Abstract
Natural abundance 13C spin-lattice (T1) relaxation time measurements are reported for unilamellar vesicles of 1,2-dipalmitoylphosphatidylcholine (1,2-dipalmitoyl-sn-glycero-3-phosphocholine), in the liquid crystalline phase, at magnetic field strengths of 1.40, 1.87, 2.35, 4.23, 7.05, 8.45, and 11.7 tesla (resonance frequencies of 15.0, 20.0, 25.1, 45.3, 75.5, 90.5, and 126 MHz, respectively), and the results are compared to previous 2H T1 studies of multilamellar dispersions. For both the 13C and 2H T1 studies, a dramatic frequency dependence of the relaxation was observed. At superconducting magnetic field strengths (4.23-11.7 tesla), plots of the 13C T1(-1) relaxation rates as a function of acyl chain segment position clearly reveal the characteristic "plateau" signature of the liquid crystalline phase, as found previously from 2H NMR studies. The dependence of T1(1) on ordering, determined previously from 2H NMR, and the T1(-1) dependence on frequency, determined from both 13C and 2H NMR studies, suggest that a unified picture of the bilayer molecular dynamics can be provided by a simple relaxation law of the form T1(-1) approximately equal to A tau f + BS2C-H omega -1/2(0). In the above expression, A and B are constants, SC-H (= SC-D) is the bond segmental order parameter, and omega 0 is the nuclear Larmor frequency. The first (A) term includes contributions from fast, local segmental motions characterized by the effective correlation time tau f, whereas the second (B) term describes slower, collective fluctuations in the local ordering. The value of tau f approximately equal to 10(-11) sec, obtained by extrapolating T1(-1) to infinite frequency, suggests that the segmental microviscosity of the bilayer hydrocarbon region does not differ appreciably from that of the equivalent n-paraffinic liquids of similar chain length.
Full text
PDF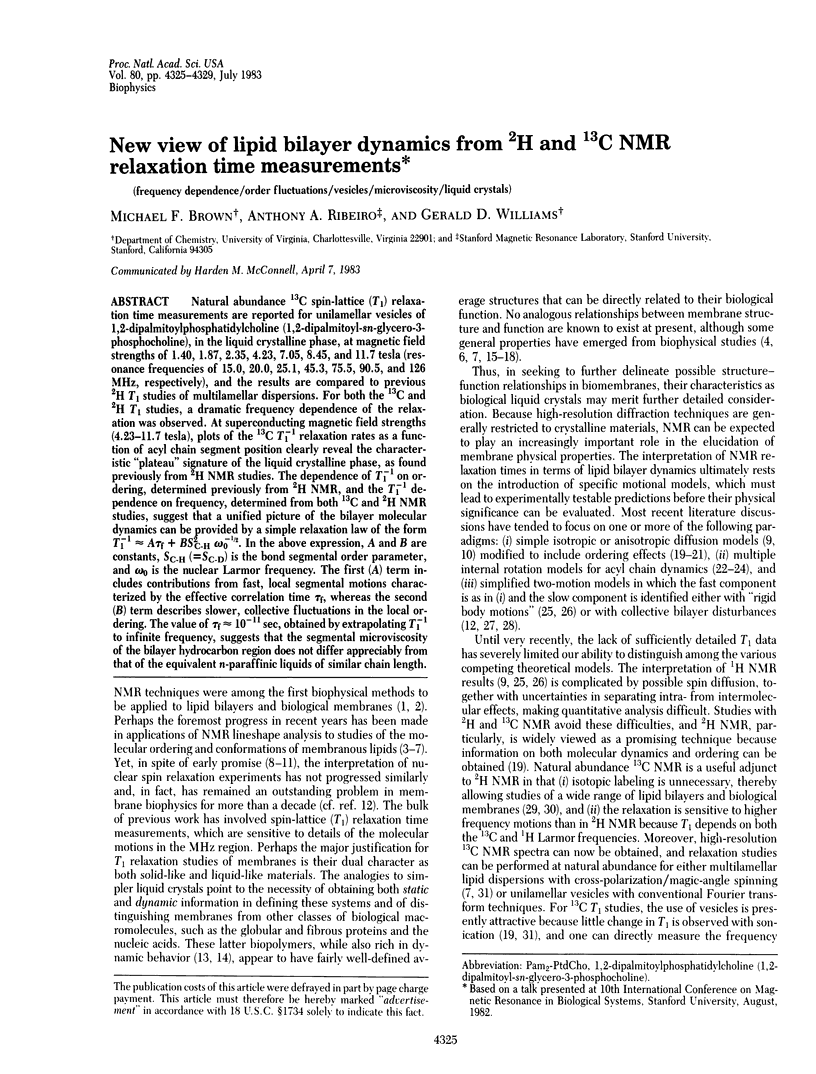
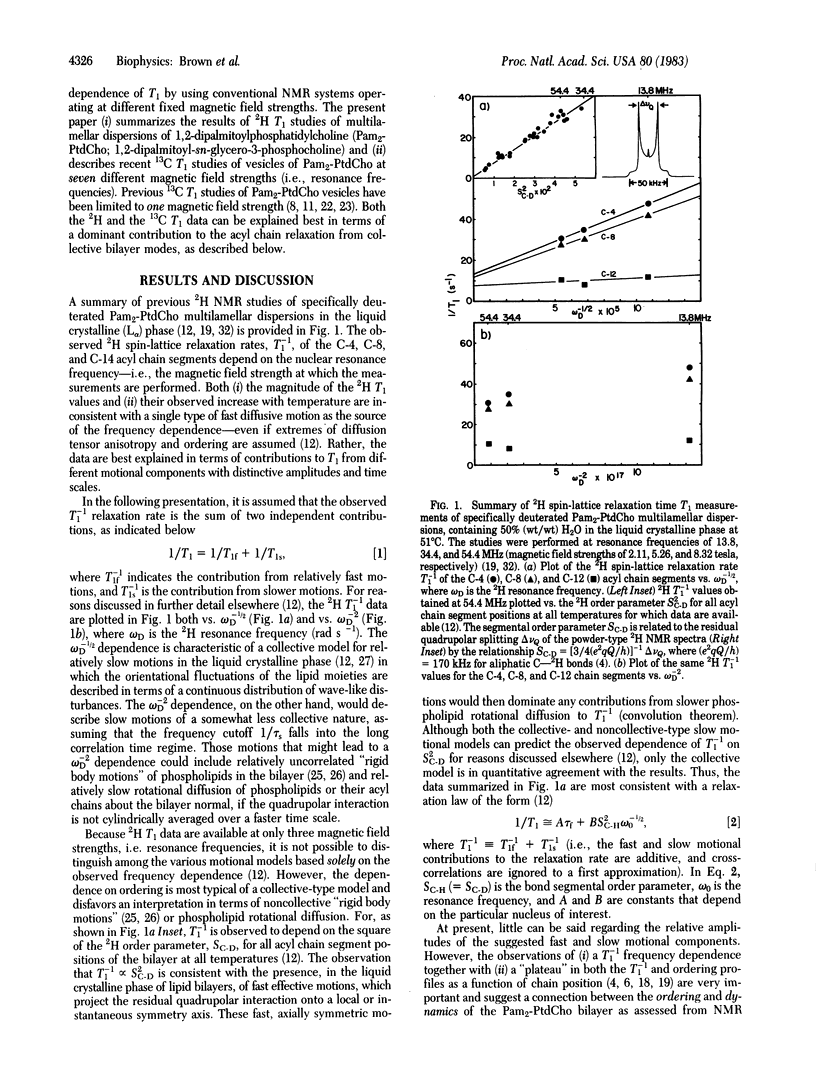
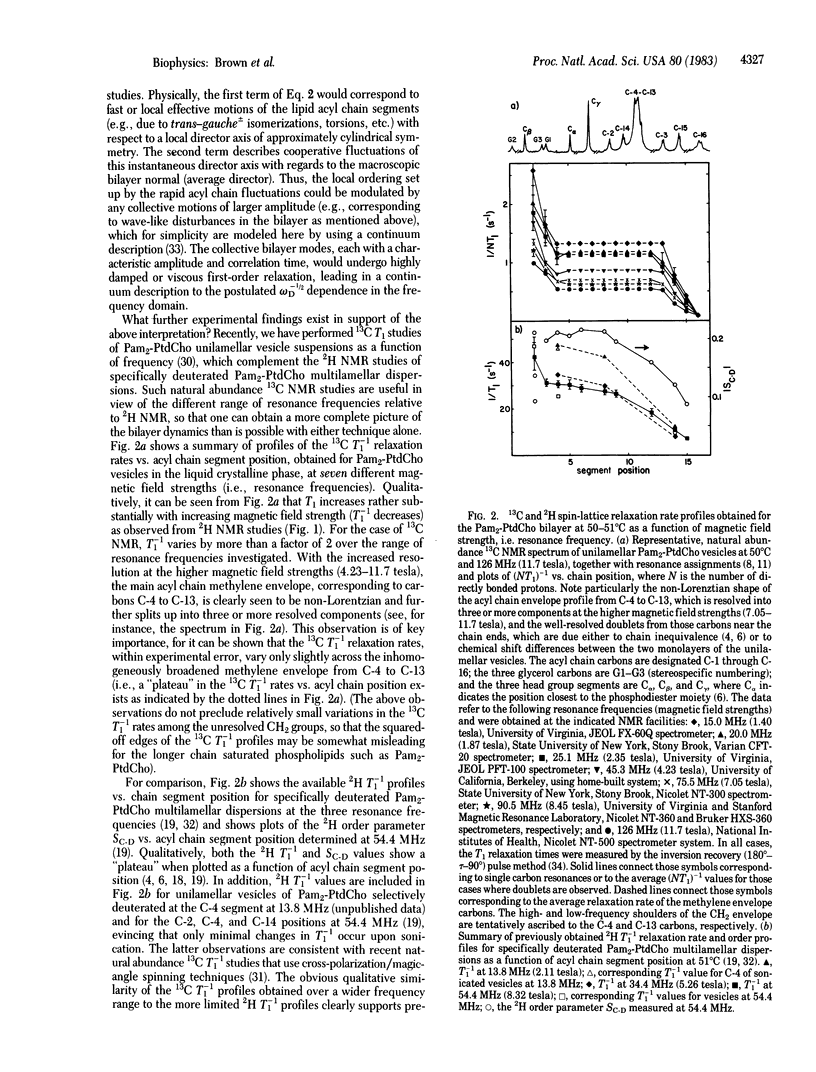
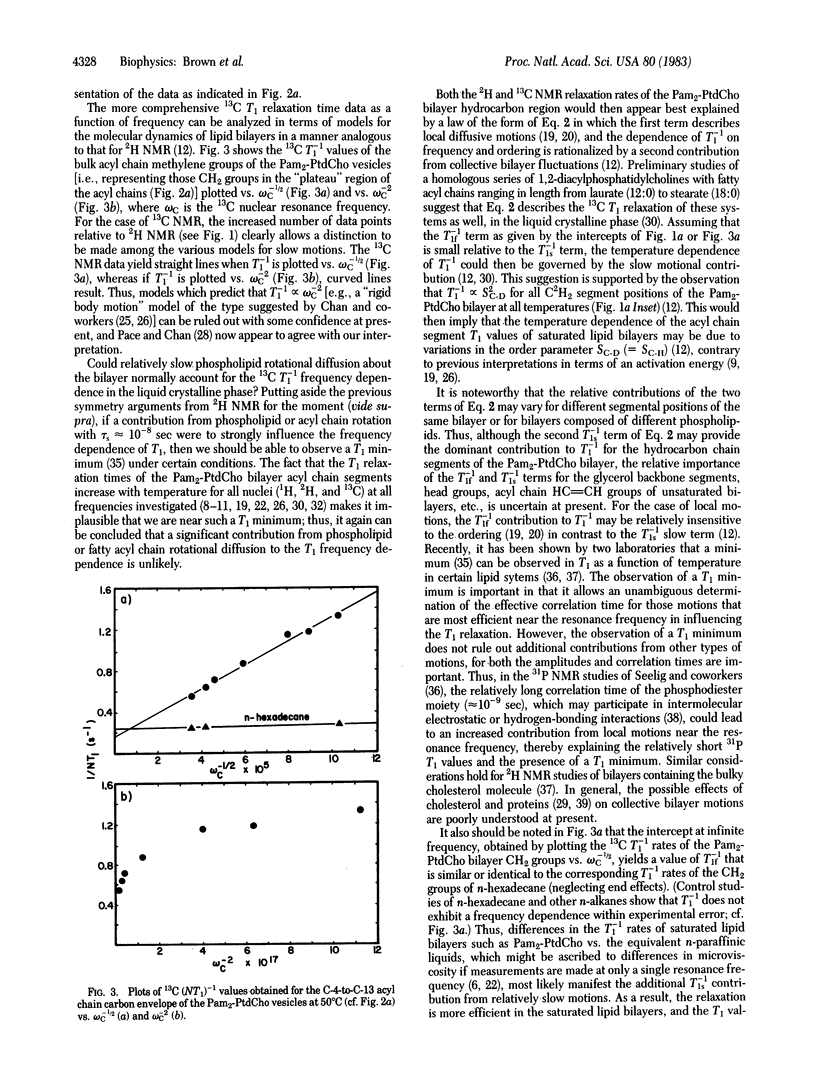
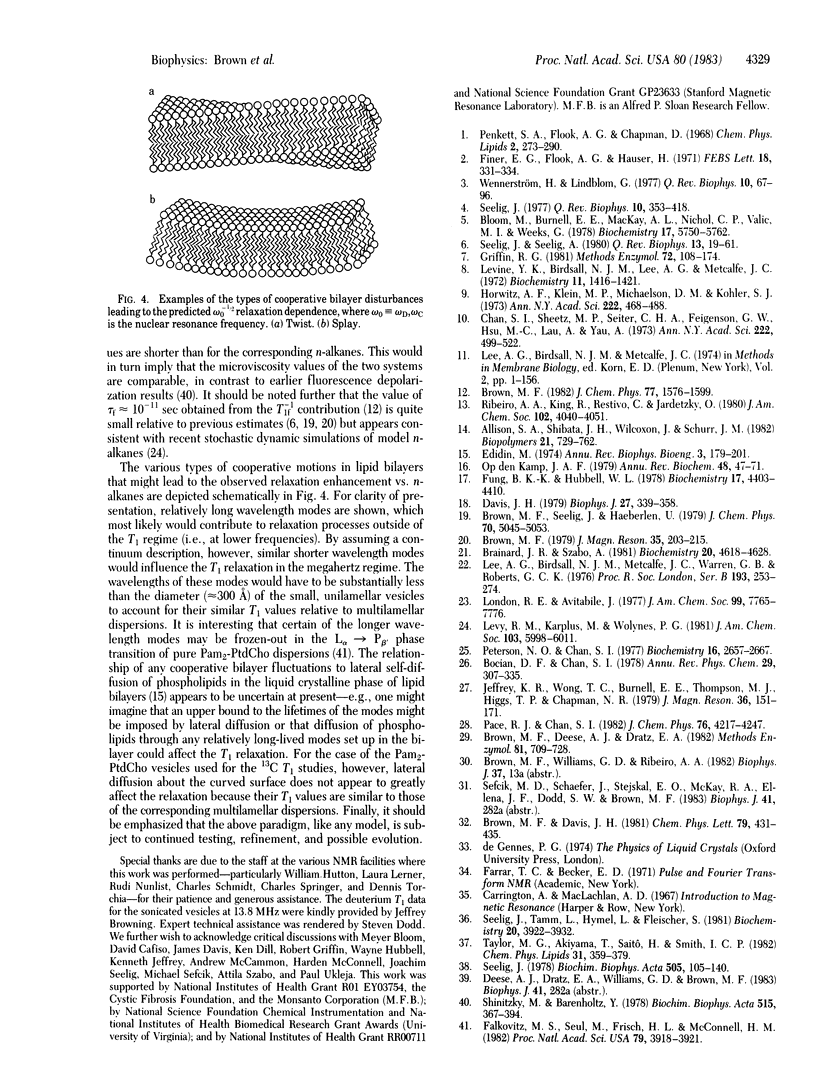
Selected References
These references are in PubMed. This may not be the complete list of references from this article.
- Allison S. A., Shibata J. H., Wilcoxon J., Schurr J. M. NMR relaxation in DNA. I. The contribution of torsional deformation modes of the elastic filament. Biopolymers. 1982 Apr;21(4):729–762. doi: 10.1002/bip.360210403. [DOI] [PubMed] [Google Scholar]
- Bloom M., Burnell E. E., MacKay A. L., Nichol C. P., Valic M. I., Weeks G. Fatty acyl chain order in lecithin model membranes determined from proton magnetic resonance. Biochemistry. 1978 Dec 26;17(26):5750–5762. doi: 10.1021/bi00619a024. [DOI] [PubMed] [Google Scholar]
- Brainard J. R., Szabo A. Theory for nuclear magnetic relaxation of probes in anisotropic systems: application of cholesterol in phospholipid vesicles. Biochemistry. 1981 Aug 4;20(16):4618–4628. doi: 10.1021/bi00519a016. [DOI] [PubMed] [Google Scholar]
- Brown M. F., Deese A. J., Dratz E. A. Proton, carbon-13, and phosphorus-31 NMR methods for the investigation of rhodopsin--lipid interactions in retinal rod outer segment membranes. Methods Enzymol. 1982;81:709–728. doi: 10.1016/s0076-6879(82)81098-7. [DOI] [PubMed] [Google Scholar]
- Chan S. I., Sheetz M. P., Seiter C. H., Feigenson G. W., Hsu M. C., Lau A., Yau A. Nuclear magnetic resonance studies of the structure of model membrane systems: the effect of surface curvature. Ann N Y Acad Sci. 1973 Dec 31;222:499–522. doi: 10.1111/j.1749-6632.1973.tb15283.x. [DOI] [PubMed] [Google Scholar]
- Davis J. H. Deuterium magnetic resonance study of the gel and liquid crystalline phases of dipalmitoyl phosphatidylcholine. Biophys J. 1979 Sep;27(3):339–358. doi: 10.1016/S0006-3495(79)85222-4. [DOI] [PMC free article] [PubMed] [Google Scholar]
- Edidin M. Rotational and translational diffusion in membranes. Annu Rev Biophys Bioeng. 1974;3(0):179–201. doi: 10.1146/annurev.bb.03.060174.001143. [DOI] [PubMed] [Google Scholar]
- Falkovitz M. S., Seul M., Frisch H. L., McConnell H. M. Theory of periodic structures in lipid bilayer membranes. Proc Natl Acad Sci U S A. 1982 Jun;79(12):3918–3921. doi: 10.1073/pnas.79.12.3918. [DOI] [PMC free article] [PubMed] [Google Scholar]
- Finer E. G., Flook A. G., Hauser H. The use of NMR spectra of sonicated phospholipid dispersions in studies of interactions with the bilayer. FEBS Lett. 1971 Nov 1;18(2):331–334. doi: 10.1016/0014-5793(71)80479-9. [DOI] [PubMed] [Google Scholar]
- Fung B. K., Hubbell W. L. Organization of rhodopsin in photoreceptor membranes. 2. Transmembrane organization of bovine rhodopsin: evidence from proteolysis and lactoperoxidase-catalyzed iodination of native and reconstituted membranes. Biochemistry. 1978 Oct 17;17(21):4403–4410. doi: 10.1021/bi00614a008. [DOI] [PubMed] [Google Scholar]
- Griffin R. G. Solid state nuclear magnetic resonance of lipid bilayers. Methods Enzymol. 1981;72:108–174. doi: 10.1016/s0076-6879(81)72010-x. [DOI] [PubMed] [Google Scholar]
- Horwitz A. F., Klein M. P., Michaelson D. M., Kohler S. J. Magnetic resonance studies of membrane and model membrane systems. V. Comparisons of aqueous dispersions of pure and mixed phospholipids. Ann N Y Acad Sci. 1973 Dec 31;222:468–488. doi: 10.1111/j.1749-6632.1973.tb15281.x. [DOI] [PubMed] [Google Scholar]
- Lee A. G., Birdsall N. J., Metcalfe J. C., Warren G. B., Roberts G. C. A determination of the mobility gradient in lipid bilayers by 13C nuclear magnetic resonance. Proc R Soc Lond B Biol Sci. 1976 May 18;193(1112):253–274. doi: 10.1098/rspb.1976.0045. [DOI] [PubMed] [Google Scholar]
- Levine Y. K., Birdsall N. J., Lee A. G., Metcalfe J. C. 13 C nuclear magnetic resonance relaxation measurements of synthetic lecithins and the effect of spin-labeled lipids. Biochemistry. 1972 Apr 11;11(8):1416–1421. doi: 10.1021/bi00758a014. [DOI] [PubMed] [Google Scholar]
- Petersen N. O., Chan S. I. More on the motional state of lipid bilayer membranes: interpretation of order parameters obtained from nuclear magnetic resonance experiments. Biochemistry. 1977 Jun 14;16(12):2657–2667. doi: 10.1021/bi00631a012. [DOI] [PubMed] [Google Scholar]
- Seelig J. 31P nuclear magnetic resonance and the head group structure of phospholipids in membranes. Biochim Biophys Acta. 1978 Jul 31;515(2):105–140. doi: 10.1016/0304-4157(78)90001-1. [DOI] [PubMed] [Google Scholar]
- Seelig J. Deuterium magnetic resonance: theory and application to lipid membranes. Q Rev Biophys. 1977 Aug;10(3):353–418. doi: 10.1017/s0033583500002948. [DOI] [PubMed] [Google Scholar]
- Seelig J., Seelig A. Lipid conformation in model membranes and biological membranes. Q Rev Biophys. 1980 Feb;13(1):19–61. doi: 10.1017/s0033583500000305. [DOI] [PubMed] [Google Scholar]
- Seelig J., Tamm L., Hymel L., Fleischer S. Deuterium and phosphorus nuclear magnetic resonance and fluorescence depolarization studies of functional reconstituted sarcoplasmic reticulum membrane vesicles. Biochemistry. 1981 Jun 23;20(13):3922–3932. doi: 10.1021/bi00516a040. [DOI] [PubMed] [Google Scholar]
- Shinitzky M., Barenholz Y. Fluidity parameters of lipid regions determined by fluorescence polarization. Biochim Biophys Acta. 1978 Dec 15;515(4):367–394. doi: 10.1016/0304-4157(78)90010-2. [DOI] [PubMed] [Google Scholar]
- Taylor M. G., Akiyama T., Saitô H., Smith I. C. Direct observation of the properties of cholesterol in membranes by deuterium NMR. Chem Phys Lipids. 1982 Dec;31(4):359–379. doi: 10.1016/0009-3084(82)90072-x. [DOI] [PubMed] [Google Scholar]
- Wennerström H., Lindblom G. Biological and model membranes studied by nuclear magnetic resonance of spin one half nuclei. Q Rev Biophys. 1977 Feb;10(1):67–96. doi: 10.1017/s0033583500000147. [DOI] [PubMed] [Google Scholar]