Abstract
In cultured hamster cells starved for glucose for 24 hr there is an enhancement of the rate of α-aminoisobutyric acid transport (“shiftup”). When the starved cells are re-fed with glucose, the rate of transport shifts back down to the low, “regulated” rate typical of cells continuously fed with medium containing glucose (“shiftdown”). The high, deregulated rate of transport is maintained, however, when cycloheximide is present for 24 hr during the re-feeding with glucose. Maintenance of the high transport rate is evident only when the cells are incubated in amino acid-free medium just prior to the transport assay or when the assays are conducted with isolated membrane vesicles. A premature, pseudoshiftdown was observed in intact cells within as little as 2 hr after re-feeding when care was not taken to deplete the amino acid pool prior to the transport assay. In addition, a cycloheximide-insensitive increase in transport was observed when cultures were re-fed for 2 hr with amino acid-free medium containing fresh serum. These results emphasize the often overlooked precautions that should be taken to guard against artifacts that could mislead interpretations of amino acid transport data. More important, however, is the finding that Na+-dependent amino acid transport in cultured animal cells is regulated in part by a factor (or factors) that becomes inactivated when the cells are maintained under nonglycolytic culture conditions. In order to reactivate the control mechanism, starved cells that have been re-fed with glucose must resynthesize the regulatory factor(s). Thus, in at least cultured hamster cells, Na+-dependent amino acid transport regulation is much like the hexose transport regulation in that catabolic control (shiftdown) requires protein synthesis.
Keywords: α-aminoisobutyric acid, glucose shiftdown, membrane vesicles, cycloheximide
Full text
PDF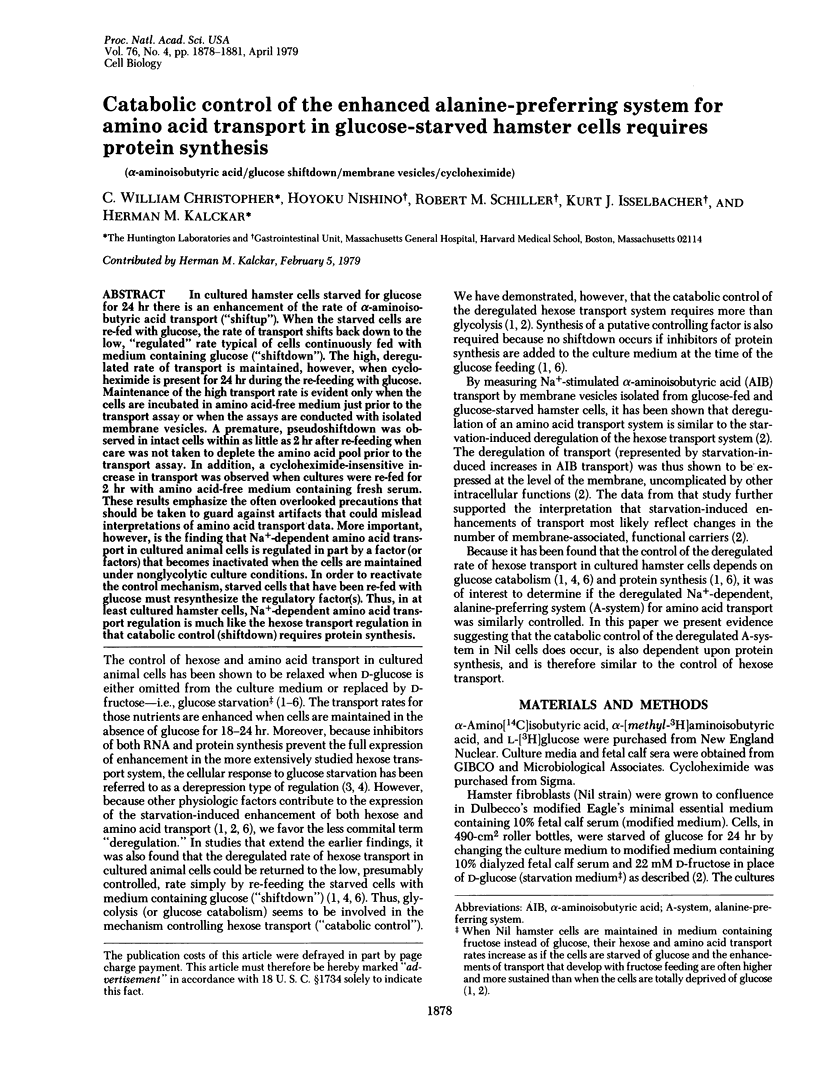
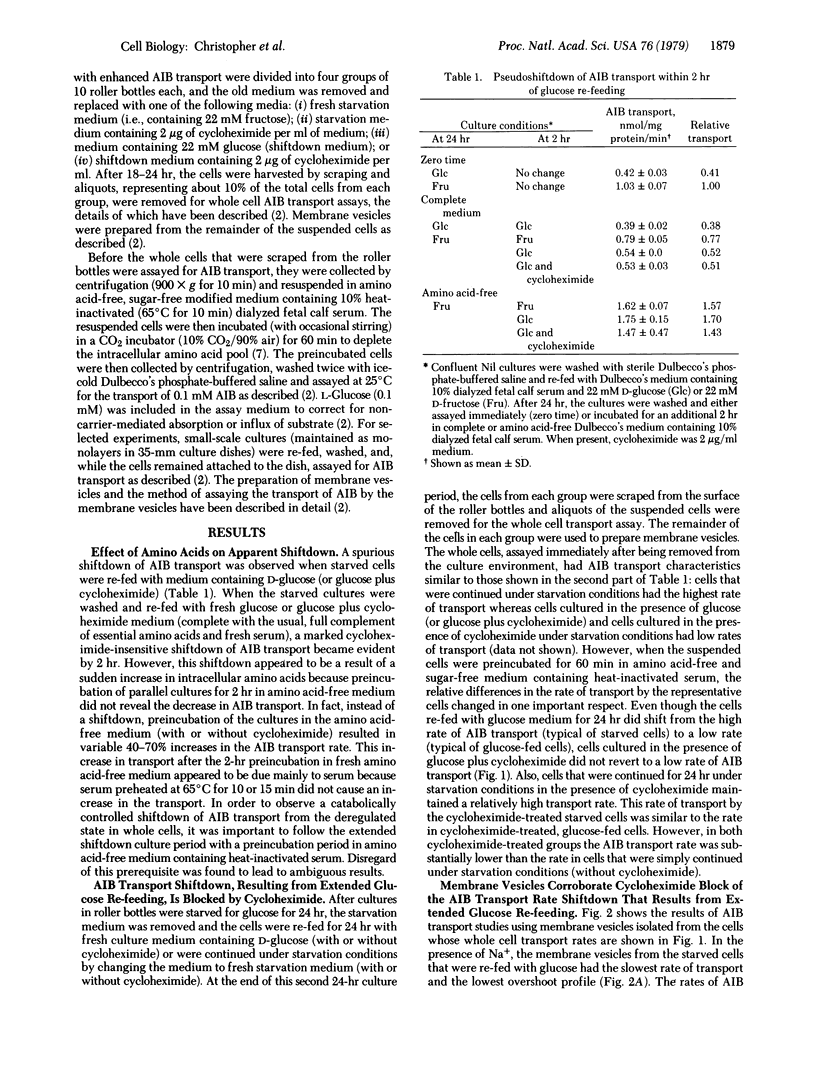
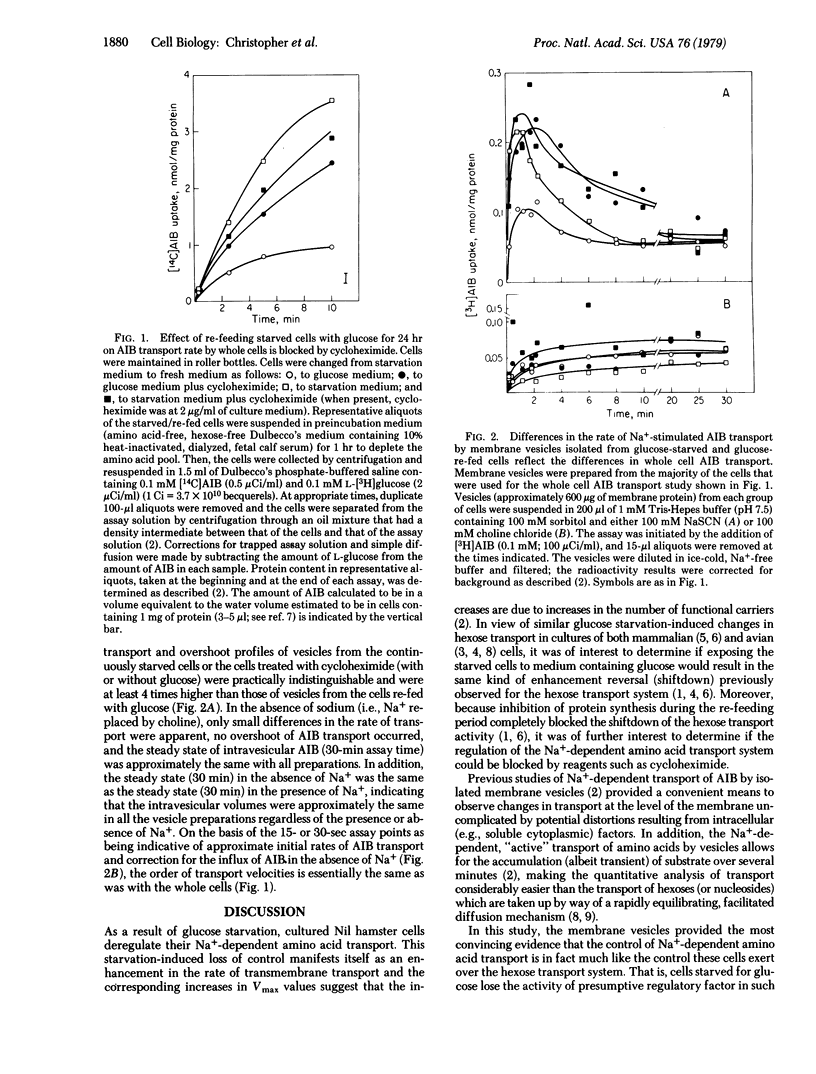
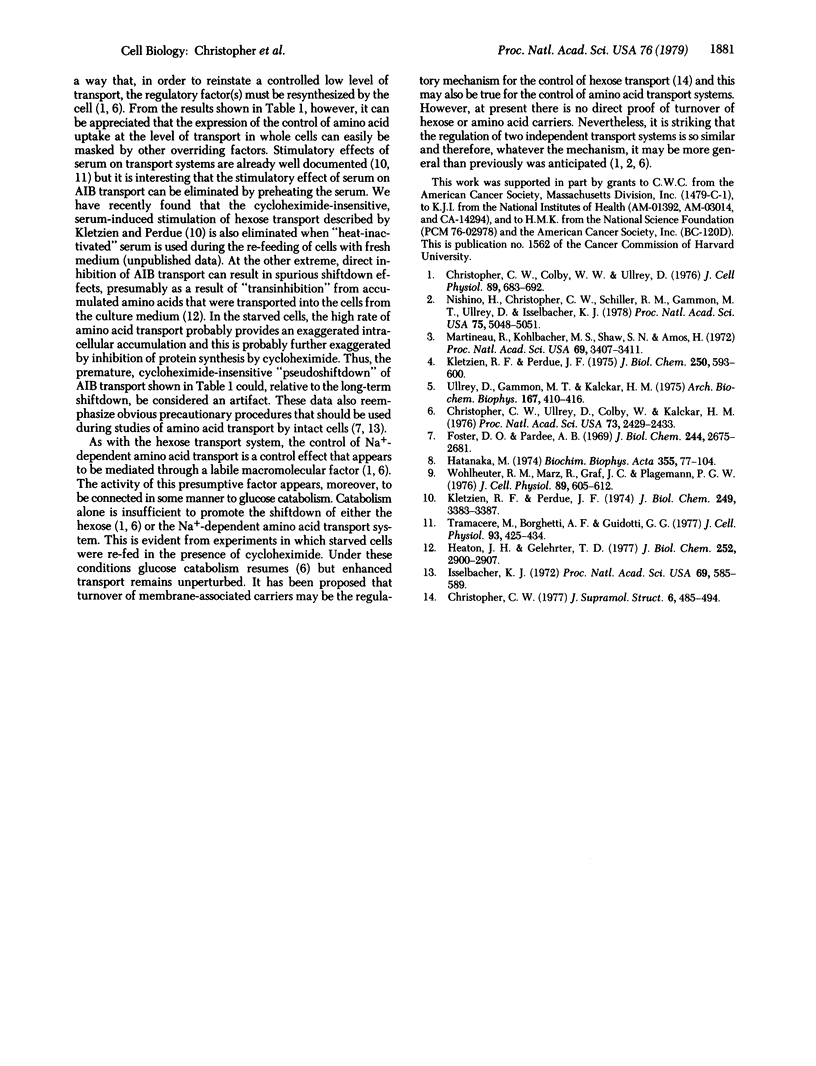
Selected References
These references are in PubMed. This may not be the complete list of references from this article.
- Christopher C. W., Colby W. W., Ullrey D. Derepression and carrier turnover: evidence for two distinct mechanisms of hexose transport regulation in animal cells. J Cell Physiol. 1976 Dec;89(4):683–692. doi: 10.1002/jcp.1040890427. [DOI] [PubMed] [Google Scholar]
- Christopher C. W. Hexose transport regulation in cultured hamster cells. J Supramol Struct. 1977;6(4):485–494. doi: 10.1002/jss.400060403. [DOI] [PubMed] [Google Scholar]
- Christopher C. W., Ullrey D., Colby W., Kalckar M. Paradoxical effects of cycloheximide and cytochalasin B on hamster cell hexose uptake. Proc Natl Acad Sci U S A. 1976 Jul;73(7):2429–2433. doi: 10.1073/pnas.73.7.2429. [DOI] [PMC free article] [PubMed] [Google Scholar]
- Foster D. O., Pardee A. B. Transport of amino acids by confluent and nonconfluent 3T3 and polyoma virus-transformed 3T3 cells growing on glass cover slips. J Biol Chem. 1969 May 25;244(10):2675–2681. [PubMed] [Google Scholar]
- Hatanaka M. Transport of sugars in tumor cell membranes. Biochim Biophys Acta. 1974 Apr 29;355(1):77–104. doi: 10.1016/0304-419x(74)90008-0. [DOI] [PubMed] [Google Scholar]
- Heaton J. H., Gelehrter T. D. Derepression of amino acid transport by amino acid starvation in rat hepatoma cells. J Biol Chem. 1977 May 10;252(9):2900–2907. [PubMed] [Google Scholar]
- Isselbacher K. J. Increased uptake of amino acids and 2-deoxy-D-glucose by virus-transformed cells in culture. Proc Natl Acad Sci U S A. 1972 Mar;69(3):585–589. doi: 10.1073/pnas.69.3.585. [DOI] [PMC free article] [PubMed] [Google Scholar]
- Kleitzien R. F., Perdue J. F. Sugar transport in chick embryo fibroblasts. 3. Evidence for post-transcriptional and post-translational regulation of transport following serum addition. J Biol Chem. 1974 Jun 10;249(11):3383–3387. [PubMed] [Google Scholar]
- Kletzien R. F., Perdue J. F. Induction of sugar transport in chick embryo fibroblasts by hexose starvation. Evidence for transcriptional regulation of transport. J Biol Chem. 1975 Jan 25;250(2):593–600. [PubMed] [Google Scholar]
- Martineau R., Kohlbacher M., Shaw S. N., Amos H. Enhancement of hexose entry into chick fibroblasts by starvation: differential effect on galactose and glucose. Proc Natl Acad Sci U S A. 1972 Nov;69(11):3407–3411. doi: 10.1073/pnas.69.11.3407. [DOI] [PMC free article] [PubMed] [Google Scholar]
- Nishino H., Christopher C. W., Schiller R. M., Gammon M. T., Ullrey D., Isselbacher K. J. Sodium-dependent amino acid transport by cultured hamster cells: membrane vesicles retain transport changes due to glucose starvation and cycloheximide. Proc Natl Acad Sci U S A. 1978 Oct;75(10):5048–5051. doi: 10.1073/pnas.75.10.5048. [DOI] [PMC free article] [PubMed] [Google Scholar]
- Tramacere M., Borghetti A. F., Guidotti G. G. Serum-mediated regulation of amino acid transport in cultured chick embryo fibroblasts. J Cell Physiol. 1977 Dec;93(3):425–433. doi: 10.1002/jcp.1040930314. [DOI] [PubMed] [Google Scholar]
- Ullrey D., Gammon M. T., Kalckar H. M. Uptake patterns and transport enhancements in cultures of hamster cells deprived of carbohydrates. Arch Biochem Biophys. 1975 Apr;167(2):410–416. doi: 10.1016/0003-9861(75)90481-6. [DOI] [PubMed] [Google Scholar]
- Wohlhueter R. M., Marz R., Graff J. C., Plagemann P. G. The application of rapid kinetic techniques to the transport of thymidine and 3-O-Methylglucose into Mammalian cells in suspension culture. J Cell Physiol. 1976 Dec;89(4):605–612. doi: 10.1002/jcp.1040890417. [DOI] [PubMed] [Google Scholar]