Abstract
The addition of different detergents to Chlorella cells that had previously accumulated 6-deoxyglucose causes a rapid release of the hexose analogue into the medium. This effect is independent of the nature of the detergent and is observed only when the cells are in an energized state. Thus, in the presence of the uncoupler p-trifluoromethoxycarbonylcyanide phenylhydrazone or of inhibitors such as N-ethylmaleimide, the cells show a greatly reduced susceptibility towards detergents. Similarly, the detergent-induced loss of accumulated alpha-aminoisobutyric acid from Saccharomyces cerevisiae and of potassium from Escherichia coli is also strongly affected by the energy state of the cells. The differential susceptibility of energized and nonenergized cells was observed at all detergent concentrations tested. Measurements of substrate efflux at different concentrations of Triton indicated that only Triton monomers are responsible for the increase in permeability. The absorption of [14C]Triton X-100 by Chlorella and the binding of detergent to the cells were measured in the presence of metabolic inhibitors. Again, nonenergized cells bound a significantly lower amount of Triton X-100. The amphiphilic antibiotic nystatin produced effects on cell permeability similar to those of detergents, whereas toluene, which is apolar, gave opposite results.
Full text
PDF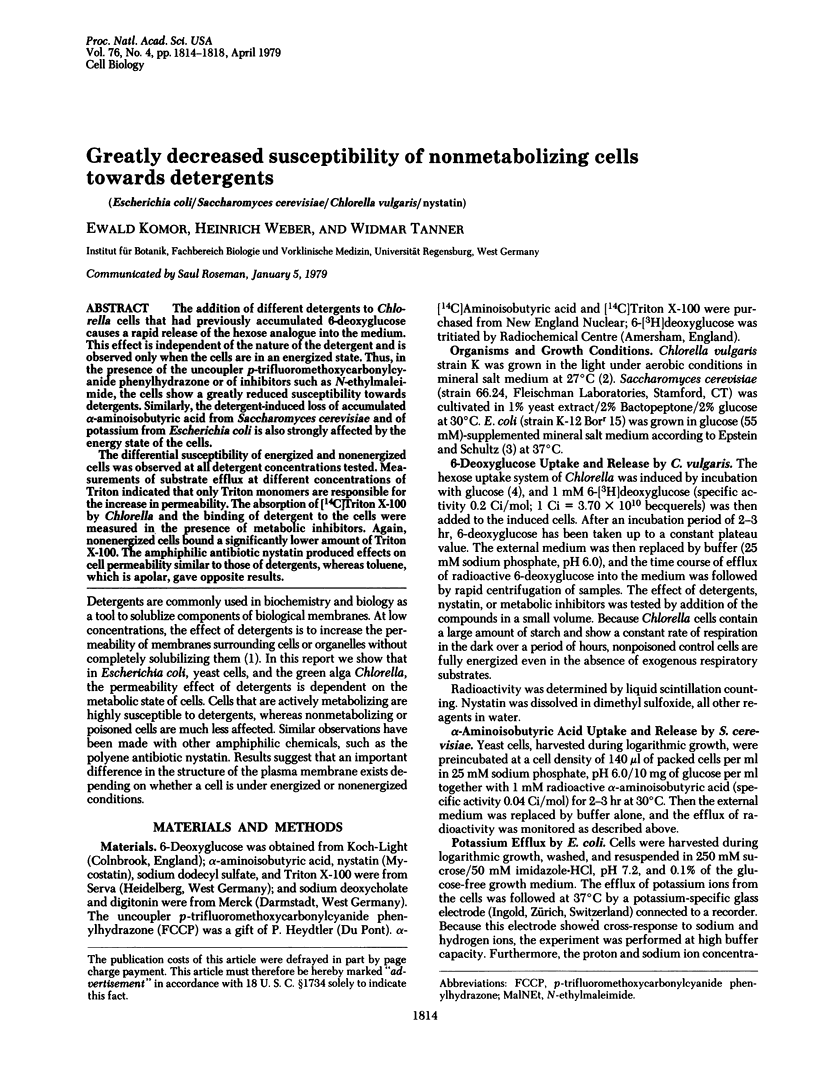
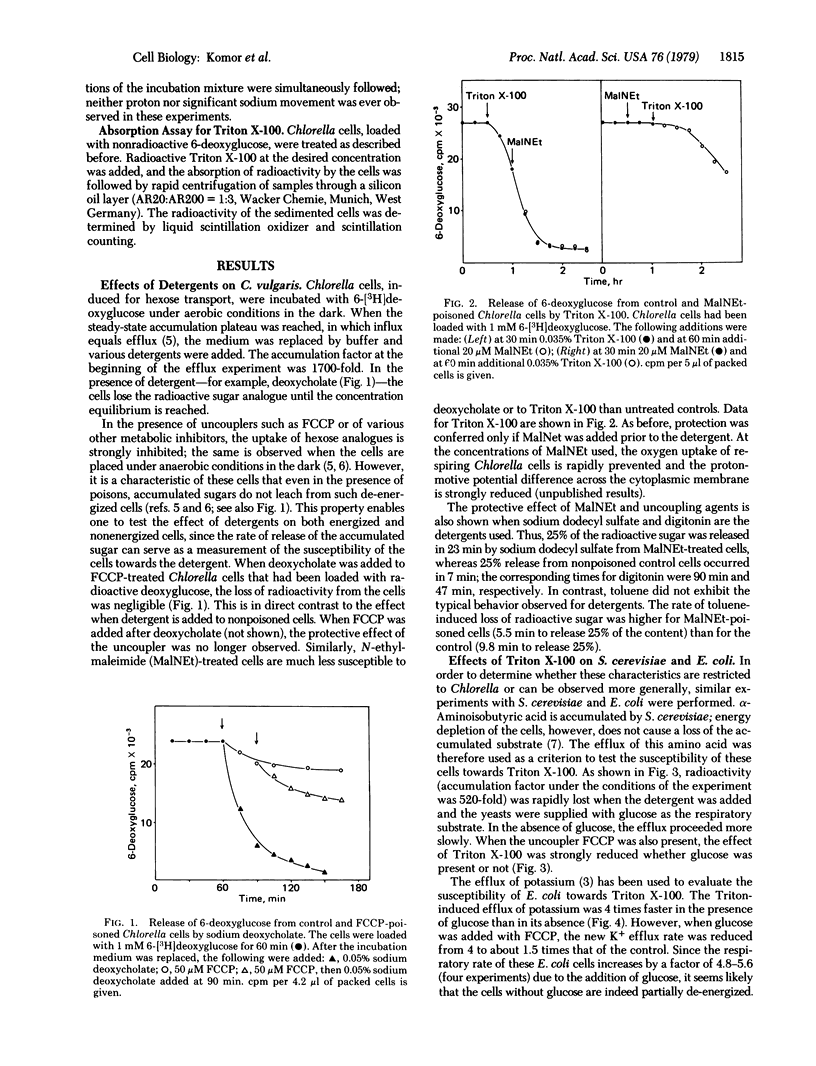
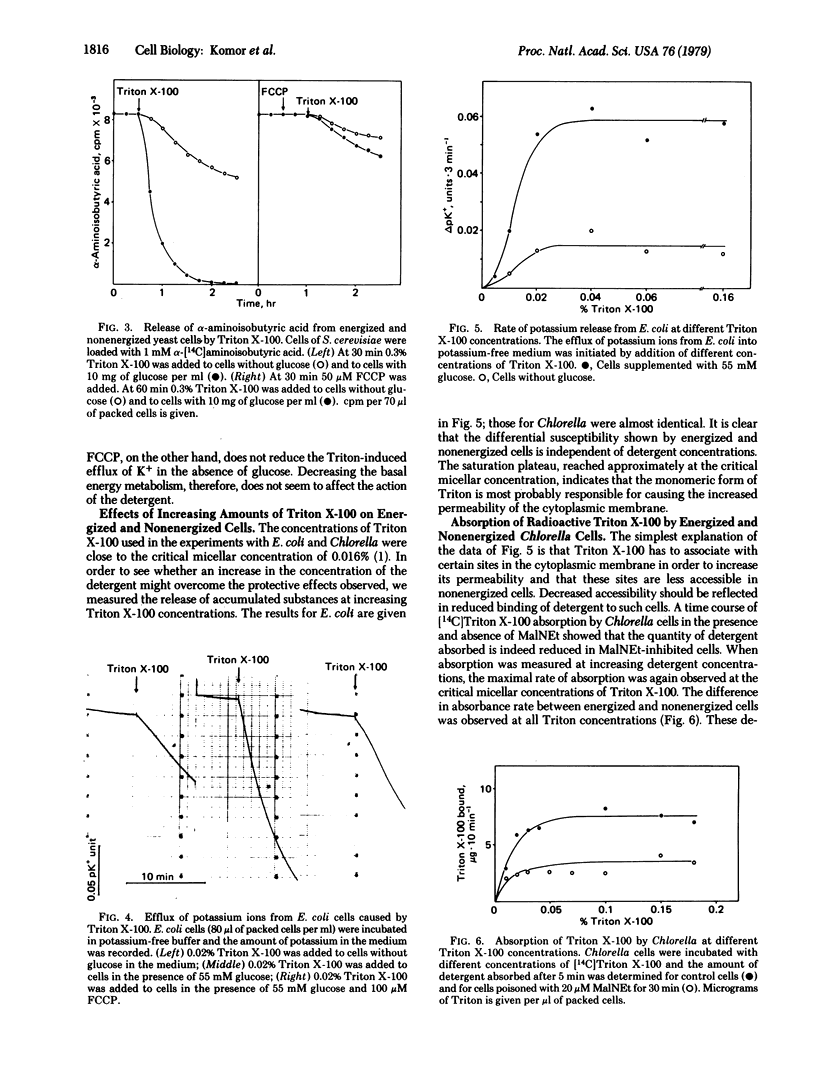
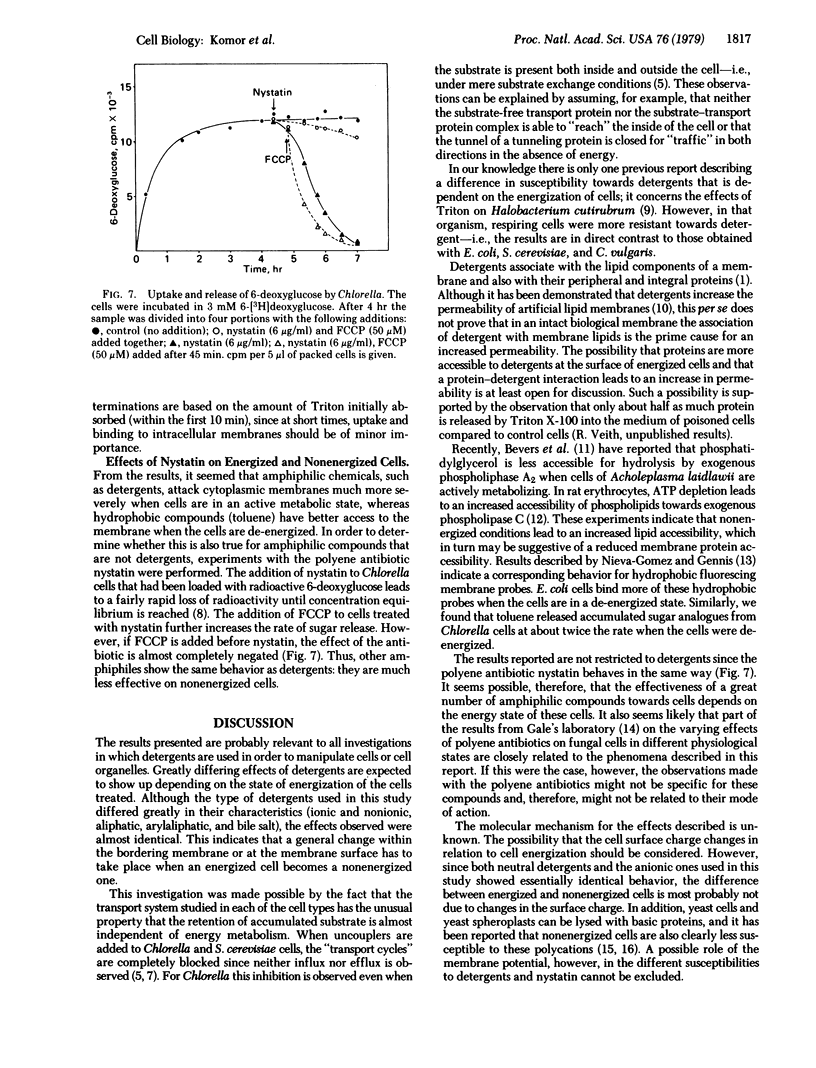
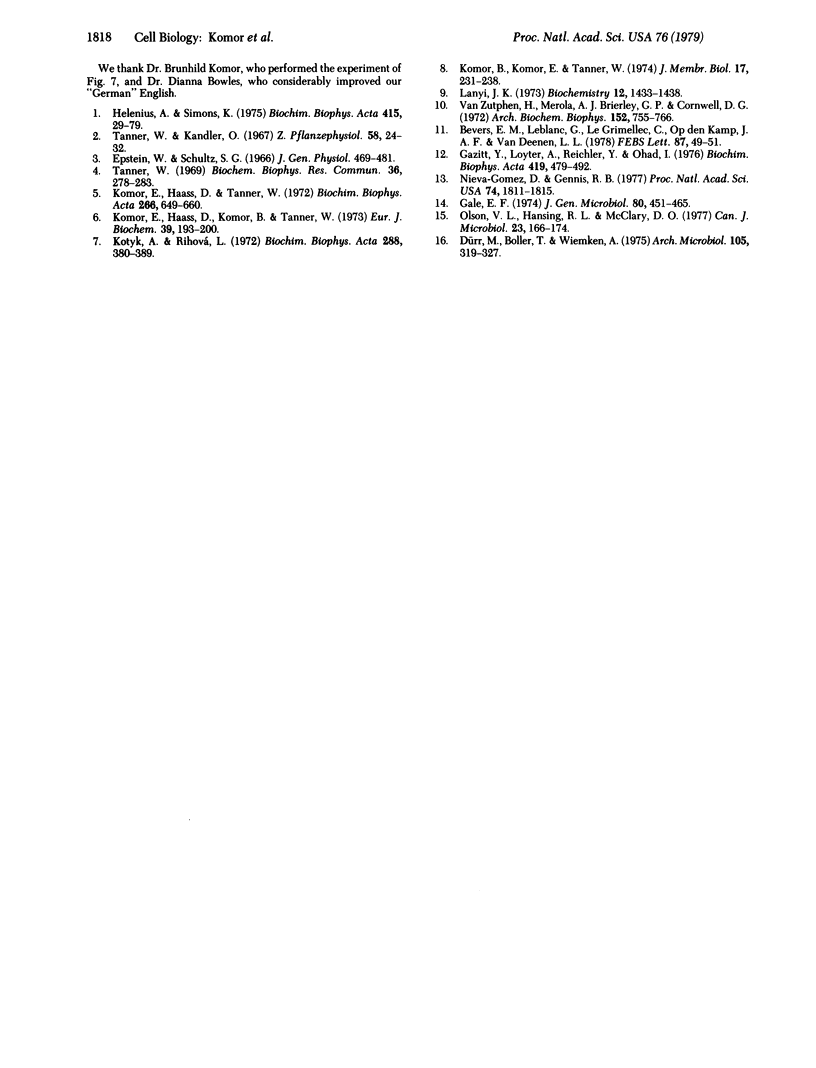
Selected References
These references are in PubMed. This may not be the complete list of references from this article.
- Bevers E. M., Leblanc G., Le Grimellec C., Op den Kamp J. A., van Deenen L. L. Disposition of phosphatidylglycerol in metabolizing cells of Acholeplasma laidlawii. FEBS Lett. 1978 Mar 1;87(1):49–51. doi: 10.1016/0014-5793(78)80130-6. [DOI] [PubMed] [Google Scholar]
- Dürr M., Boller T., Wiemken A. Polybase induced lysis of yeast spheroplasts. A new gentle method for preparation of vacuoles. Arch Microbiol. 1975 Nov 7;105(3):319–327. doi: 10.1007/BF00447152. [DOI] [PubMed] [Google Scholar]
- Epstein W., Schultz S. G. Cation transport in Escherichia coli. VI. K exchange. J Gen Physiol. 1966 Jan;49(3):469–481. doi: 10.1085/jgp.49.3.469. [DOI] [PMC free article] [PubMed] [Google Scholar]
- Gale E. F. The release of potassium ions from Candida albicans in the presence of polyene antibiotics. J Gen Microbiol. 1974 Feb;80(2):451–465. doi: 10.1099/00221287-80-2-451. [DOI] [PubMed] [Google Scholar]
- Gazitt Y., Loyter A., Reichler Y., Ohad I. Correlation between changes in the membrane organization and susceptibility to phospholipase C attack induced by ATP depletion of rat erythrocytes. Biochim Biophys Acta. 1976 Feb 6;419(3):479–492. doi: 10.1016/0005-2736(76)90260-1. [DOI] [PubMed] [Google Scholar]
- Helenius A., Simons K. Solubilization of membranes by detergents. Biochim Biophys Acta. 1975 Mar 25;415(1):29–79. doi: 10.1016/0304-4157(75)90016-7. [DOI] [PubMed] [Google Scholar]
- Komor B., Komor E., Tanner W. Transformation of a strictly coupled active transport system into a facilitated diffusion system by nystatin. J Membr Biol. 1974 Jul 12;17(3):231–238. doi: 10.1007/BF01870184. [DOI] [PubMed] [Google Scholar]
- Komor E., Haass D., Komor B., Tanner W. The active hexose-uptake system of Chlorella vulgaris. Km-values for 6-deoxyglucose influx and efflux and their contribution to sugar accumulation. Eur J Biochem. 1973 Nov 1;39(1):193–200. doi: 10.1111/j.1432-1033.1973.tb03117.x. [DOI] [PubMed] [Google Scholar]
- Komor E., Haass D., Tanner W. Unusual features of the active hexose uptake system of Chlorella vulgaris. Biochim Biophys Acta. 1972 Jun 20;266(3):649–660. doi: 10.1016/0006-3002(72)90008-x. [DOI] [PubMed] [Google Scholar]
- Kotyk A., Ríhová L. Transport of -aminoisobutyric acid in Saccharomyces cerevisiae. Biochim Biophys Acta. 1972 Nov 2;288(2):380–389. doi: 10.1016/0005-2736(72)90259-3. [DOI] [PubMed] [Google Scholar]
- Lanyi J. K. Influence of electron transport on the interaction between membrane lipids and Triton X-100 in Halobacterium cutirubrum. Biochemistry. 1973 Mar 27;12(7):1433–1438. doi: 10.1021/bi00731a025. [DOI] [PubMed] [Google Scholar]
- Nieva-Gomez D., Gennis R. B. Affinity of intact Escherichia coli for hydrophobic membrane probes is a function of the physiological state of the cells. Proc Natl Acad Sci U S A. 1977 May;74(5):1811–1815. doi: 10.1073/pnas.74.5.1811. [DOI] [PMC free article] [PubMed] [Google Scholar]
- Olson V. L., Hansing R. L., McClary D. O. The role of metabolic energy in the lethal action of basic proteins on Candida albicans. Can J Microbiol. 1977 Feb;23(2):166–174. doi: 10.1139/m77-024. [DOI] [PubMed] [Google Scholar]
- Tanner W. Light-driven active uptake of 3-O-methylglucose via an inducible hexose uptake system of Chlorella. Biochem Biophys Res Commun. 1969 Jul 23;36(2):278–283. doi: 10.1016/0006-291x(69)90326-x. [DOI] [PubMed] [Google Scholar]
- Van Zutphen H., Merola A. J., Brierley G. P., Cornwell D. G. The interaction of nonionic detergents with lipid bilayer membranes. Arch Biochem Biophys. 1972 Oct;152(2):755–766. doi: 10.1016/0003-9861(72)90271-8. [DOI] [PubMed] [Google Scholar]