Abstract
The main function of white adipose tissue is to store nutrient energy in the form of triglycerides. The mechanism by which free fatty acids (FFA) move into and out of the adipocyte has not been resolved. We show here that changes in intracellular pH (pH1) in adipocytes correlate with the movement of FFA across cellular membranes as predicted by the Kamp and Hamilton model of passive diffusion of FFA. Exposure of fat cells to lipolytic agents or external FFA results is a rapid intracellular acidification that is reversed by metabolism of the FFA or its removal by albumin. In contrast, insulin causes an alkalinization of the cell, consistent with its main function to promote esterification. Inhibition of Na+/H+ exchange in adipocytes does not prevent the changes in pHi caused by FFA, lipolytic agents, or insulin. A fatty acid dimer, which diffuses into the cell but is not metabolized, causes an irreversible acidification. Taken together, the data suggest that changes in pHi occur in adipocytes in response to the passive diffusion of un-ionized FFA (flip-flop) into and out of the cell and in response to their metabolism and production within the cell. These changes in pHi may, in turn, modulate hormonal signaling and metabolism with significant impact on cell function.
Full text
PDF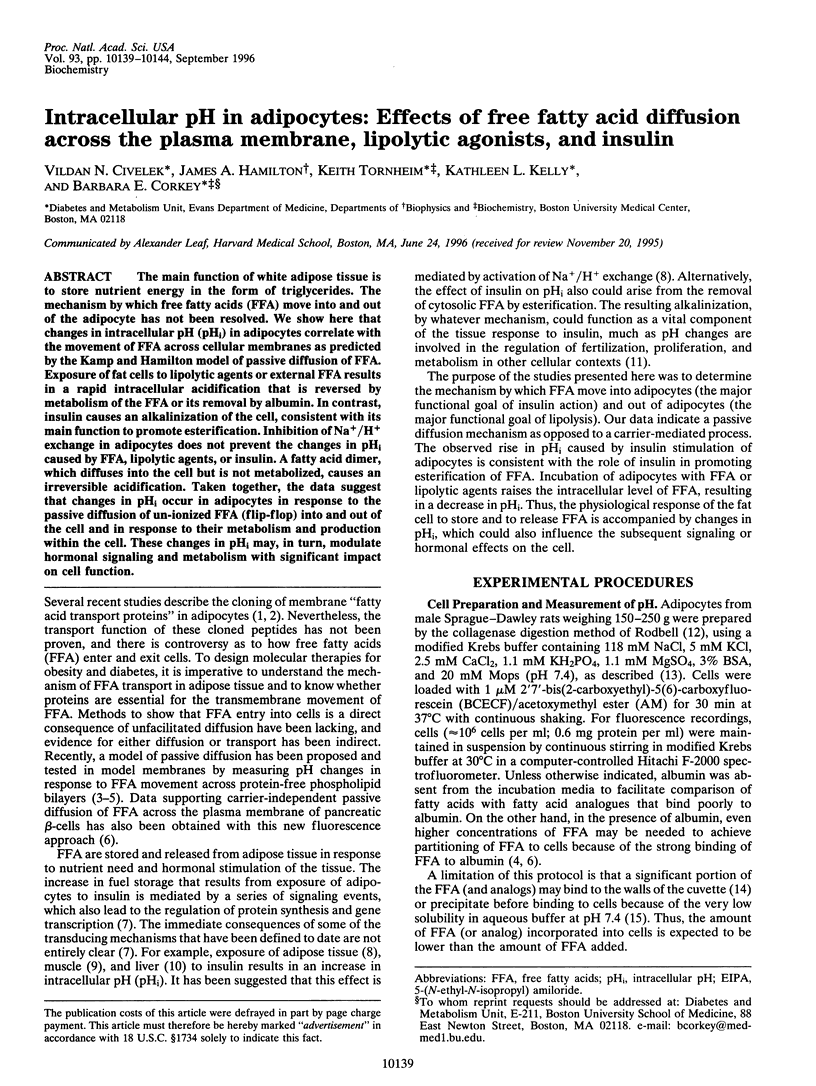
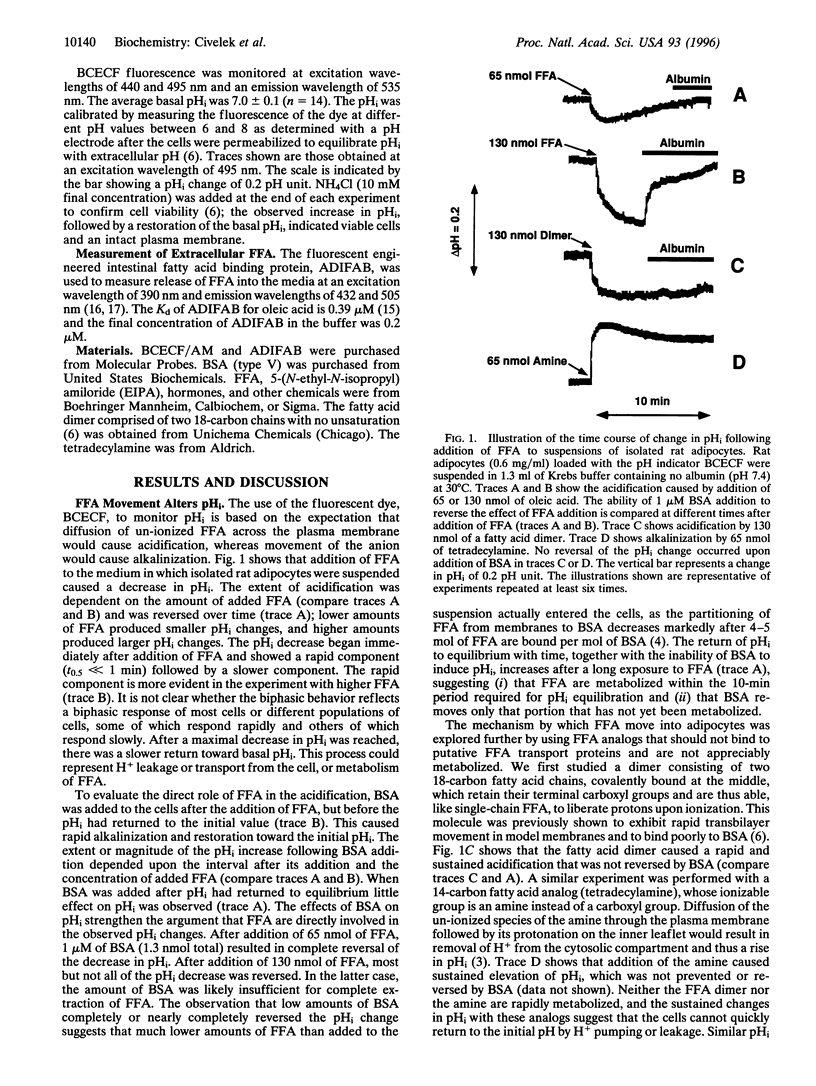
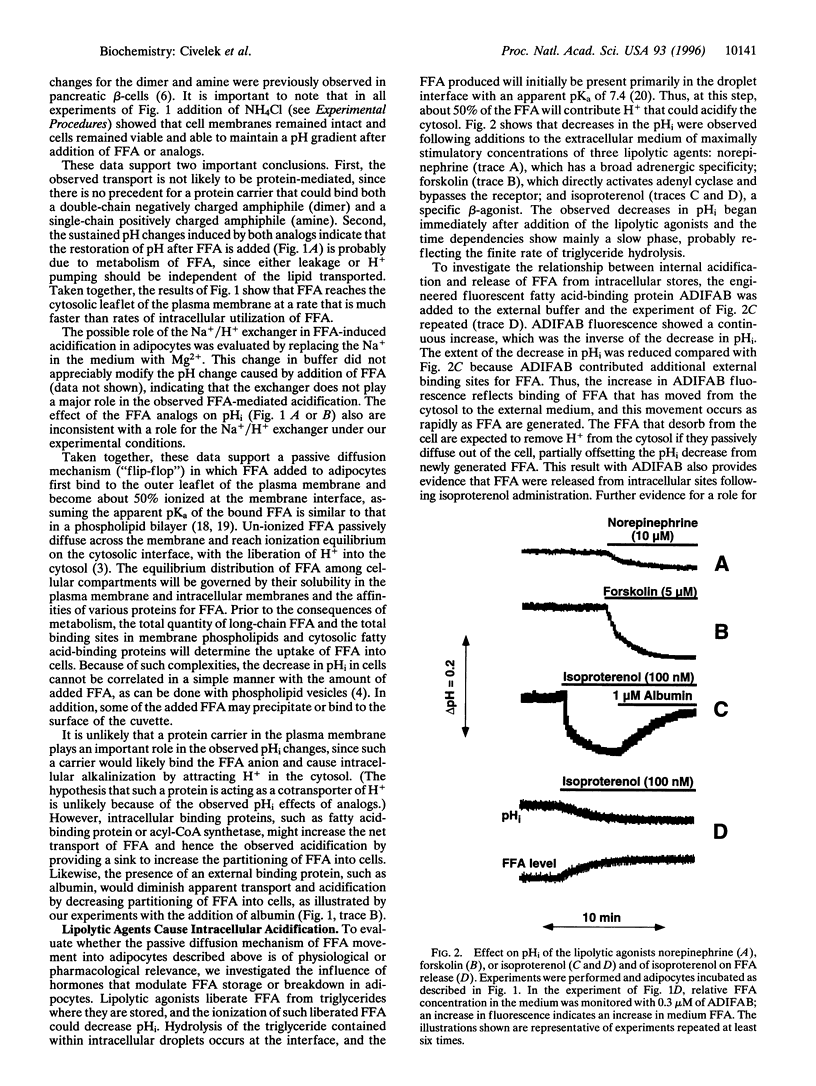
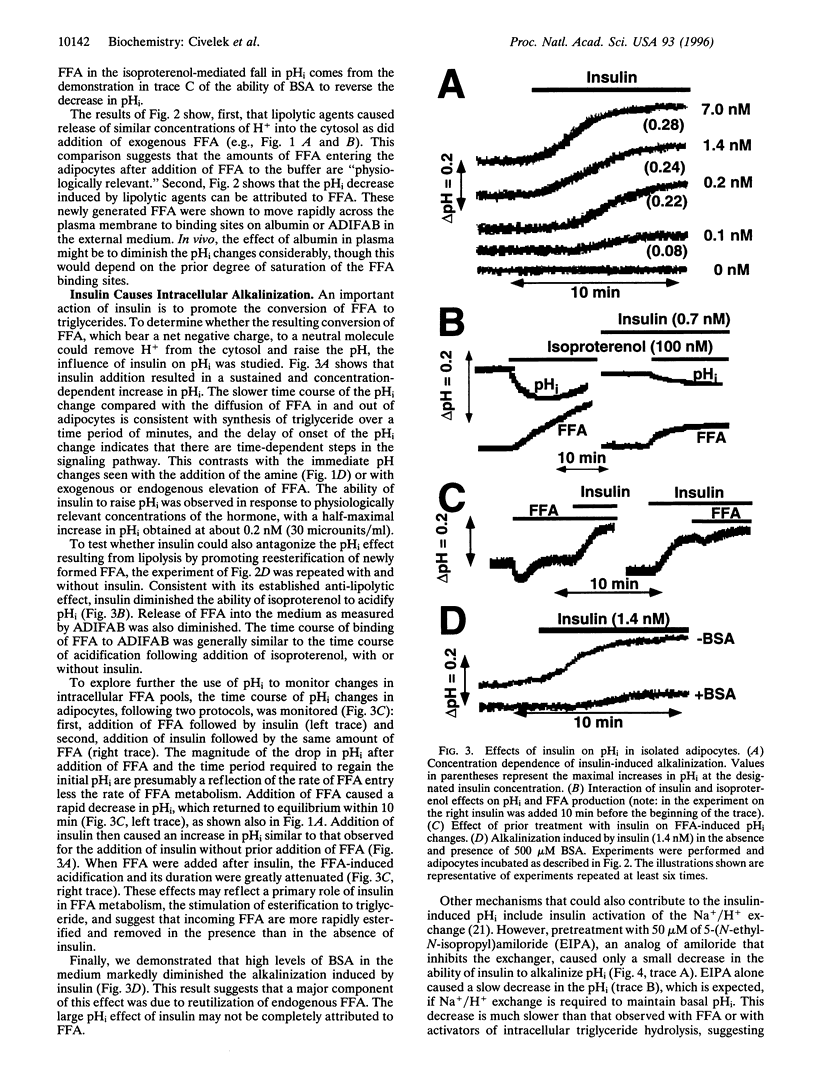
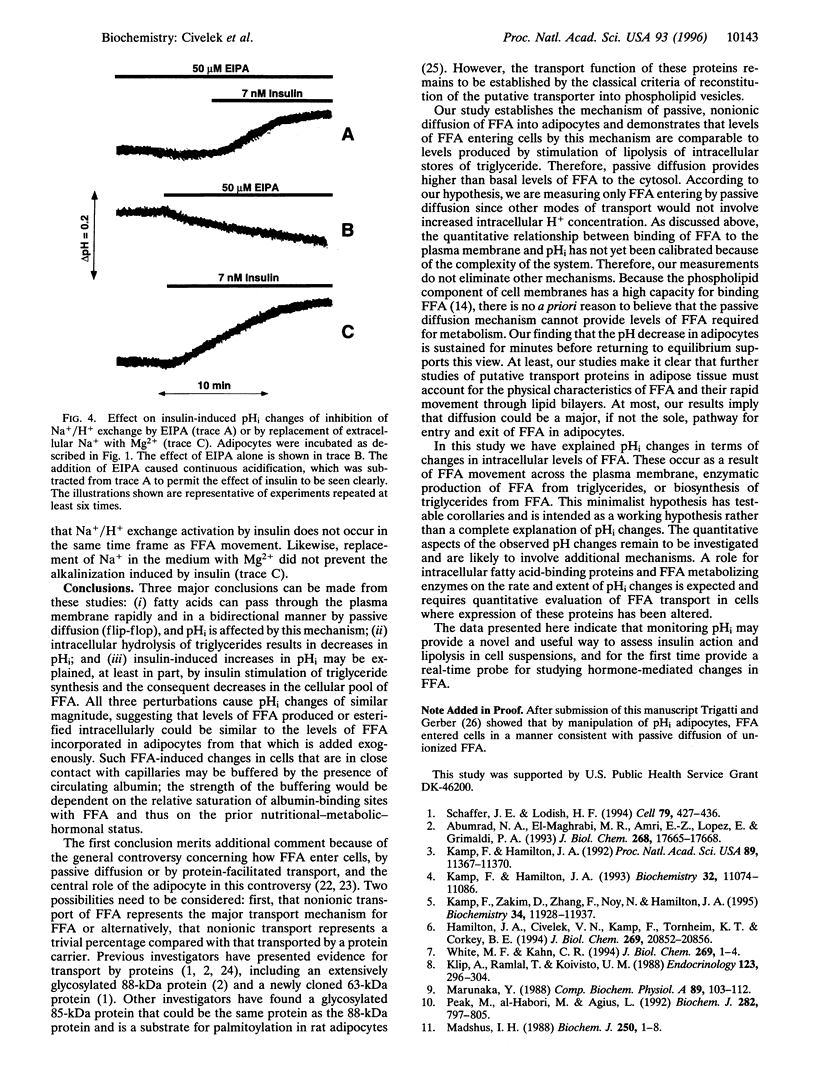
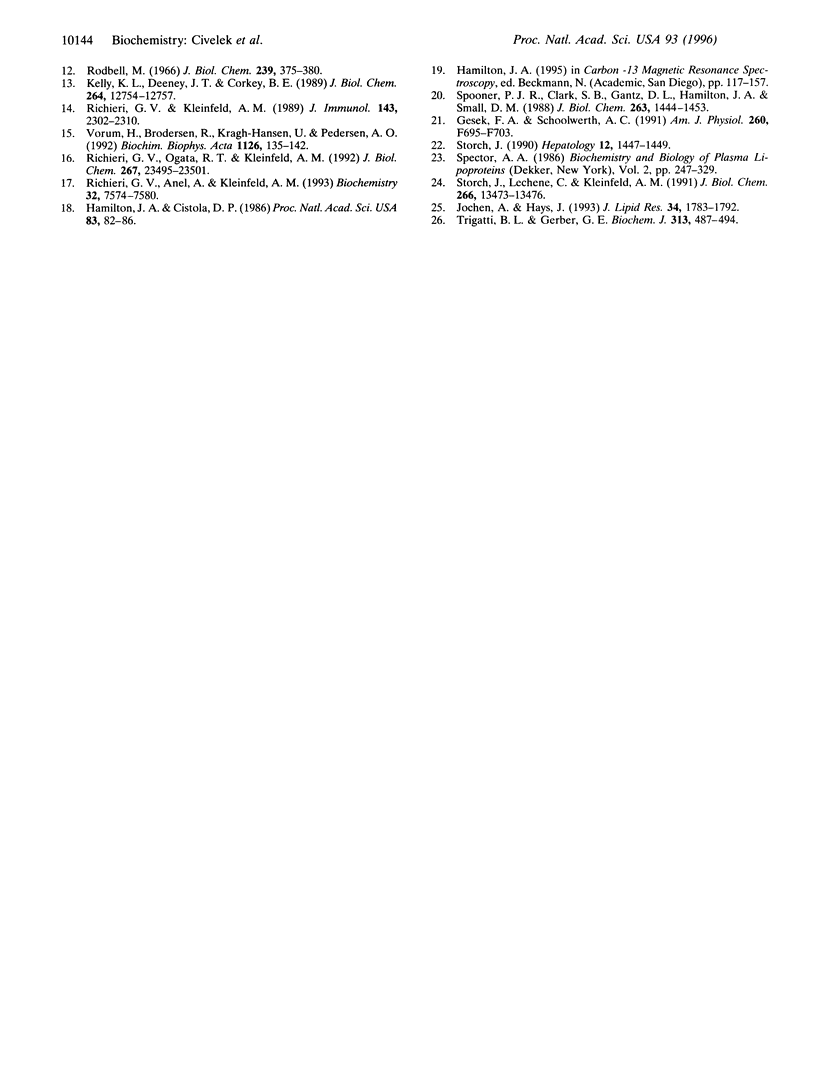
Selected References
These references are in PubMed. This may not be the complete list of references from this article.
- Abumrad N. A., el-Maghrabi M. R., Amri E. Z., Lopez E., Grimaldi P. A. Cloning of a rat adipocyte membrane protein implicated in binding or transport of long-chain fatty acids that is induced during preadipocyte differentiation. Homology with human CD36. J Biol Chem. 1993 Aug 25;268(24):17665–17668. [PubMed] [Google Scholar]
- Gesek F. A., Schoolwerth A. C. Insulin increases Na(+)-H+ exchange activity in proximal tubules from normotensive and hypertensive rats. Am J Physiol. 1991 May;260(5 Pt 2):F695–F703. doi: 10.1152/ajprenal.1991.260.5.F695. [DOI] [PubMed] [Google Scholar]
- Hamilton J. A., Cistola D. P. Transfer of oleic acid between albumin and phospholipid vesicles. Proc Natl Acad Sci U S A. 1986 Jan;83(1):82–86. doi: 10.1073/pnas.83.1.82. [DOI] [PMC free article] [PubMed] [Google Scholar]
- Hamilton J. A., Civelek V. N., Kamp F., Tornheim K., Corkey B. E. Changes in internal pH caused by movement of fatty acids into and out of clonal pancreatic beta-cells (HIT). J Biol Chem. 1994 Aug 19;269(33):20852–20856. [PubMed] [Google Scholar]
- Jochen A., Hays J. Purification of the major substrate for palmitoylation in rat adipocytes: N-terminal homology with CD36 and evidence for cell surface acylation. J Lipid Res. 1993 Oct;34(10):1783–1792. [PubMed] [Google Scholar]
- Kamp F., Hamilton J. A., Kamp F., Westerhoff H. V., Hamilton J. A. Movement of fatty acids, fatty acid analogues, and bile acids across phospholipid bilayers. Biochemistry. 1993 Oct 19;32(41):11074–11086. doi: 10.1021/bi00092a017. [DOI] [PubMed] [Google Scholar]
- Kamp F., Hamilton J. A. pH gradients across phospholipid membranes caused by fast flip-flop of un-ionized fatty acids. Proc Natl Acad Sci U S A. 1992 Dec 1;89(23):11367–11370. doi: 10.1073/pnas.89.23.11367. [DOI] [PMC free article] [PubMed] [Google Scholar]
- Kamp F., Zakim D., Zhang F., Noy N., Hamilton J. A. Fatty acid flip-flop in phospholipid bilayers is extremely fast. Biochemistry. 1995 Sep 19;34(37):11928–11937. doi: 10.1021/bi00037a034. [DOI] [PubMed] [Google Scholar]
- Kelly K. L., Deeney J. T., Corkey B. E. Cytosolic free calcium in adipocytes. Distinct mechanisms of regulation and effects on insulin action. J Biol Chem. 1989 Aug 5;264(22):12754–12757. [PubMed] [Google Scholar]
- Klip A., Ramlal T., Koivisto U. M. Stimulation of Na+/H+ exchange by insulin and phorbol ester during differentiation of 3T3-L1 cells. Relation to hexose uptake. Endocrinology. 1988 Jul;123(1):296–304. doi: 10.1210/endo-123-1-296. [DOI] [PubMed] [Google Scholar]
- Madshus I. H. Regulation of intracellular pH in eukaryotic cells. Biochem J. 1988 Feb 15;250(1):1–8. doi: 10.1042/bj2500001. [DOI] [PMC free article] [PubMed] [Google Scholar]
- Marunaka Y. Relationship between ionic surroundings and insulin actions on glucose transport and Na,K-pump in muscles. Comp Biochem Physiol A Comp Physiol. 1988;89(2):103–112. doi: 10.1016/0300-9629(88)91065-1. [DOI] [PubMed] [Google Scholar]
- Peak M., al-Habori M., Agius L. Regulation of glycogen synthesis and glycolysis by insulin, pH and cell volume. Interactions between swelling and alkalinization in mediating the effects of insulin. Biochem J. 1992 Mar 15;282(Pt 3):797–805. doi: 10.1042/bj2820797. [DOI] [PMC free article] [PubMed] [Google Scholar]
- RODBELL M. METABOLISM OF ISOLATED FAT CELLS. I. EFFECTS OF HORMONES ON GLUCOSE METABOLISM AND LIPOLYSIS. J Biol Chem. 1964 Feb;239:375–380. [PubMed] [Google Scholar]
- Richieri G. V., Anel A., Kleinfeld A. M. Interactions of long-chain fatty acids and albumin: determination of free fatty acid levels using the fluorescent probe ADIFAB. Biochemistry. 1993 Jul 27;32(29):7574–7580. doi: 10.1021/bi00080a032. [DOI] [PubMed] [Google Scholar]
- Richieri G. V., Kleinfeld A. M. Free fatty acid perturbation of transmembrane signaling in cytotoxic T lymphocytes. J Immunol. 1989 Oct 1;143(7):2302–2310. [PubMed] [Google Scholar]
- Richieri G. V., Ogata R. T., Kleinfeld A. M. A fluorescently labeled intestinal fatty acid binding protein. Interactions with fatty acids and its use in monitoring free fatty acids. J Biol Chem. 1992 Nov 25;267(33):23495–23501. [PubMed] [Google Scholar]
- Schaffer J. E., Lodish H. F. Expression cloning and characterization of a novel adipocyte long chain fatty acid transport protein. Cell. 1994 Nov 4;79(3):427–436. doi: 10.1016/0092-8674(94)90252-6. [DOI] [PubMed] [Google Scholar]
- Spooner P. J., Clark S. B., Gantz D. L., Hamilton J. A., Small D. M. The ionization and distribution behavior of oleic acid in chylomicrons and chylomicron-like emulsion particles and the influence of serum albumin. J Biol Chem. 1988 Jan 25;263(3):1444–1453. [PubMed] [Google Scholar]
- Storch J., Lechene C., Kleinfeld A. M. Direct determination of free fatty acid transport across the adipocyte plasma membrane using quantitative fluorescence microscopy. J Biol Chem. 1991 Jul 25;266(21):13473–13476. [PubMed] [Google Scholar]
- Storch J. Mechanism for binding of fatty acids to hepatocyte plasma membranes: different interpretation. Hepatology. 1990 Dec;12(6):1447–1449. doi: 10.1002/hep.1840120632. [DOI] [PubMed] [Google Scholar]
- Vorum H., Brodersen R., Kragh-Hansen U., Pedersen A. O. Solubility of long-chain fatty acids in phosphate buffer at pH 7.4. Biochim Biophys Acta. 1992 Jun 22;1126(2):135–142. doi: 10.1016/0005-2760(92)90283-2. [DOI] [PubMed] [Google Scholar]
- White M. F., Kahn C. R. The insulin signaling system. J Biol Chem. 1994 Jan 7;269(1):1–4. [PubMed] [Google Scholar]