Abstract
Escherichia coli bacteria sensed the redox state in their surroundings and they swam to a niche that had a preferred reduction potential. In a spatial redox gradient of benzoquinone/benzoquinol, E. coli cells migrated to form a sharply defined band. Bacteria swimming out of either face of the band tumbled and returned to the preferred conditions at the site of the band. This behavioral response was named redox taxis. Redox molecules, such as substituted quinones, that elicited redox taxis, interact with the bacterial electron transport system, thereby altering electron transport and the proton motive force. The magnitude of the behavioral response was dependent on the reduction potential of the chemoeffector. The Tsr, Tar, Trg, Tap, and CheR proteins, which have a role in chemotaxis, were not essential for redox taxis. A cheB mutant had inverted responses in redox taxis, as previously demonstrated in aerotaxis. A model is proposed in which a redox effector molecule perturbs the electron transport system, and an unknown sensor in the membrane detects changes in the proton motive force or the redox status of the electron transport system, and transduces this information into a signal that regulates phosphorylation of the CheA protein. A similar mechanism has been proposed for aerotaxis. Redox taxis may play an important role in the distribution of bacterial species in natural environments.
Full text
PDF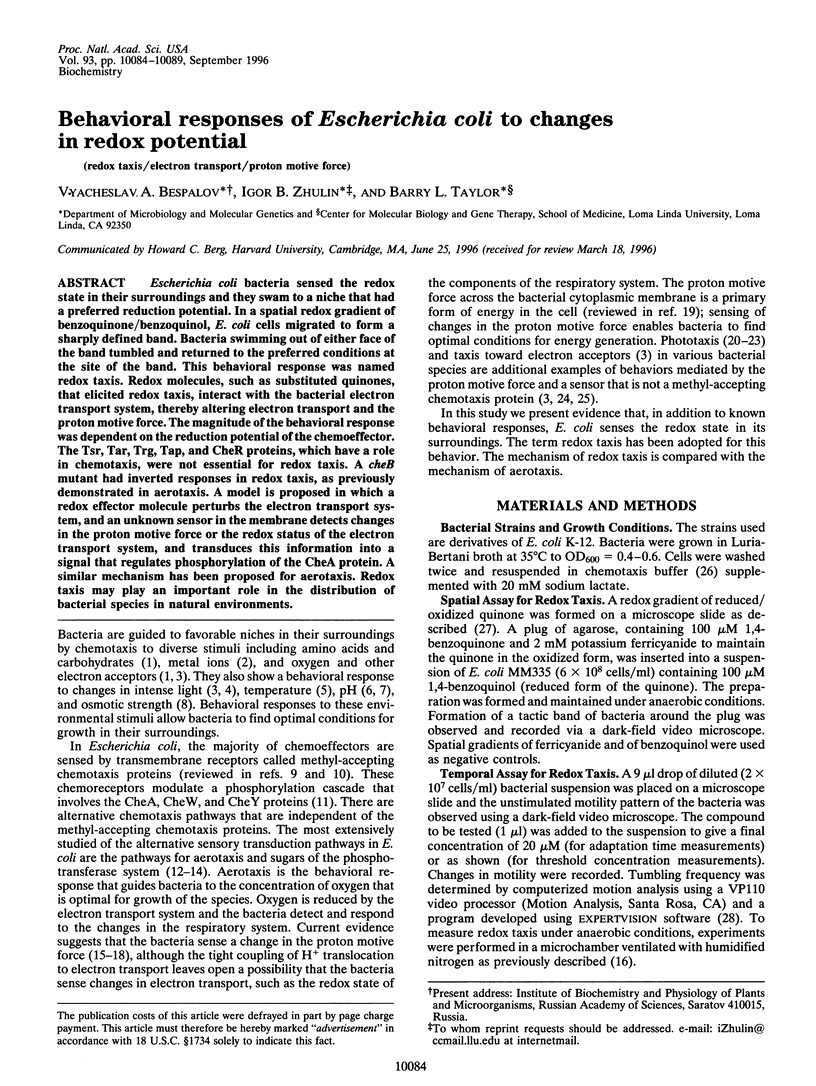
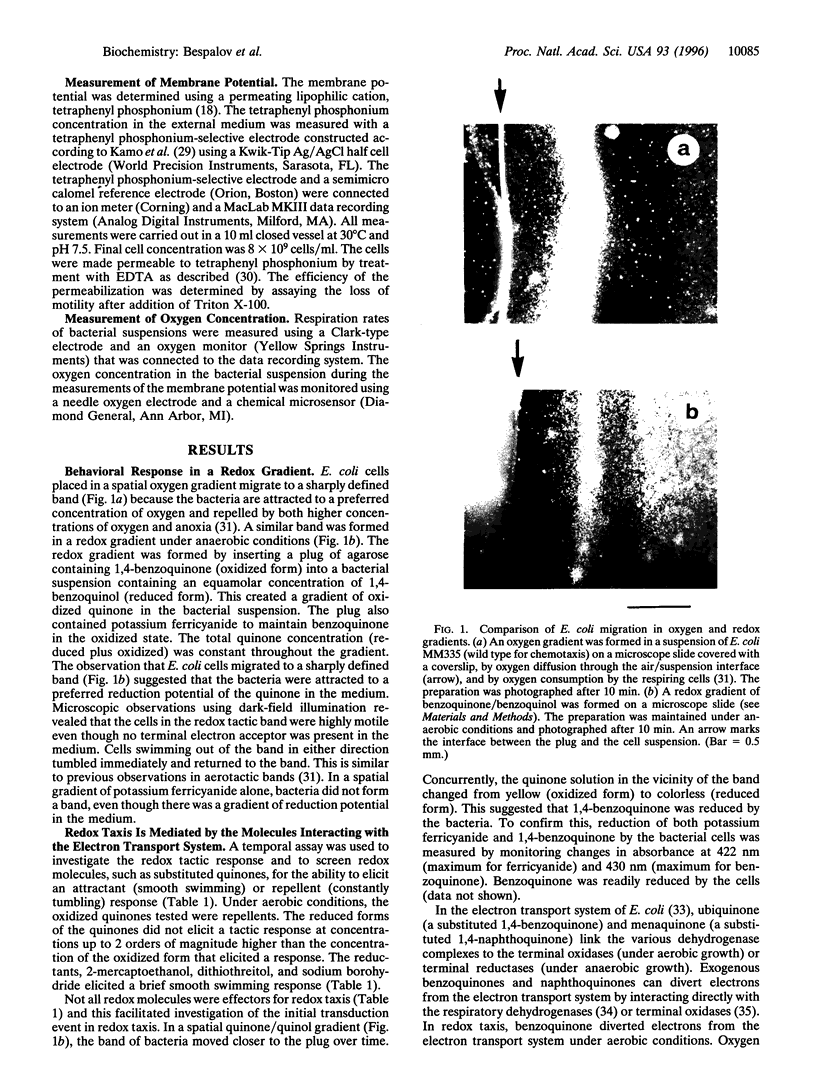
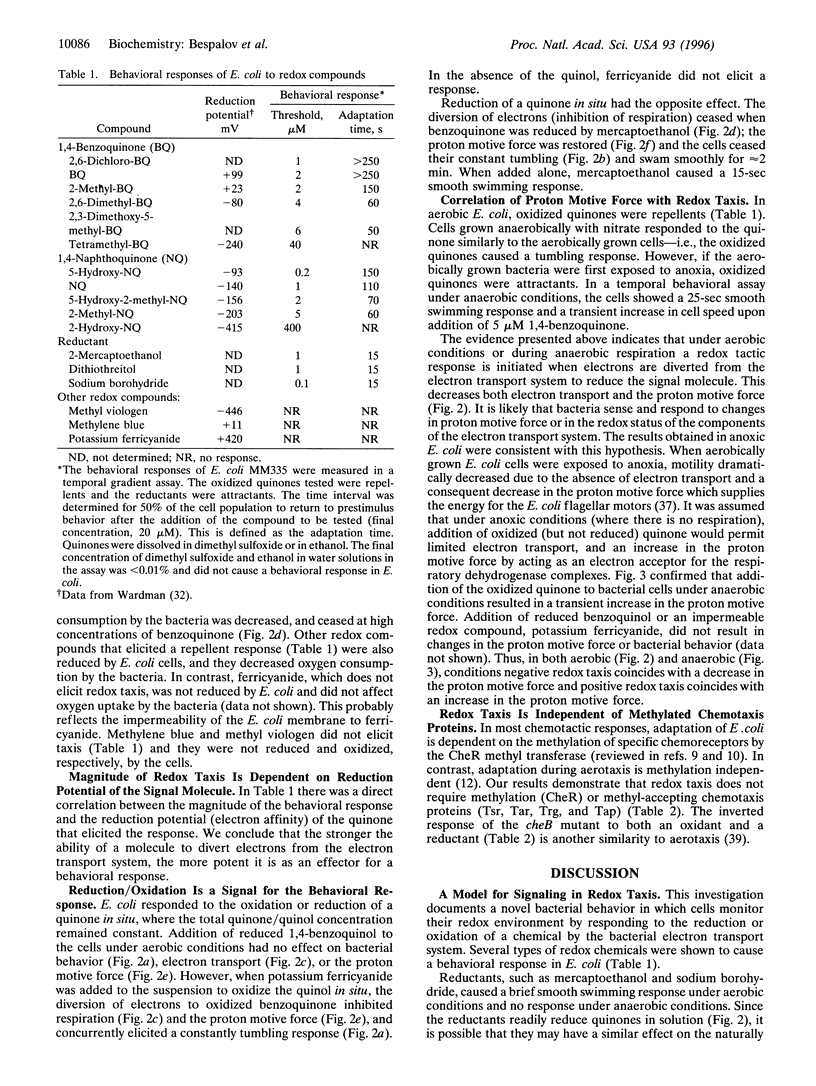
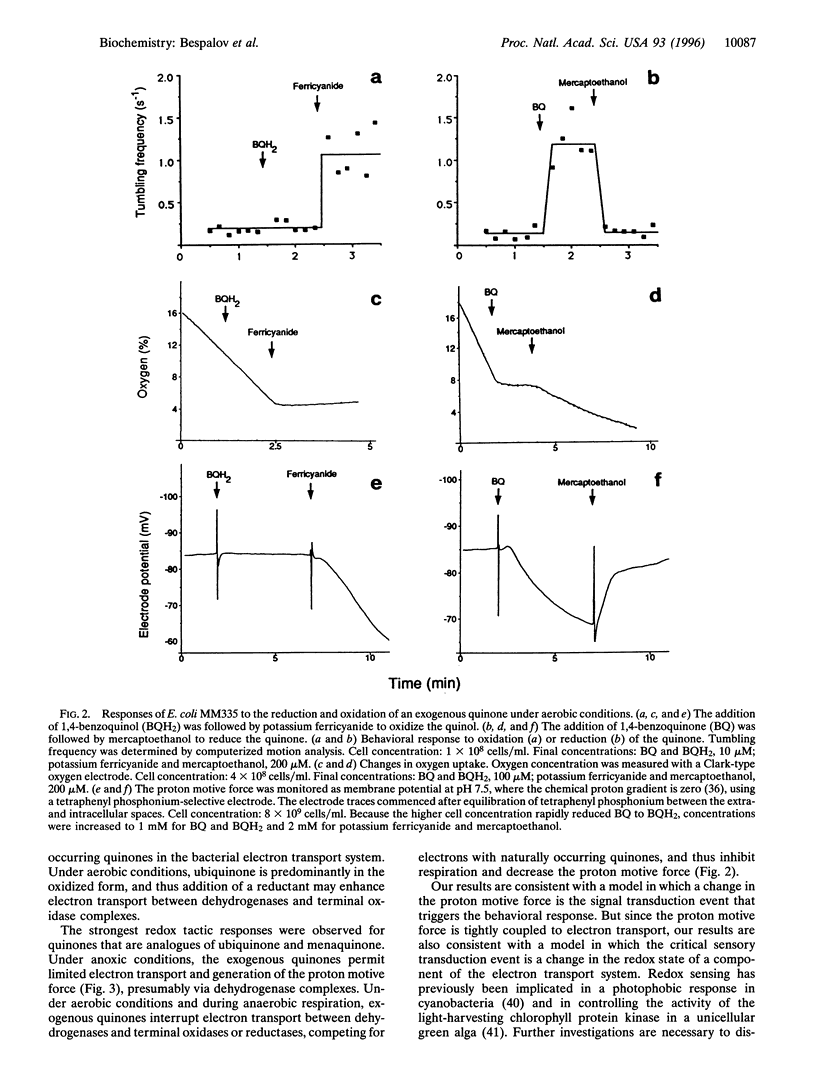
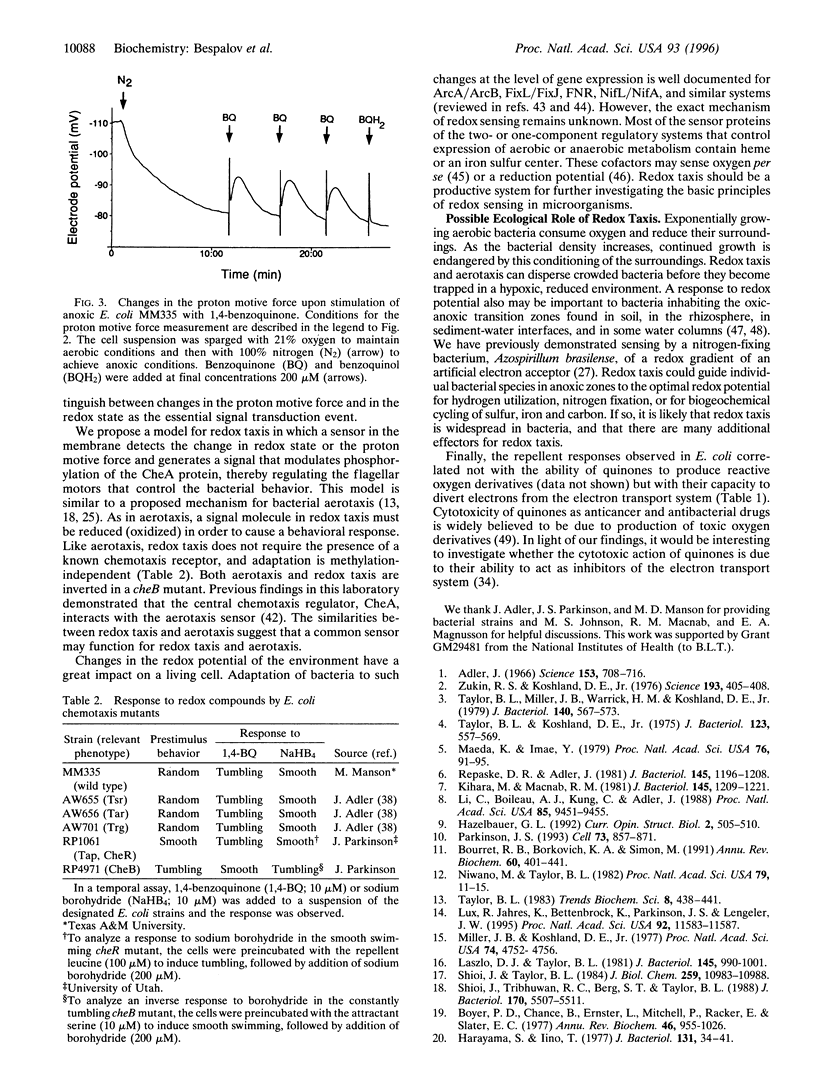
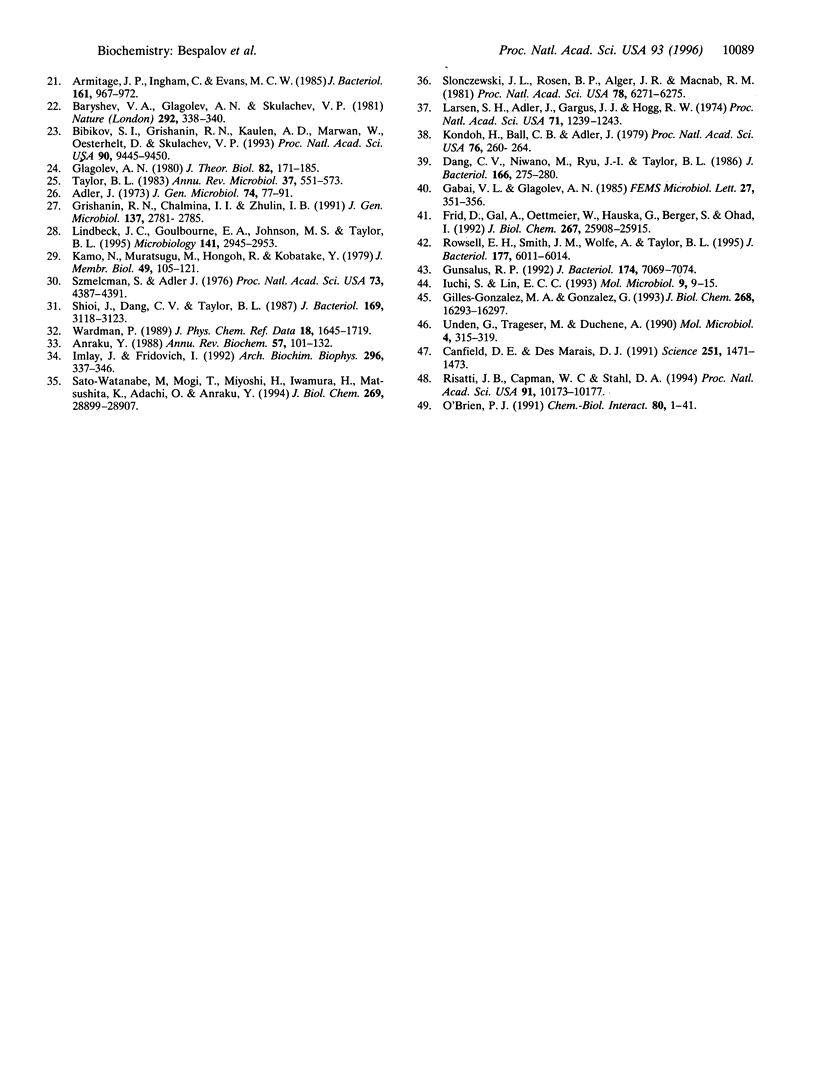
Images in this article
Selected References
These references are in PubMed. This may not be the complete list of references from this article.
- Adler J. A method for measuring chemotaxis and use of the method to determine optimum conditions for chemotaxis by Escherichia coli. J Gen Microbiol. 1973 Jan;74(1):77–91. doi: 10.1099/00221287-74-1-77. [DOI] [PubMed] [Google Scholar]
- Adler J. Chemotaxis in bacteria. Science. 1966 Aug 12;153(3737):708–716. doi: 10.1126/science.153.3737.708. [DOI] [PubMed] [Google Scholar]
- Anraku Y. Bacterial electron transport chains. Annu Rev Biochem. 1988;57:101–132. doi: 10.1146/annurev.bi.57.070188.000533. [DOI] [PubMed] [Google Scholar]
- Armitage J. P., Ingham C., Evans M. C. Role of proton motive force in phototactic and aerotactic responses of Rhodopseudomonas sphaeroides. J Bacteriol. 1985 Mar;161(3):967–972. doi: 10.1128/jb.161.3.967-972.1985. [DOI] [PMC free article] [PubMed] [Google Scholar]
- Bibikov S. I., Grishanin R. N., Kaulen A. D., Marwan W., Oesterhelt D., Skulachev V. P. Bacteriorhodopsin is involved in halobacterial photoreception. Proc Natl Acad Sci U S A. 1993 Oct 15;90(20):9446–9450. doi: 10.1073/pnas.90.20.9446. [DOI] [PMC free article] [PubMed] [Google Scholar]
- Bourret R. B., Borkovich K. A., Simon M. I. Signal transduction pathways involving protein phosphorylation in prokaryotes. Annu Rev Biochem. 1991;60:401–441. doi: 10.1146/annurev.bi.60.070191.002153. [DOI] [PubMed] [Google Scholar]
- Canfield D. E., Des Marais D. J. Aerobic sulfate reduction in microbial mats. Science. 1991 Mar 22;251:1471–1473. doi: 10.1126/science.11538266. [DOI] [PubMed] [Google Scholar]
- Dang C. V., Niwano M., Ryu J., Taylor B. L. Inversion of aerotactic response in Escherichia coli deficient in cheB protein methylesterase. J Bacteriol. 1986 Apr;166(1):275–280. doi: 10.1128/jb.166.1.275-280.1986. [DOI] [PMC free article] [PubMed] [Google Scholar]
- Frid D., Gal A., Oettmeier W., Hauska G., Berger S., Ohad I. The redox-controlled light-harvesting chlorophyll a/b protein kinase. Deactivation by substituted quinones. J Biol Chem. 1992 Dec 25;267(36):25908–25915. [PubMed] [Google Scholar]
- Gilles-Gonzalez M. A., Gonzalez G. Regulation of the kinase activity of heme protein FixL from the two-component system FixL/FixJ of Rhizobium meliloti. J Biol Chem. 1993 Aug 5;268(22):16293–16297. [PubMed] [Google Scholar]
- Glagolev A. N. Reception of the energy level in bacterial taxis. J Theor Biol. 1980 Jan 21;82(2):171–185. doi: 10.1016/0022-5193(80)90097-1. [DOI] [PubMed] [Google Scholar]
- Gunsalus R. P. Control of electron flow in Escherichia coli: coordinated transcription of respiratory pathway genes. J Bacteriol. 1992 Nov;174(22):7069–7074. doi: 10.1128/jb.174.22.7069-7074.1992. [DOI] [PMC free article] [PubMed] [Google Scholar]
- Harayama S., Iino T. Phototaxis and membrane potential in the photosynthetic bacterium Rhodospirillum rubrum. J Bacteriol. 1977 Jul;131(1):34–41. doi: 10.1128/jb.131.1.34-41.1977. [DOI] [PMC free article] [PubMed] [Google Scholar]
- Imlay J., Fridovich I. Exogenous quinones directly inhibit the respiratory NADH dehydrogenase in Escherichia coli. Arch Biochem Biophys. 1992 Jul;296(1):337–346. doi: 10.1016/0003-9861(92)90581-g. [DOI] [PubMed] [Google Scholar]
- Iuchi S., Lin E. C. Adaptation of Escherichia coli to redox environments by gene expression. Mol Microbiol. 1993 Jul;9(1):9–15. doi: 10.1111/j.1365-2958.1993.tb01664.x. [DOI] [PubMed] [Google Scholar]
- Kamo N., Muratsugu M., Hongoh R., Kobatake Y. Membrane potential of mitochondria measured with an electrode sensitive to tetraphenyl phosphonium and relationship between proton electrochemical potential and phosphorylation potential in steady state. J Membr Biol. 1979 Aug;49(2):105–121. doi: 10.1007/BF01868720. [DOI] [PubMed] [Google Scholar]
- Kihara M., Macnab R. M. Cytoplasmic pH mediates pH taxis and weak-acid repellent taxis of bacteria. J Bacteriol. 1981 Mar;145(3):1209–1221. doi: 10.1128/jb.145.3.1209-1221.1981. [DOI] [PMC free article] [PubMed] [Google Scholar]
- Kondoh H., Ball C. B., Adler J. Identification of a methyl-accepting chemotaxis protein for the ribose and galactose chemoreceptors of Escherichia coli. Proc Natl Acad Sci U S A. 1979 Jan;76(1):260–264. doi: 10.1073/pnas.76.1.260. [DOI] [PMC free article] [PubMed] [Google Scholar]
- Larsen S. H., Adler J., Gargus J. J., Hogg R. W. Chemomechanical coupling without ATP: the source of energy for motility and chemotaxis in bacteria. Proc Natl Acad Sci U S A. 1974 Apr;71(4):1239–1243. doi: 10.1073/pnas.71.4.1239. [DOI] [PMC free article] [PubMed] [Google Scholar]
- Laszlo D. J., Taylor B. L. Aerotaxis in Salmonella typhimurium: role of electron transport. J Bacteriol. 1981 Feb;145(2):990–1001. doi: 10.1128/jb.145.2.990-1001.1981. [DOI] [PMC free article] [PubMed] [Google Scholar]
- Li C., Boileau A. J., Kung C., Adler J. Osmotaxis in Escherichia coli. Proc Natl Acad Sci U S A. 1988 Dec;85(24):9451–9455. doi: 10.1073/pnas.85.24.9451. [DOI] [PMC free article] [PubMed] [Google Scholar]
- Lindbeck J. C., Goulbourne E. A., Jr, Johnson M. S., Taylor B. L. Aerotaxis in Halobacterium salinarium is methylation-dependent. Microbiology. 1995 Nov;141(Pt 11):2945–2953. doi: 10.1099/13500872-141-11-2945. [DOI] [PubMed] [Google Scholar]
- Lux R., Jahreis K., Bettenbrock K., Parkinson J. S., Lengeler J. W. Coupling the phosphotransferase system and the methyl-accepting chemotaxis protein-dependent chemotaxis signaling pathways of Escherichia coli. Proc Natl Acad Sci U S A. 1995 Dec 5;92(25):11583–11587. doi: 10.1073/pnas.92.25.11583. [DOI] [PMC free article] [PubMed] [Google Scholar]
- Maeda K., Imae Y. Thermosensory transduction in Escherichia coli: inhibition of the thermoresponse by L-serine. Proc Natl Acad Sci U S A. 1979 Jan;76(1):91–95. doi: 10.1073/pnas.76.1.91. [DOI] [PMC free article] [PubMed] [Google Scholar]
- Miller J. B., Koshland D. E., Jr Sensory electrophysiology of bacteria: relationship of the membrane potential to motility and chemotaxis in Bacillus subtilis. Proc Natl Acad Sci U S A. 1977 Nov;74(11):4752–4756. doi: 10.1073/pnas.74.11.4752. [DOI] [PMC free article] [PubMed] [Google Scholar]
- Niwano M., Taylor B. L. Novel sensory adaptation mechanism in bacterial chemotaxis to oxygen and phosphotransferase substrates. Proc Natl Acad Sci U S A. 1982 Jan;79(1):11–15. doi: 10.1073/pnas.79.1.11. [DOI] [PMC free article] [PubMed] [Google Scholar]
- O'Brien P. J. Molecular mechanisms of quinone cytotoxicity. Chem Biol Interact. 1991;80(1):1–41. doi: 10.1016/0009-2797(91)90029-7. [DOI] [PubMed] [Google Scholar]
- Parkinson J. S. Signal transduction schemes of bacteria. Cell. 1993 Jun 4;73(5):857–871. doi: 10.1016/0092-8674(93)90267-t. [DOI] [PubMed] [Google Scholar]
- Repaske D. R., Adler J. Change in intracellular pH of Escherichia coli mediates the chemotactic response to certain attractants and repellents. J Bacteriol. 1981 Mar;145(3):1196–1208. doi: 10.1128/jb.145.3.1196-1208.1981. [DOI] [PMC free article] [PubMed] [Google Scholar]
- Risatti J. B., Capman W. C., Stahl D. A. Community structure of a microbial mat: the phylogenetic dimension. Proc Natl Acad Sci U S A. 1994 Oct 11;91(21):10173–10177. doi: 10.1073/pnas.91.21.10173. [DOI] [PMC free article] [PubMed] [Google Scholar]
- Rowsell E. H., Smith J. M., Wolfe A., Taylor B. L. CheA, CheW, and CheY are required for chemotaxis to oxygen and sugars of the phosphotransferase system in Escherichia coli. J Bacteriol. 1995 Oct;177(20):6011–6014. doi: 10.1128/jb.177.20.6011-6014.1995. [DOI] [PMC free article] [PubMed] [Google Scholar]
- Sato-Watanabe M., Mogi T., Miyoshi H., Iwamura H., Matsushita K., Adachi O., Anraku Y. Structure-function studies on the ubiquinol oxidation site of the cytochrome bo complex from Escherichia coli using p-benzoquinones and substituted phenols. J Biol Chem. 1994 Nov 18;269(46):28899–28907. [PubMed] [Google Scholar]
- Shioi J., Dang C. V., Taylor B. L. Oxygen as attractant and repellent in bacterial chemotaxis. J Bacteriol. 1987 Jul;169(7):3118–3123. doi: 10.1128/jb.169.7.3118-3123.1987. [DOI] [PMC free article] [PubMed] [Google Scholar]
- Shioi J., Taylor B. L. Oxygen taxis and proton motive force in Salmonella typhimurium. J Biol Chem. 1984 Sep 10;259(17):10983–10988. [PubMed] [Google Scholar]
- Shioi J., Tribhuwan R. C., Berg S. T., Taylor B. L. Signal transduction in chemotaxis to oxygen in Escherichia coli and Salmonella typhimurium. J Bacteriol. 1988 Dec;170(12):5507–5511. doi: 10.1128/jb.170.12.5507-5511.1988. [DOI] [PMC free article] [PubMed] [Google Scholar]
- Slater E. C. Mechanism of oxidative phosphorylation. Annu Rev Biochem. 1977;46:1015–1026. doi: 10.1146/annurev.bi.46.070177.005055. [DOI] [PMC free article] [PubMed] [Google Scholar]
- Slonczewski J. L., Rosen B. P., Alger J. R., Macnab R. M. pH homeostasis in Escherichia coli: measurement by 31P nuclear magnetic resonance of methylphosphonate and phosphate. Proc Natl Acad Sci U S A. 1981 Oct;78(10):6271–6275. doi: 10.1073/pnas.78.10.6271. [DOI] [PMC free article] [PubMed] [Google Scholar]
- Szmelcman S., Adler J. Change in membrane potential during bacterial chemotaxis. Proc Natl Acad Sci U S A. 1976 Dec;73(12):4387–4391. doi: 10.1073/pnas.73.12.4387. [DOI] [PMC free article] [PubMed] [Google Scholar]
- Taylor B. L., Koshland D. E., Jr Intrinsic and extrinsic light responses of Salmonella typhimurium and Escherichia coli. J Bacteriol. 1975 Aug;123(2):557–569. doi: 10.1128/jb.123.2.557-569.1975. [DOI] [PMC free article] [PubMed] [Google Scholar]
- Taylor B. L., Miller J. B., Warrick H. M., Koshland D. E., Jr Electron acceptor taxis and blue light effect on bacterial chemotaxis. J Bacteriol. 1979 Nov;140(2):567–573. doi: 10.1128/jb.140.2.567-573.1979. [DOI] [PMC free article] [PubMed] [Google Scholar]
- Taylor B. L. Role of proton motive force in sensory transduction in bacteria. Annu Rev Microbiol. 1983;37:551–573. doi: 10.1146/annurev.mi.37.100183.003003. [DOI] [PubMed] [Google Scholar]
- Unden G., Trageser M., Duchêne A. Effect of positive redox potentials (greater than +400 mV) on the expression of anaerobic respiratory enzymes in Escherichia coli. Mol Microbiol. 1990 Feb;4(2):315–319. doi: 10.1111/j.1365-2958.1990.tb00598.x. [DOI] [PubMed] [Google Scholar]
- Zukin R. S., Koshland D. E., Jr Mg2+, Ca2+-dependent adenosine triphosphatase as receptor for divalent cations in bacterial sensing. Science. 1976 Jul 30;193(4251):405–408. doi: 10.1126/science.132702. [DOI] [PubMed] [Google Scholar]