Abstract
Plant cells contain two major pools of K+, one in the vacuole and one in the cytosol. The behavior of K+ concentrations in these pools is fundamental to understanding the way this nutrient affects plant growth. Triple-barreled microelectrodes have been used to obtain the first fully quantitative measurements of the changes in K+ activity (aK) in the vacuole and cytosol of barley (Hordeum vulgare L.) root cells grown in different K+ concentrations. The electrodes incorporate a pH-selective barrel allowing each measurement to be assigned to either the cytosol or vacuole. The measurements revealed that vacuolar aK declined linearly with decreases in tissue K+ concentration, whereas cytosolic aK initially remained constant in both epidermal and cortical cells but then declined at different rates in each cell type. An unexpected finding was that cytoplasmic pH declined in parallel with cytosolic aK, but acidification of the cytosol with butyrate did not reveal any short-term link between these two parameters. These measurements show the very different responses of the vacuolar and cytosolic K+ pools to changes in K+ availability and also show that cytosolic K+ homeostasis differs quantitatively in different cell types. The data have been used in thermodynamic calculations to predict the need for, and likely mechanisms of, active K+ transport into the vacuole and cytosol. The direction of active K+ transport at the vacuolar membrane changes with tissue K+ status.
Full text
PDF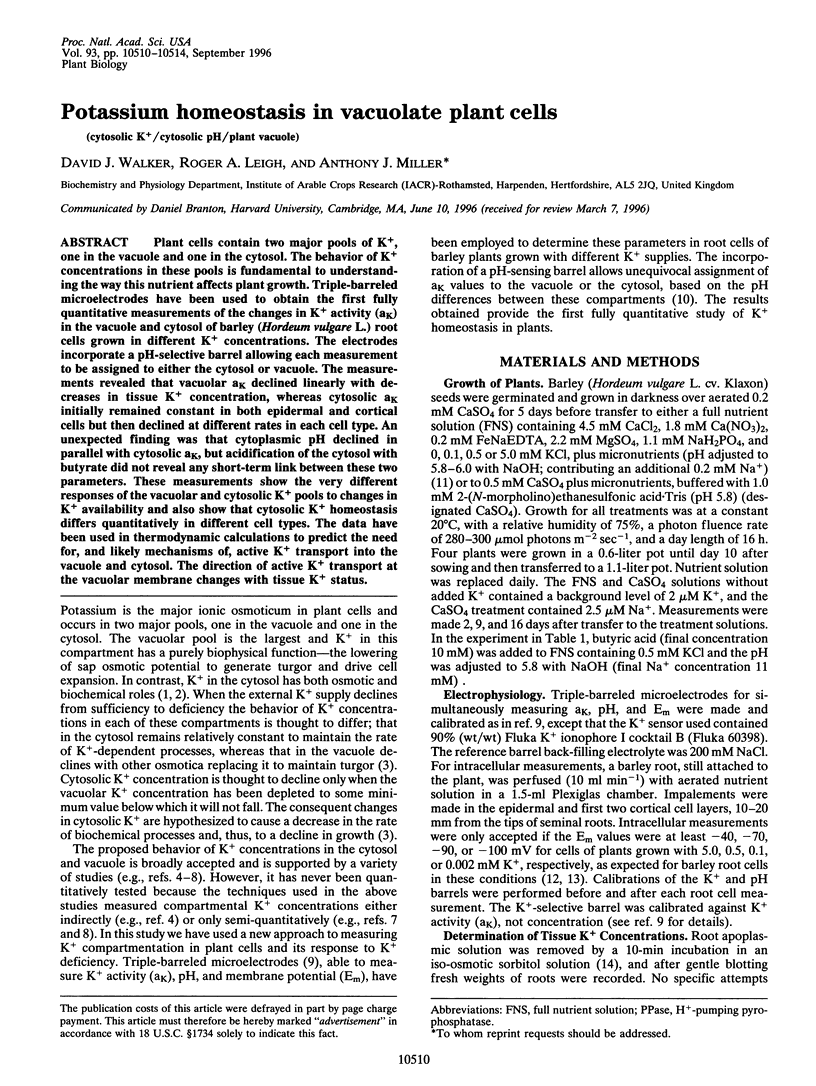
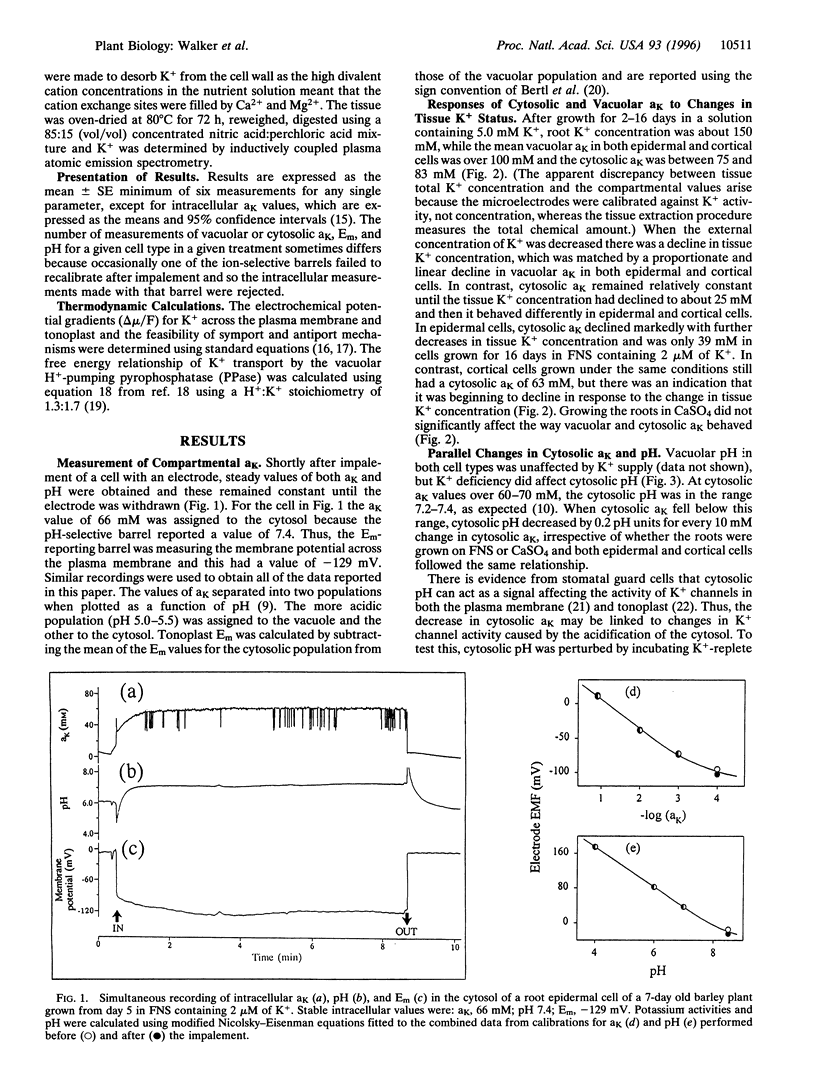
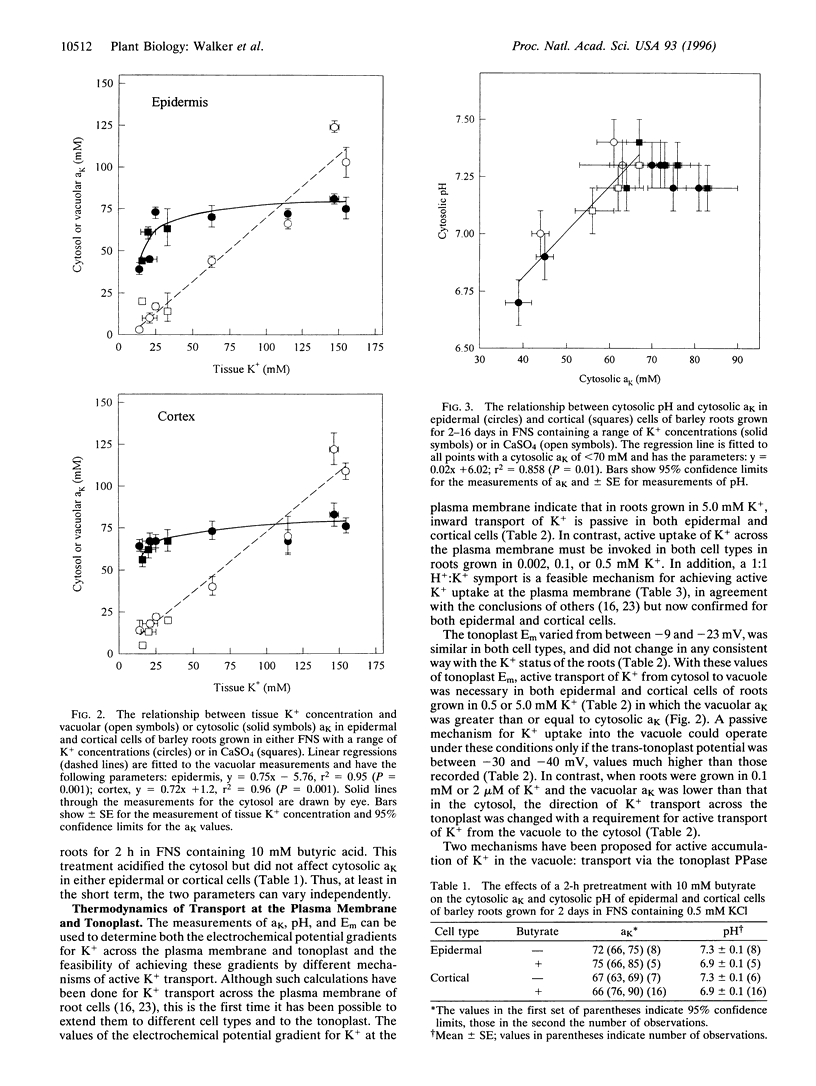
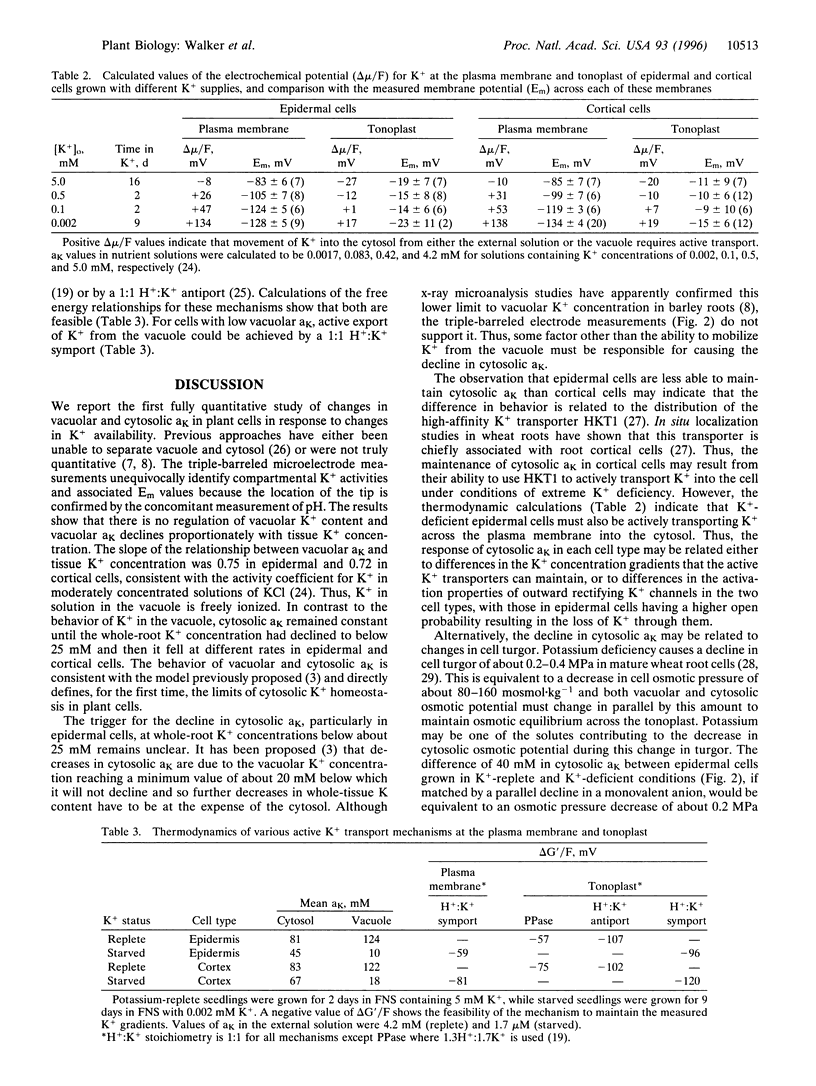
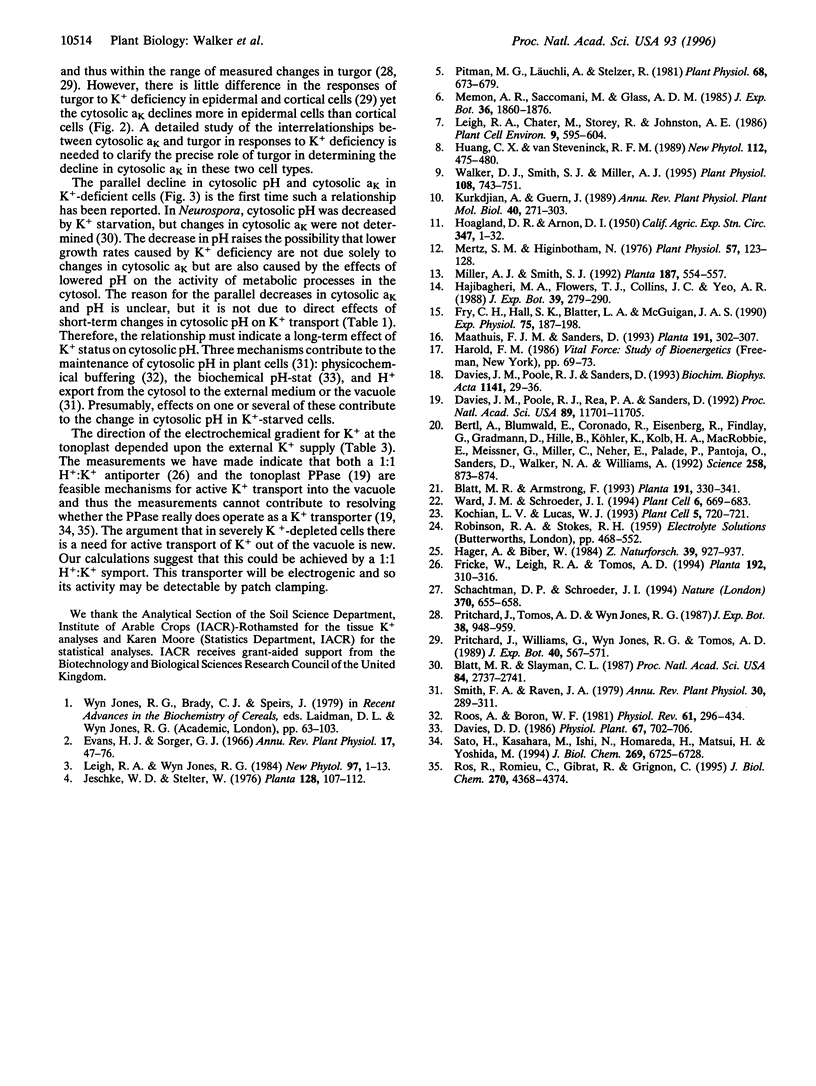
Selected References
These references are in PubMed. This may not be the complete list of references from this article.
- Bertl A., Blumwald E., Coronado R., Eisenberg R., Findlay G., Gradmann D., Hille B., Köhler K., Kolb H. A., MacRobbie E. Electrical measurements on endomembranes. Science. 1992 Nov 6;258(5084):873–874. doi: 10.1126/science.1439795. [DOI] [PubMed] [Google Scholar]
- Blatt M. R., Slayman C. L. Role of "active" potassium transport in the regulation of cytoplasmic pH by nonanimal cells. Proc Natl Acad Sci U S A. 1987 May;84(9):2737–2741. doi: 10.1073/pnas.84.9.2737. [DOI] [PMC free article] [PubMed] [Google Scholar]
- Davies J. M., Poole R. J., Rea P. A., Sanders D. Potassium transport into plant vacuoles energized directly by a proton-pumping inorganic pyrophosphatase. Proc Natl Acad Sci U S A. 1992 Dec 15;89(24):11701–11705. doi: 10.1073/pnas.89.24.11701. [DOI] [PMC free article] [PubMed] [Google Scholar]
- Fry C. H., Hall S. K., Blatter L. A., McGuigan J. A. Analysis and presentation of intracellular measurements obtained with ion-selective microelectrodes. Exp Physiol. 1990 Mar;75(2):187–198. doi: 10.1113/expphysiol.1990.sp003393. [DOI] [PubMed] [Google Scholar]
- Kochian L. V., Lucas W. J. Can K+ Channels Do It All? Plant Cell. 1993 Jul;5(7):720–721. doi: 10.1105/tpc.5.7.720. [DOI] [PMC free article] [PubMed] [Google Scholar]
- Mertz S. M., Higinbotham N. Transmembrane electropotential in barley roots as related to cell type, cell location, and cutting and aging effects. Plant Physiol. 1976 Feb;57(2):123–128. doi: 10.1104/pp.57.2.123. [DOI] [PMC free article] [PubMed] [Google Scholar]
- Pitman M. G., Läuchli A., Stelzer R. Ion distribution in roots of barley seedlings measured by electron probe x-ray microanalysis. Plant Physiol. 1981 Sep;68(3):673–679. doi: 10.1104/pp.68.3.673. [DOI] [PMC free article] [PubMed] [Google Scholar]
- Roos A., Boron W. F. Intracellular pH. Physiol Rev. 1981 Apr;61(2):296–434. doi: 10.1152/physrev.1981.61.2.296. [DOI] [PubMed] [Google Scholar]
- Ros R., Romieu C., Gibrat R., Grignon C. The plant inorganic pyrophosphatase does not transport K+ in vacuole membrane vesicles multilabeled with fluorescent probes for H+, K+, and membrane potential. J Biol Chem. 1995 Mar 3;270(9):4368–4374. doi: 10.1074/jbc.270.9.4368. [DOI] [PubMed] [Google Scholar]
- Rusthoven J. J., Davies T. A., Lerner S. A. Clinical isolation and characterization of aminoglycoside-resistant small colony variants of Enterobacter aerogenes. Am J Med. 1979 Oct;67(4):702–706. doi: 10.1016/0002-9343(79)90269-9. [DOI] [PubMed] [Google Scholar]
- Sato M. H., Kasahara M., Ishii N., Homareda H., Matsui H., Yoshida M. Purified vacuolar inorganic pyrophosphatase consisting of a 75-kDa polypeptide can pump H+ into reconstituted proteoliposomes. J Biol Chem. 1994 Mar 4;269(9):6725–6728. [PubMed] [Google Scholar]
- Schachtman D. P., Schroeder J. I. Structure and transport mechanism of a high-affinity potassium uptake transporter from higher plants. Nature. 1994 Aug 25;370(6491):655–658. doi: 10.1038/370655a0. [DOI] [PubMed] [Google Scholar]
- Walker D. J., Smith S. J., Miller A. J. Simultaneous Measurement of Intracellular pH and K+ or NO3- in Barley Root Cells Using Triple-Barreled, Ion-Selective Microelectrodes. Plant Physiol. 1995 Jun;108(2):743–751. doi: 10.1104/pp.108.2.743. [DOI] [PMC free article] [PubMed] [Google Scholar]
- Ward J. M., Schroeder J. I. Calcium-Activated K+ Channels and Calcium-Induced Calcium Release by Slow Vacuolar Ion Channels in Guard Cell Vacuoles Implicated in the Control of Stomatal Closure. Plant Cell. 1994 May;6(5):669–683. doi: 10.1105/tpc.6.5.669. [DOI] [PMC free article] [PubMed] [Google Scholar]