Abstract
Sugars such as glucose are transported into Escherichia coli by a coupled phosphorylation mechanism (the phosphoenolpyruvate:sugar phosphotransferase system, PTS). Transport of sugars through the PTS results in inhibition of adenylate cyclase [ATP pyrophosphate-lyase (cyclizing), EC 4.6.1.1] activity by a mechanism involving a change in the state of phosphorylation of PTS proteins. Other sugars (e.g., lactose) are transported without modification by a mechanism involving proton cotransport, which requires a proton motive force across the cell membrane. We show here that uptake of sugars through the lactose transport system results in inhibition of adenylate cyclase activity if the proton symport mechanism is also active. The protonophore carbonyl cyanide m-chlorophenylhydrazone also inhibits adenylate cyclase activity. These data suggest that the steady-state electrochemical proton gradient regulates the activity of adenylate cyclase. We propose that sugar-dependent inhibition of adenylate cyclase activity may occur by either of two mechanisms. Sugars transported by the PTS inhibited adenylate cyclase activity by dephosphorylation of a regulatory protein, while sugars transported by the proton motive force system inhibit adenylate cyclase activity as a result of collapse of the proton electrochemical gradient.
Full text
PDF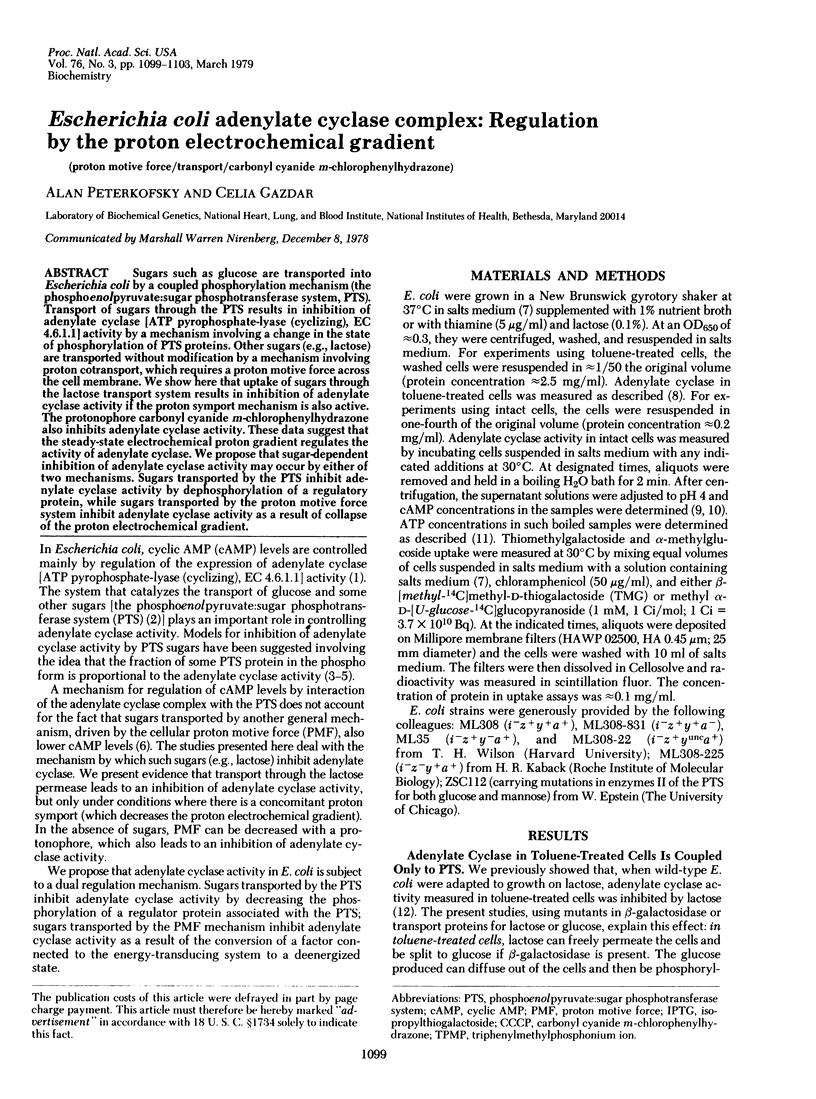
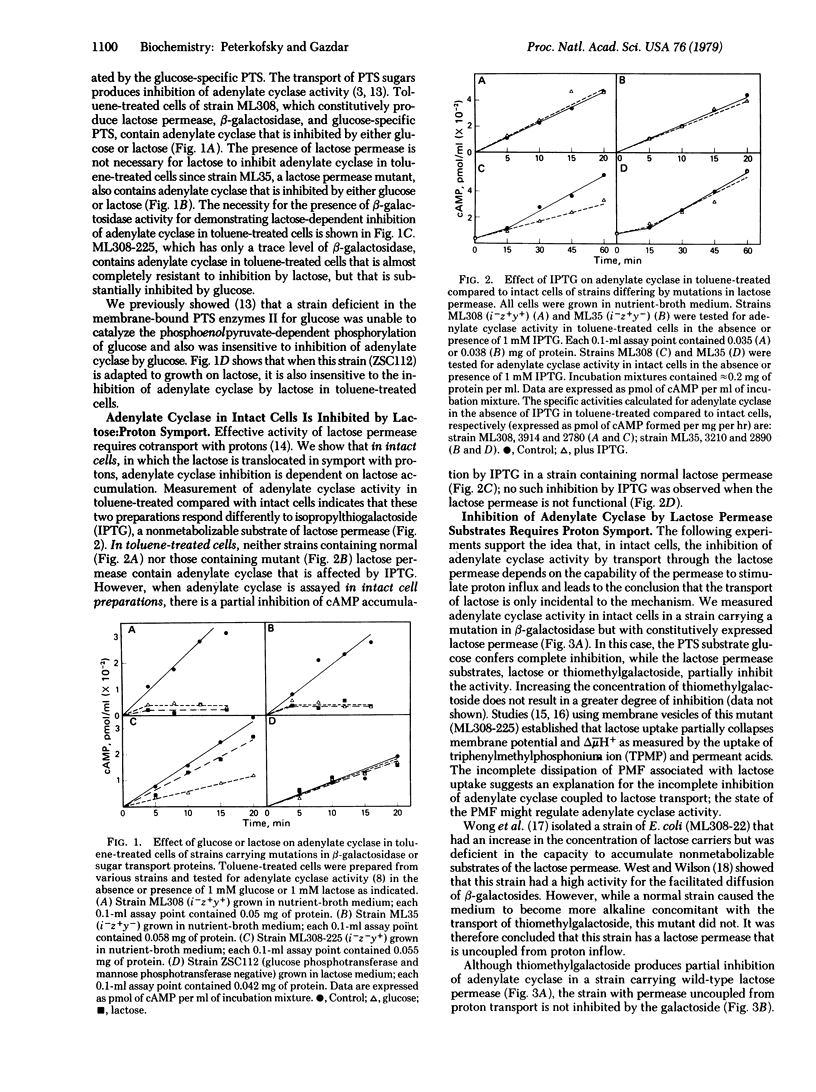
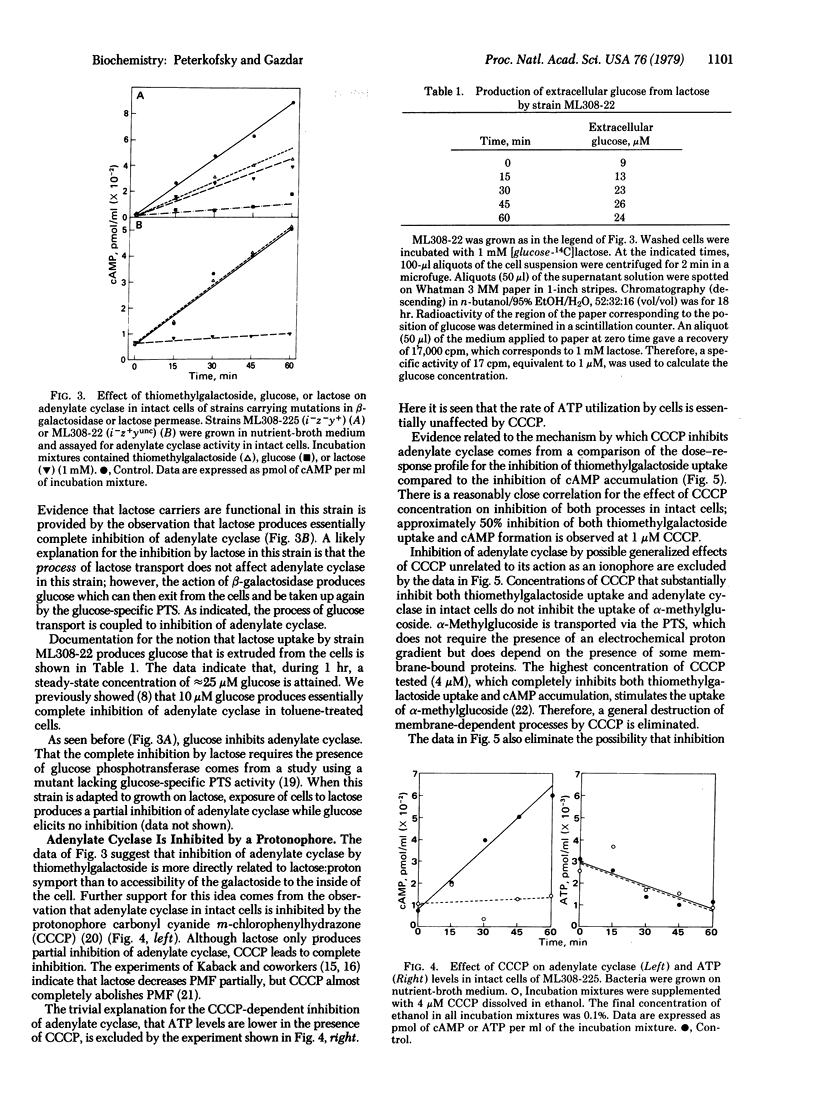
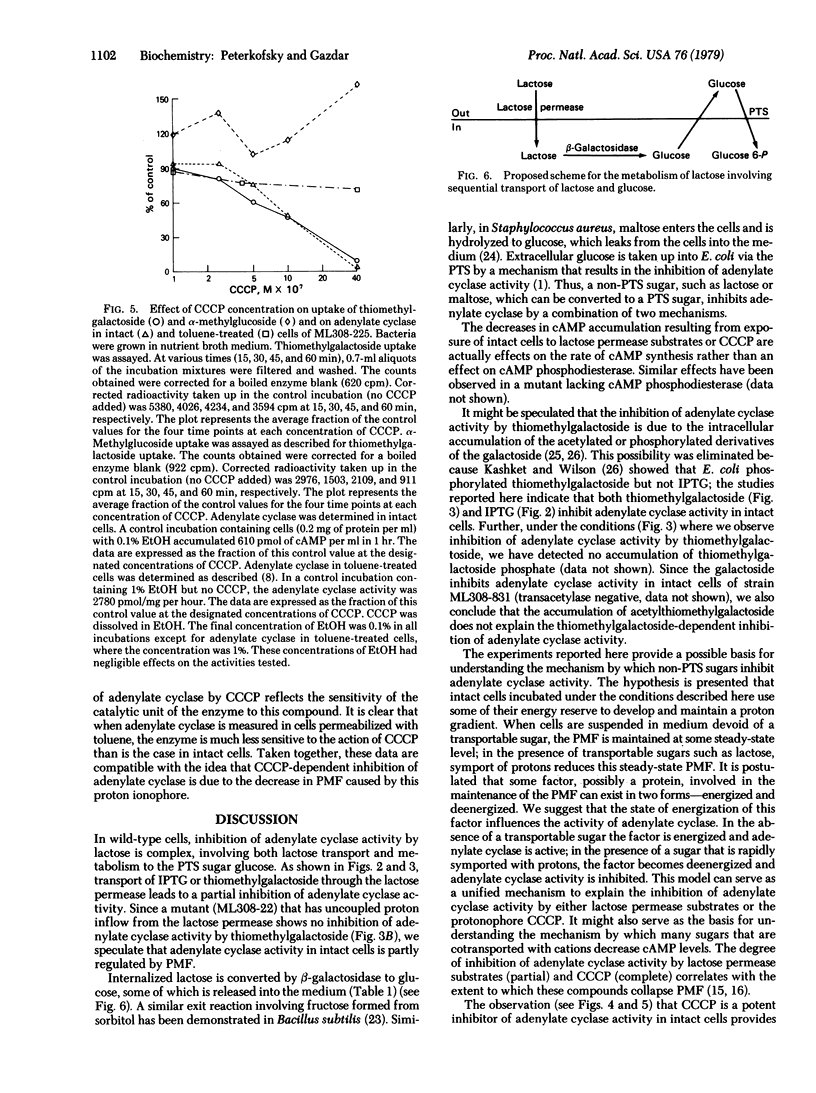
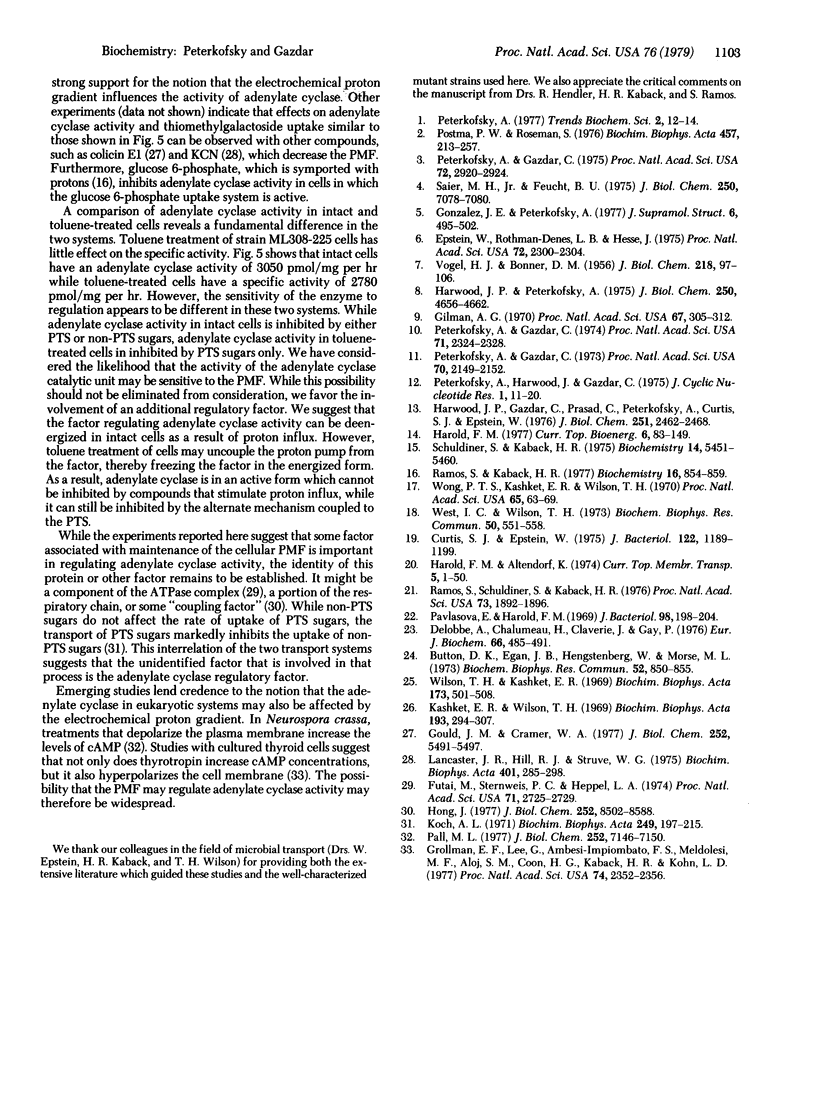
Selected References
These references are in PubMed. This may not be the complete list of references from this article.
- Button D. K., Egan J. B., Hengstenberg W., Morse M. L. Carbohydrate transport in Staphylococcus aureus. IV. Maltose accumulation and metabolism. Biochem Biophys Res Commun. 1973 Jun 8;52(3):850–855. doi: 10.1016/0006-291x(73)91015-2. [DOI] [PubMed] [Google Scholar]
- Curtis S. J., Epstein W. Phosphorylation of D-glucose in Escherichia coli mutants defective in glucosephosphotransferase, mannosephosphotransferase, and glucokinase. J Bacteriol. 1975 Jun;122(3):1189–1199. doi: 10.1128/jb.122.3.1189-1199.1975. [DOI] [PMC free article] [PubMed] [Google Scholar]
- Delobbe A., Chalumeau H., Claverie J. M., Gay P. Phosphorylation of intracellular fructose in Bacillus subtilis mediated by phosphoenolpyruvate-1-fructose phosphotransferase. Eur J Biochem. 1976 Jul 15;66(3):485–491. doi: 10.1111/j.1432-1033.1976.tb10573.x. [DOI] [PubMed] [Google Scholar]
- Epstein W., Rothman-Denes L. B., Hesse J. Adenosine 3':5'-cyclic monophosphate as mediator of catabolite repression in Escherichia coli. Proc Natl Acad Sci U S A. 1975 Jun;72(6):2300–2304. doi: 10.1073/pnas.72.6.2300. [DOI] [PMC free article] [PubMed] [Google Scholar]
- Futai M., Sternweis P. C., Heppel L. A. Purification and properties of reconstitutively active and inactive adenosinetriphosphatase from Escherichia coli. Proc Natl Acad Sci U S A. 1974 Jul;71(7):2725–2729. doi: 10.1073/pnas.71.7.2725. [DOI] [PMC free article] [PubMed] [Google Scholar]
- Gilman A. G. A protein binding assay for adenosine 3':5'-cyclic monophosphate. Proc Natl Acad Sci U S A. 1970 Sep;67(1):305–312. doi: 10.1073/pnas.67.1.305. [DOI] [PMC free article] [PubMed] [Google Scholar]
- Gonzalez J. E., Peterkofsky A. The mechanism of sugar-dependent repression of synthesis of catabolic enzymes in Escherichia coli. J Supramol Struct. 1977;6(4):495–502. doi: 10.1002/jss.400060404. [DOI] [PubMed] [Google Scholar]
- Gould J. M., Cramer W. A. Studies on the depolarization of the Escherichia coli cell membrane by colicin E1. J Biol Chem. 1977 Aug 10;252(15):5491–5497. [PubMed] [Google Scholar]
- Grollman E. F., Lee G., Ambesi-Impiombato F. S., Meldolesi M. F., Aloj S. M., Coon H. G., Kaback H. R., Kohn L. D. Effects of thyrotropin on the thyroid cell membrane: hyperpolarization induced by hormone-receptor interaction. Proc Natl Acad Sci U S A. 1977 Jun;74(6):2352–2356. doi: 10.1073/pnas.74.6.2352. [DOI] [PMC free article] [PubMed] [Google Scholar]
- Harwood J. P., Gazdar C., Prasad C., Peterkofsky A., Curtis S. J., Epstein W. Involvement of the glucose enzymes II of the sugar phosphotransferase system in the regulation of adenylate cyclase by glucose in Escherichia coli. J Biol Chem. 1976 Apr 25;251(8):2462–2468. [PubMed] [Google Scholar]
- Harwood J. P., Peterkofsky A. Glucose-sensitive adenylate cyclase in toluene-treated cells of Escherichia coli B. J Biol Chem. 1975 Jun 25;250(12):4656–4662. [PubMed] [Google Scholar]
- Hong J. S. An ecf mutation in Escherichia coli pleiotropically affecting energy coupling in active transport but not generation or maintenance of membrane potential. J Biol Chem. 1977 Dec 10;252(23):8582–8588. [PubMed] [Google Scholar]
- Kashket E. R., Wilson T. H. Isolation and properties of mutants of Escherichia coli with increased phosphorylations of thiomethyl-beta-galactoside. Biochim Biophys Acta. 1969;193(2):294–307. doi: 10.1016/0005-2736(69)90190-4. [DOI] [PubMed] [Google Scholar]
- Koch A. L. Local and non-local interactions of fluxes mediated by the glucose and galactoside permeases of Escherichia coli. Biochim Biophys Acta. 1971 Oct 12;249(1):197–215. doi: 10.1016/0005-2736(71)90097-6. [DOI] [PubMed] [Google Scholar]
- Lancaster J. R., Jr, Hill R. J., Struve W. G. The characterization of energized and partially de-energized (respiration-independent) beta-galactoside transport into Escherichia coli. Biochim Biophys Acta. 1975 Aug 20;401(2):285–298. doi: 10.1016/0005-2736(75)90312-0. [DOI] [PubMed] [Google Scholar]
- Pall M. L. Cyclic AMP and the plasma membrane potential in Neurospora crassa. J Biol Chem. 1977 Oct 25;252(20):7146–7150. [PubMed] [Google Scholar]
- Pavlasova E., Harold F. M. Energy coupling in the transport of beta-galactosides by Escherichia coli: effect of proton conductors. J Bacteriol. 1969 Apr;98(1):198–204. doi: 10.1128/jb.98.1.198-204.1969. [DOI] [PMC free article] [PubMed] [Google Scholar]
- Peterkofsky A., Gazdar C. Glucose inhibition of adenylate cyclase in intact cells of Escherichia coli B. Proc Natl Acad Sci U S A. 1974 Jun;71(6):2324–2328. doi: 10.1073/pnas.71.6.2324. [DOI] [PMC free article] [PubMed] [Google Scholar]
- Peterkofsky A., Gazdar C. Interaction of enzyme I of the phosphoenolpyruvate:sugar phosphotransferase system with adenylate cyclase of Escherichia coli. Proc Natl Acad Sci U S A. 1975 Aug;72(8):2920–2924. doi: 10.1073/pnas.72.8.2920. [DOI] [PMC free article] [PubMed] [Google Scholar]
- Peterkofsky A., Gazdar C. Measurements of rates of adenosine 3':5'-cyclic monophosphate synthesis in intact Escherichia coli B. Proc Natl Acad Sci U S A. 1973 Jul;70(7):2149–2152. doi: 10.1073/pnas.70.7.2149. [DOI] [PMC free article] [PubMed] [Google Scholar]
- Postma P. W., Roseman S. The bacterial phosphoenolpyruvate: sugar phosphotransferase system. Biochim Biophys Acta. 1976 Dec 14;457(3-4):213–257. doi: 10.1016/0304-4157(76)90001-0. [DOI] [PubMed] [Google Scholar]
- Ramos S., Kaback H. R. The relationship between the electrochemical proton gradient and active transport in Escherichia coli membrane vesicles. Biochemistry. 1977 Mar 8;16(5):854–859. doi: 10.1021/bi00624a007. [DOI] [PubMed] [Google Scholar]
- Ramos S., Schuldiner S., Kaback H. R. The electrochemical gradient of protons and its relationship to active transport in Escherichia coli membrane vesicles. Proc Natl Acad Sci U S A. 1976 Jun;73(6):1892–1896. doi: 10.1073/pnas.73.6.1892. [DOI] [PMC free article] [PubMed] [Google Scholar]
- Saier M. H., Jr, Feucht B. U. Coordinate regulation of adenylate cyclase and carbohydrate permeases by the phosphoenolpyruvate:sugar phosphotransferase system in Salmonella typhimurium. J Biol Chem. 1975 Sep 10;250(17):7078–7080. [PubMed] [Google Scholar]
- Schuldiner S., Kaback H. R. Membrane potential and active transport in membrane vesicles from Escherichia coli. Biochemistry. 1975 Dec 16;14(25):5451–5461. doi: 10.1021/bi00696a011. [DOI] [PubMed] [Google Scholar]
- VOGEL H. J., BONNER D. M. Acetylornithinase of Escherichia coli: partial purification and some properties. J Biol Chem. 1956 Jan;218(1):97–106. [PubMed] [Google Scholar]
- West I. C., Wilson T. H. Galactoside transport dissociated from proton movement in mutants of Escherichia coli. Biochem Biophys Res Commun. 1973 Jan 23;50(2):551–558. doi: 10.1016/0006-291x(73)90875-9. [DOI] [PubMed] [Google Scholar]
- Wilson T. H., Kashket E. R. Isolation and properties of thiogalactoside transacetylase-negative mutants of Escherichia coli. Biochim Biophys Acta. 1969 Apr;173(3):501–508. doi: 10.1016/0005-2736(69)90014-5. [DOI] [PubMed] [Google Scholar]
- Wong P. T., Kashket E. R., Wilson T. H. Energy coupling in the lactose transport system of Escherichia coli. Proc Natl Acad Sci U S A. 1970 Jan;65(1):63–69. doi: 10.1073/pnas.65.1.63. [DOI] [PMC free article] [PubMed] [Google Scholar]