg. 1973 Jan;4(1):63–91. doi: 10.1007/BF01516051. [DOI] [PubMed] [Google Scholar]
- Mitchell P. Vectorial chemistry and the molecular mechanics of chemiosmotic coupling: power transmission by proticity. Biochem Soc Trans. 1976;4(3):399–430. doi: 10.1042/bst0040399. [DOI] [PubMed] [Google Scholar]
- Moss J., Lane M. D. The biotin-dependent enzymes. Adv Enzymol Relat Areas Mol Biol. 1971;35:321–442. doi: 10.1002/9780470122808.ch7. [DOI] [PubMed] [Google Scholar]
- Nakamura T., Tokuda H., Unemoto T. K+/H+ antiporter functions as a regulator of cytoplasmic pH in a marine bacterium, Vibrio alginolyticus. Biochim Biophys Acta. 1984 Oct 3;776(2):330–336. doi: 10.1016/0005-2736(84)90222-0. [DOI] [PubMed] [Google Scholar]
- Ng S. K., Hamilton I. R. Lactate metabolism by Veillonella parvula. J Bacteriol. 1971 Mar;105(3):999–1005. doi: 10.1128/jb.105.3.999-1005.1971. [DOI] [PMC free article] [PubMed] [Google Scholar]
- Niiya S., Yamasaki K., Wilson T. H., Tsuchiya T. Altered cation coupling to melibiose transport in mutants of Escherichia coli. J Biol Chem. 1982 Aug 10;257(15):8902–8906. [PubMed] [Google Scholar]
- Niven D. F., MacLeod R. A. Sodium ion-proton antiport in a marine bacterium. J Bacteriol. 1978 Jun;134(3):737–743. doi: 10.1128/jb.134.3.737-743.1978. [DOI] [PMC free article] [PubMed] [Google Scholar]
- Niven D. F., MacLeod R. A. Sodium ion-substrate symport in a marine bacterium. J Bacteriol. 1980 May;142(2):603–607. doi: 10.1128/jb.142.2.603-607.1980. [DOI] [PMC free article] [PubMed] [Google Scholar]
- O'Brien Induction by sodium of the citrate fermentation enzymes in Klebsiella aerogenes. FEBS Lett. 1975 Apr 15;53(1):61–63. doi: 10.1016/0014-5793(75)80682-x. [DOI] [PubMed] [Google Scholar]
- O'Brien R. W., Frost G. M., Stern J. R. Enzymatic analysis of the requirement for sodium in aerobic growth of Salmonella typhimurium on citrate. J Bacteriol. 1969 Aug;99(2):395–400. doi: 10.1128/jb.99.2.395-400.1969. [DOI] [PMC free article] [PubMed] [Google Scholar]
- O'Brien R. W., Stern J. R. Requirement for sodium in the anaerobic growth of Aerobacter aerogenes on citrate. J Bacteriol. 1969 May;98(2):388–393. doi: 10.1128/jb.98.2.388-393.1969. [DOI] [PMC free article] [PubMed] [Google Scholar]
- O'Brien R. W., Stern J. R. Role of sodium in determining alternate pathways of aerobic citrate catabolism in Aerobacter aerogenes. J Bacteriol. 1969 Aug;99(2):389–394. doi: 10.1128/jb.99.2.389-394.1969. [DOI] [PMC free article] [PubMed] [Google Scholar]
- Otto R., Sonnenberg A. S., Veldkamp H., Konings W. N. Generation of an electrochemical proton gradient in Streptococcus cremoris by lactate efflux. Proc Natl Acad Sci U S A. 1980 Sep;77(9):5502–5506. doi: 10.1073/pnas.77.9.5502. [DOI] [PMC free article] [PubMed] [Google Scholar]
- Padan E., Zilberstein D., Schuldiner S. pH homeostasis in bacteria. Biochim Biophys Acta. 1981 Dec;650(2-3):151–166. doi: 10.1016/0304-4157(81)90004-6. [DOI] [PubMed] [Google Scholar]
- ROGOSA M. THE GENUS VEILLONELLA. I. GENERAL CULTURAL, ECOLOGICAL, AND BIOCHEMICAL CONSIDERATIONS. J Bacteriol. 1964 Jan;87:162–170. doi: 10.1128/jb.87.1.162-170.1964. [DOI] [PMC free article] [PubMed] [Google Scholar]
- Racker E., Violand B., O'Neal S., Alfonzo M., Telford J. Reconstitution, a way of biochemical research; some new approaches to membrane-bound enzymes. Arch Biochem Biophys. 1979 Dec;198(2):470–477. doi: 10.1016/0003-9861(79)90521-6. [DOI] [PubMed] [Google Scholar]
- Reenstra W. W., Patel L., Rottenberg H., Kaback H. R. Electrochemical proton gradient in inverted membrane vesicles from Escherichia coli. Biochemistry. 1980 Jan 8;19(1):1–9. doi: 10.1021/bi00542a001. [DOI] [PubMed] [Google Scholar]
- Reichelt J. L., Baumann P. Effect of sodium chloride on growth of heterotrophic marine bacteria. Arch Microbiol. 1974 May 20;97(4):329–345. doi: 10.1007/BF00403071. [DOI] [PubMed] [Google Scholar]
- Reynolds C. H., Silver S. Citrate utilization by Escherichia coli: plasmid- and chromosome-encoded systems. J Bacteriol. 1983 Dec;156(3):1019–1024. doi: 10.1128/jb.156.3.1019-1024.1983. [DOI] [PMC free article] [PubMed] [Google Scholar]
- Rhoads D. B., Epstein W. Energy coupling to net K+ transport in Escherichia coli K-12. J Biol Chem. 1977 Feb 25;252(4):1394–1401. [PubMed] [Google Scholar]
- Sasatsu M., Misra T. K., Chu L., Laddaga R., Silver S. Cloning and DNA sequence of a plasmid-determined citrate utilization system in Escherichia coli. J Bacteriol. 1985 Dec;164(3):983–993. doi: 10.1128/jb.164.3.983-993.1985. [DOI] [PMC free article] [PubMed] [Google Scholar]
- Schobert B., Lanyi J. K. Halorhodopsin is a light-driven chloride pump. J Biol Chem. 1982 Sep 10;257(17):10306–10313. [PubMed] [Google Scholar]
- Schuldiner S., Fishkes H. Sodium-proton antiport in isolated membrane vesicles of Escherichia coli. Biochemistry. 1978 Feb 21;17(4):706–711. doi: 10.1021/bi00597a023. [DOI] [PubMed] [Google Scholar]
- Schwarz E., Oesterhelt D. Cloning and expression of Klebsiella pneumoniae genes coding for citrate transport and fermentation. EMBO J. 1985 Jun;4(6):1599–1603. doi: 10.1002/j.1460-2075.1985.tb03823.x. [DOI] [PMC free article] [PubMed] [Google Scholar]
- Schweiger G., Buckel W. On the dehydration of (R)-lactate in the fermentation of alanine to propionate by Clostridium propionicum. FEBS Lett. 1984 Jun 4;171(1):79–84. doi: 10.1016/0014-5793(84)80463-9. [DOI] [PubMed] [Google Scholar]
- Skulachev V. P. Membrane-linked energy buffering as the biological function of Na+/K+ gradient. FEBS Lett. 1978 Mar 15;87(2):171–179. doi: 10.1016/0014-5793(78)80326-3. [DOI] [PubMed] [Google Scholar]
- Skulachev V. P. Membrane-linked energy transductions. Bioenergetic functions of sodium: H+ is not unique as a coupling ion. Eur J Biochem. 1985 Sep 2;151(2):199–208. doi: 10.1111/j.1432-1033.1985.tb09088.x. [DOI] [PubMed] [Google Scholar]
- Smith H. W., Parsell Z., Green P. Thermosensitive H1 plasmids determining citrate utilization. J Gen Microbiol. 1978 Dec;109(2):305–311. doi: 10.1099/00221287-109-2-305. [DOI] [PubMed] [Google Scholar]
- Sprott G. D., Drozdowski J. P., Martin E. L., MacLeod R. A. Kinetics of Naplus-dependent amino acid transport using cells and membrane vesicles of a marine pseudomonad. Can J Microbiol. 1975 Jan;21(1):43–50. doi: 10.1139/m75-006. [DOI] [PubMed] [Google Scholar]
- Sprott G. D., MacLeod R. A. Na + -dependent amino acid transport in isolated membrane vesicles of a marine pseudomonad energized by electron donors. Biochem Biophys Res Commun. 1972 May 26;47(4):838–845. doi: 10.1016/0006-291x(72)90569-4. [DOI] [PubMed] [Google Scholar]
- Stern J. R. Oxalacetate decarboxylase of Aerobacter aerogenes. I. Inhibition by avidin and requirement for sodium ion. Biochemistry. 1967 Nov;6(11):3545–3551. doi: 10.1021/bi00863a028. [DOI] [PubMed] [Google Scholar]
- Stock J., Roseman S. A sodium-dependent sugar co-transport system in bacteria. Biochem Biophys Res Commun. 1971 Jul 2;44(1):132–138. doi: 10.1016/s0006-291x(71)80168-7. [DOI] [PubMed] [Google Scholar]
- Stoeckenius W., Lozier R. H., Bogomolni R. A. Bacteriorhodopsin and the purple membrane of halobacteria. Biochim Biophys Acta. 1979 Mar 14;505(3-4):215–278. doi: 10.1016/0304-4173(79)90006-5. [DOI] [PubMed] [Google Scholar]
- Sugiyama S., Matsukura H., Imae Y. Relationship between Na+-dependent cytoplasmic pH homeostasis and Na+-dependent flagellar rotation and amino acid transport in alkalophilic Bacillus. FEBS Lett. 1985 Mar 25;182(2):265–268. doi: 10.1016/0014-5793(85)80312-4. [DOI] [PubMed] [Google Scholar]
- Sweet G. D., Kay C. M., Kay W. W. Tricarboxylate-binding proteins of Salmonella typhimurium. Purification, crystallization, and physical properties. J Biol Chem. 1984 Feb 10;259(3):1586–1592. [PubMed] [Google Scholar]
- Sweet G. D., Somers J. M., Kay W. W. Purification and properties of a citrate-binding transport component, the C protein of Salmonella typhimurium. Can J Biochem. 1979 Jun;57(6):710–715. doi: 10.1139/o79-089. [DOI] [PubMed] [Google Scholar]
- Tanford C. Mechanism of free energy coupling in active transport. Annu Rev Biochem. 1983;52:379–409. doi: 10.1146/annurev.bi.52.070183.002115. [DOI] [PubMed] [Google Scholar]
- Thauer R. K., Jungermann K., Decker K. Energy conservation in chemotrophic anaerobic bacteria. Bacteriol Rev. 1977 Mar;41(1):100–180. doi: 10.1128/br.41.1.100-180.1977. [DOI] [PMC free article] [PubMed] [Google Scholar]
- Thompson J., MacLeod R. A. Na+ and K+ gradients and alpha-aminoisobutyric acid transport in a marine pseudomonad. J Biol Chem. 1973 Oct 25;248(20):7106–7111. [PubMed] [Google Scholar]
- Tokuda H. Isolation of Vibrio alginolyticus mutants defective in the respiration-coupled Na+ pump. Biochem Biophys Res Commun. 1983 Jul 18;114(1):113–118. doi: 10.1016/0006-291x(83)91601-7. [DOI] [PubMed] [Google Scholar]
- Tokuda H., Kaback H. R. Sodium-dependent methyl 1-thio-beta-D-galactopyranoside transport in membrane vesicles isolated from Salmonella typhimurium. Biochemistry. 1977 May 17;16(10):2130–2136. doi: 10.1021/bi00629a013. [DOI] [PubMed] [Google Scholar]
- Tokuda H. Solubilization and reconstitution of the Na+-motive NADH oxidase activity from the marine bacterium Vibrio alginolyticus. FEBS Lett. 1984 Oct 15;176(1):125–128. doi: 10.1016/0014-5793(84)80925-4. [DOI] [PubMed] [Google Scholar]
- Tokuda H., Sugasawa M., Unemoto T. Roles of Na+ and K+ in alpha-aminoisobutyric acid transport by the marine bacterium Vibrio alginolyticus. J Biol Chem. 1982 Jan 25;257(2):788–794. [PubMed] [Google Scholar]
- Tokuda H., Udagawa T., Unemoto T. Generation of the electrochemical potential of Na+ by the Na+-motive NADH oxidase in inverted membrane vesicles of Vibrio alginolyticus. FEBS Lett. 1985 Apr 8;183(1):95–98. doi: 10.1016/0014-5793(85)80961-3. [DOI] [PubMed] [Google Scholar]
- Tokuda H., Unemoto T. A respiration-dependent primary sodium extrusion system functioning at alkaline pH in the marine bacterium Vibrio alginolyticus. Biochem Biophys Res Commun. 1981 Sep 16;102(1):265–271. doi: 10.1016/0006-291x(81)91516-3. [DOI] [PubMed] [Google Scholar]
- Tokuda H., Unemoto T. Characterization of the respiration-dependent Na+ pump in the marine bacterium Vibrio alginolyticus. J Biol Chem. 1982 Sep 10;257(17):10007–10014. [PubMed] [Google Scholar]
- Tokuda H., Unemoto T. Growth of a marine Vibrio alginolyticus and moderately halophilic V. costicola becomes uncoupler resistant when the respiration-dependent Na+ pump functions. J Bacteriol. 1983 Nov;156(2):636–643. doi: 10.1128/jb.156.2.636-643.1983. [DOI] [PMC free article] [PubMed] [Google Scholar]
- Tokuda H., Unemoto T. Na+ is translocated at NADH:quinone oxidoreductase segment in the respiratory chain of Vibrio alginolyticus. J Biol Chem. 1984 Jun 25;259(12):7785–7790. [PubMed] [Google Scholar]
- Tsuchiya T., Raven J., Wilson T. H. Co-transport of Na+ and methul-beta-D-thiogalactopyranoside mediated by the melibiose transport system of Escherichia coli. Biochem Biophys Res Commun. 1977 May 9;76(1):26–31. doi: 10.1016/0006-291x(77)91663-1. [DOI] [PubMed] [Google Scholar]
- Tsuchiya T., Shinoda S. Respiration-driven Na+ pump and Na+ circulation in Vibrio parahaemolyticus. J Bacteriol. 1985 May;162(2):794–798. doi: 10.1128/jb.162.2.794-798.1985. [DOI] [PMC free article] [PubMed] [Google Scholar]
- Tsuchiya T., Wilson T. H. Cation-sugar cotransport in the melibiose transport system of Escherichia coli. Membr Biochem. 1978;2(1):63–79. doi: 10.3109/09687687809063858. [DOI] [PubMed] [Google Scholar]
- Udagawa T., Unemoto T., Tokuda H. Generation of Na+ electrochemical potential by the Na+-motive NADH oxidase and Na+/H+ antiport system of a moderately halophilic Vibrio costicola. J Biol Chem. 1986 Feb 25;261(6):2616–2622. [PubMed] [Google Scholar]
- Unemoto T., Hayashi M., Hayashi M. Na+-dependent activation of NADH oxidase in membrane fractions from halophilic Vibrio alginolyticus and V. costicolus. J Biochem. 1977 Nov;82(5):1389–1395. doi: 10.1093/oxfordjournals.jbchem.a131826. [DOI] [PubMed] [Google Scholar]
- Unemoto T., Hayashi M. NADH: quinone oxidoreductase as a site of Na+-dependent activation in the respiratory chain of marine Vibrio alginolyticus. J Biochem. 1979 Jun;85(6):1461–1467. doi: 10.1093/oxfordjournals.jbchem.a132474. [DOI] [PubMed] [Google Scholar]
- WHEAT R. W., AJL S. J. Citritase, the citrate-splitting enzyme from Escherichia coli. II. Reaction mechanisms. J Biol Chem. 1955 Dec;217(2):909–920. [PubMed] [Google Scholar]
- WOOD H. G., STJERNHOLM R. Transcarboxylase. II. Purification and properties of methylmalonyl-oxaloacetic transcarboxylase. Proc Natl Acad Sci U S A. 1961 Mar 15;47:289–303. doi: 10.1073/pnas.47.3.289. [DOI] [PMC free article] [PubMed] [Google Scholar]
- Wagner G., Hartmann R., Oesterhelt D. Potassium uniport and ATP synthesis in Halobacterium halobium. Eur J Biochem. 1978 Aug 15;89(1):169–179. doi: 10.1111/j.1432-1033.1978.tb20909.x. [DOI] [PubMed] [Google Scholar]
- West I. C., Mitchell P. Proton/sodium ion antiport in Escherichia coli. Biochem J. 1974 Oct;144(1):87–90. doi: 10.1042/bj1440087. [DOI] [PMC free article] [PubMed] [Google Scholar]
- Westlake D. W., Horler D. F., McConnell W. B. The effect of sodium on the fermentation of glutamic acid by Peptococcus aerogenes. Biochem Biophys Res Commun. 1967 Feb 21;26(4):461–465. doi: 10.1016/0006-291x(67)90569-4. [DOI] [PubMed] [Google Scholar]
- Wieczorek L., Altendorf K. Potassium transport in Escherichia coli. Evidence for a K+-transport adenosine-5'-triphosphatase. FEBS Lett. 1979 Feb 15;98(2):233–236. doi: 10.1016/0014-5793(79)80189-1. [DOI] [PubMed] [Google Scholar]
- Wilkerson L. S., Eagon R. G. Effect of sodium on the transport and utilization of citric acid by Aerobacter (Enterobacter) aerogenes. J Bacteriol. 1974 Oct;120(1):121–124. doi: 10.1128/jb.120.1.121-124.1974. [DOI] [PMC free article] [PubMed] [Google Scholar]
- Willecke K., Gries E. M., Oehr P. Coupled transport of citrate and magnesium in Bacillus subtilis. J Biol Chem. 1973 Feb 10;248(3):807–814. [PubMed] [Google Scholar]
- Wilson T. H., Maloney P. C. Speculations on the evolution of ion transport mechanisms. Fed Proc. 1976 Aug;35(10):2174–2179. [PubMed] [Google Scholar]
- Wimmer M. J., Rose I. A. Mechanisms of enzyme-catalyzed group transfer reactions. Annu Rev Biochem. 1978;47:1031–1078. doi: 10.1146/annurev.bi.47.070178.005123. [DOI] [PubMed] [Google Scholar]
- Wohlfarth G., Buckel W. A sodium ion gradient as energy source for Peptostreptococcus asaccharolyticus. Arch Microbiol. 1985 Jul;142(2):128–135. doi: 10.1007/BF00447055. [DOI] [PubMed] [Google Scholar]
- Wood H. G., Barden R. E. Biotin enzymes. Annu Rev Biochem. 1977;46:385–413. doi: 10.1146/annurev.bi.46.070177.002125. [DOI] [PubMed] [Google Scholar]
- Yazyu H., Shiota-Niiya S., Shimamoto T., Kanazawa H., Futai M., Tsuchiya T. Nucleotide sequence of the melB gene and characteristics of deduced amino acid sequence of the melibiose carrier in Escherichia coli. J Biol Chem. 1984 Apr 10;259(7):4320–4326. [PubMed] [Google Scholar]
- Zilberstein D., Agmon V., Schuldiner S., Padan E. The sodium/proton antiporter is part of the pH homeostasis mechanism in Escherichia coli. J Biol Chem. 1982 Apr 10;257(7):3687–3691. [PubMed] [Google Scholar]
- Zilberstein D., Ophir I. J., Padan E., Schuldiner S. Na+ gradient-coupled porters of EScherichia coli share a common subunit. J Biol Chem. 1982 Apr 10;257(7):3692–3696. [PubMed] [Google Scholar]
- de Vries W., Rietveld-Struijk R. M., Stouthamer A. H. ATP formation associated with fumarate and nitrate reduction in growing cultures of Veillonella alcalescens. Antonie Van Leeuwenhoek. 1977;43(2):153–167. doi: 10.1007/BF00395670. [DOI] [PubMed] [Google Scholar]