Abstract
A molecular model of poorly understood hydrophobic effects is heuristically developed using the methods of information theory. Because primitive hydrophobic effects can be tied to the probability of observing a molecular-sized cavity in the solvent, the probability distribution of the number of solvent centers in a cavity volume is modeled on the basis of the two moments available from the density and radial distribution of oxygen atoms in liquid water. The modeled distribution then yields the probability that no solvent centers are found in the cavity volume. This model is shown to account quantitatively for the central hydrophobic phenomena of cavity formation and association of inert gas solutes. The connection of information theory to statistical thermodynamics provides a basis for clarification of hydrophobic effects. The simplicity and flexibility of the approach suggest that it should permit applications to conformational equilibria of nonpolar solutes and hydrophobic residues in biopolymers.
Full text
PDF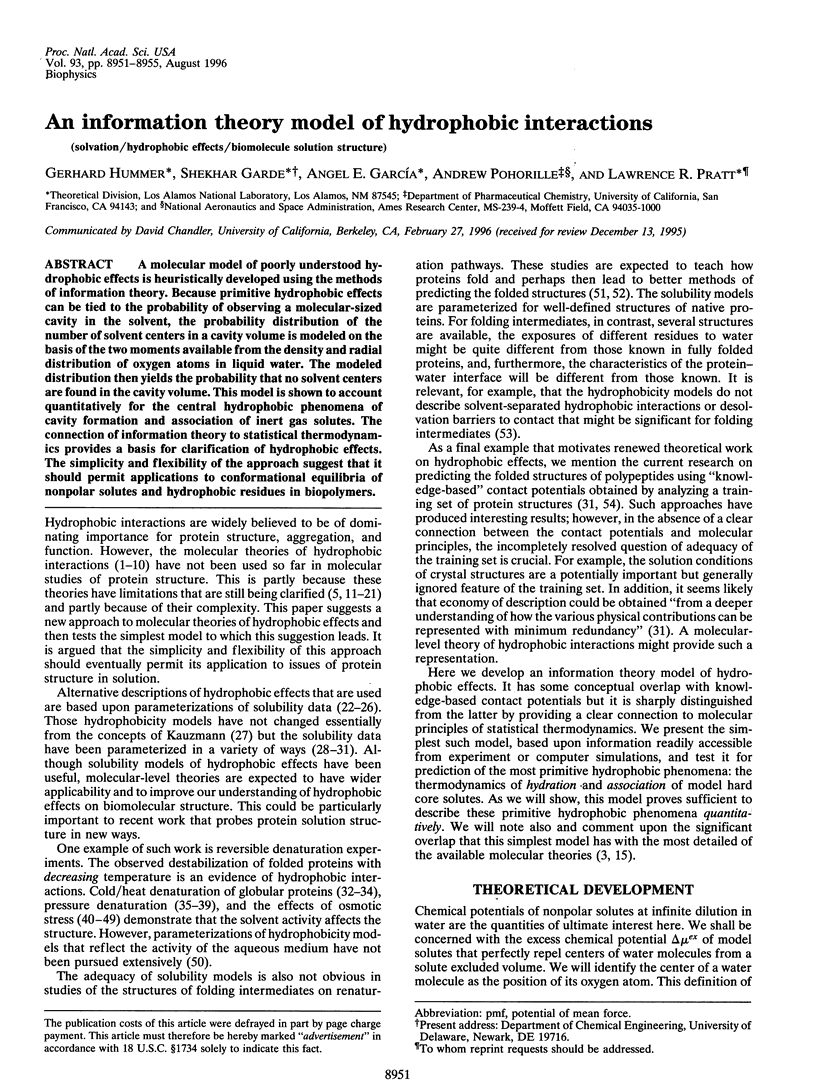
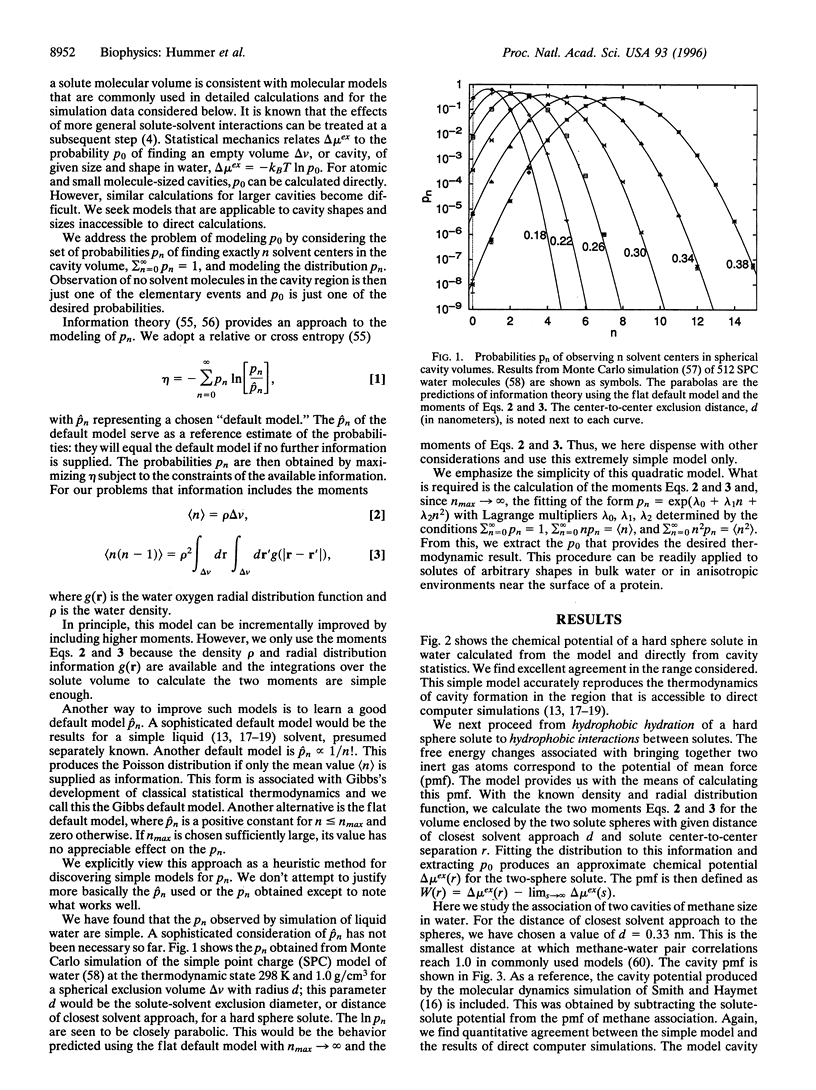
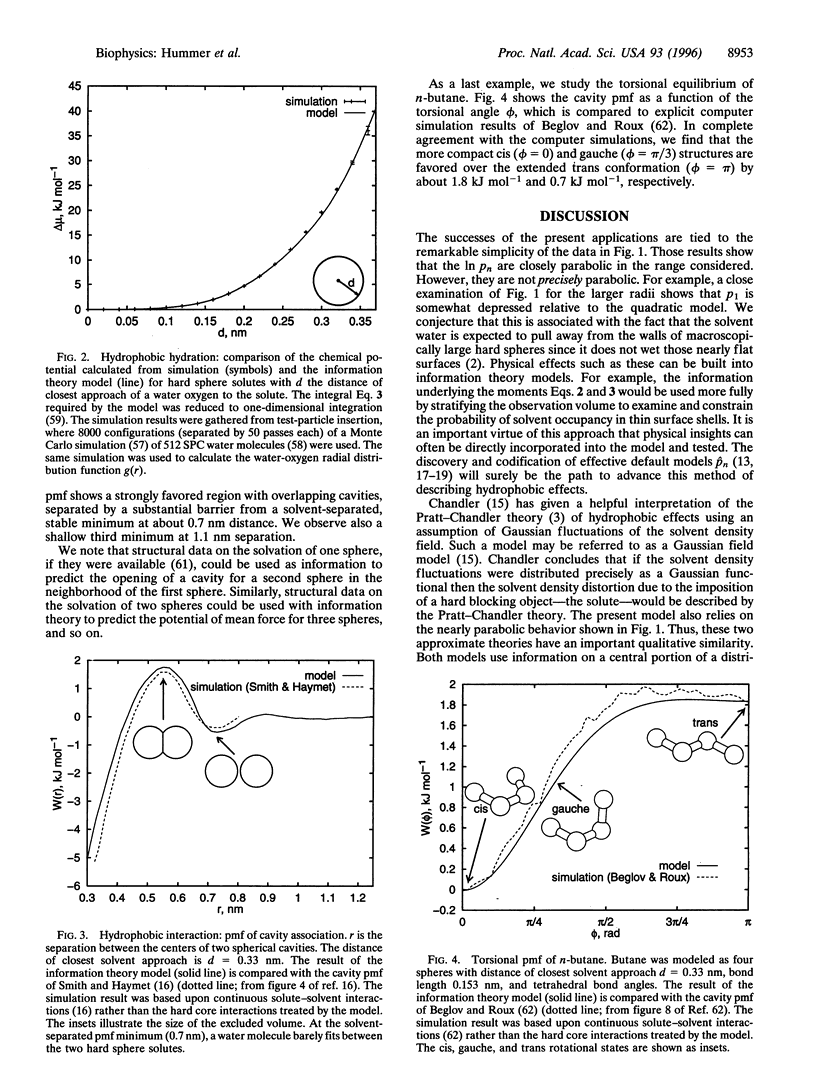
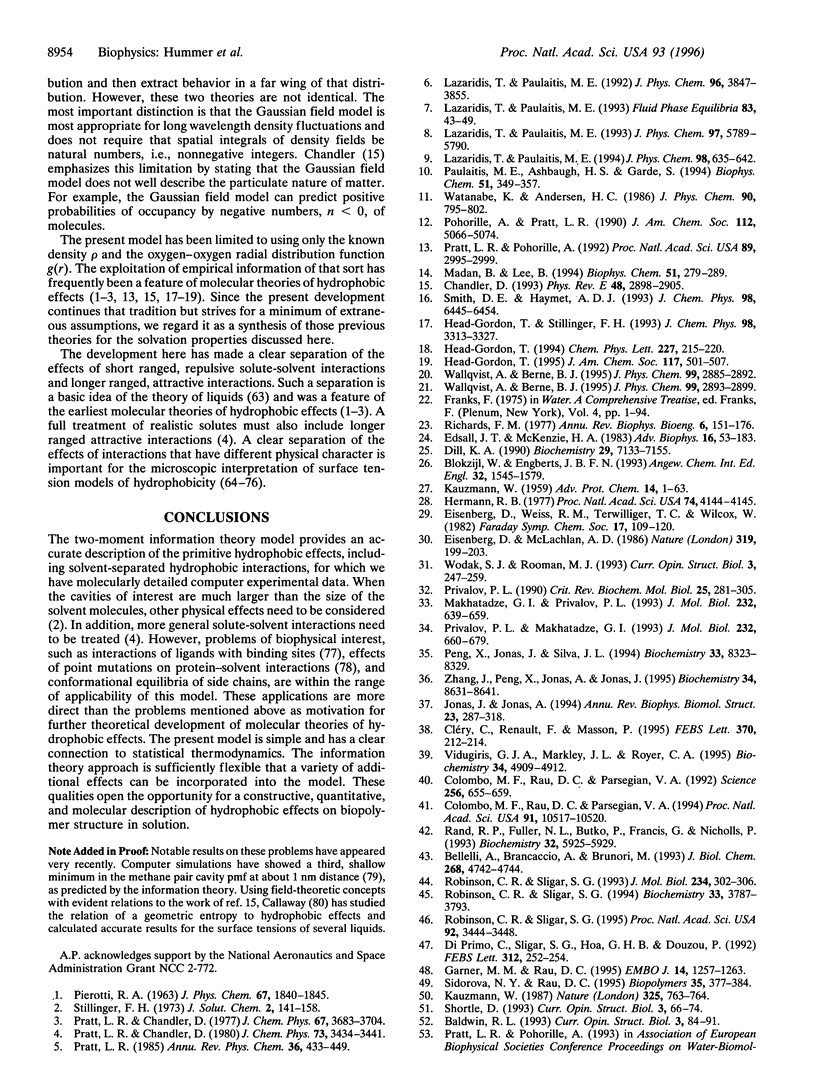
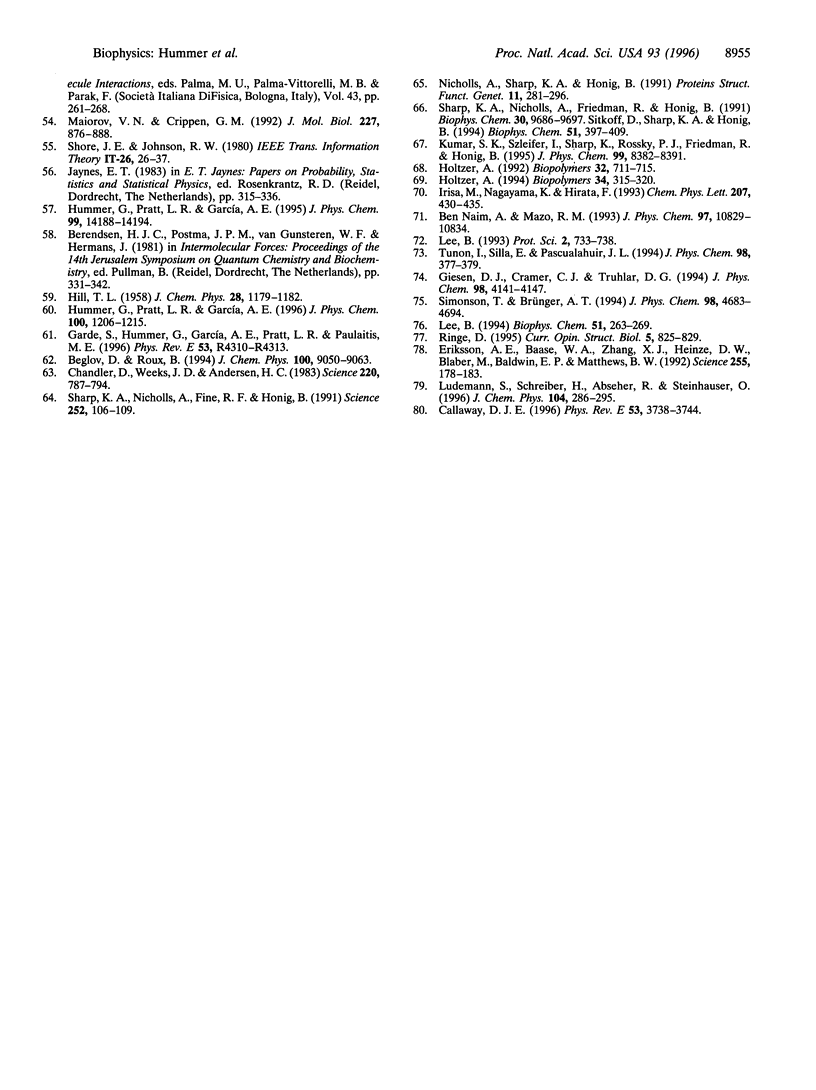
Selected References
These references are in PubMed. This may not be the complete list of references from this article.
- Bellelli A., Brancaccio A., Brunori M. Hydration and allosteric transitions in hemoglobin. J Biol Chem. 1993 Mar 5;268(7):4742–4744. [PubMed] [Google Scholar]
- Callaway DJ. Surface tension, hydrophobicity, and black holes: The entropic connection. Phys Rev E Stat Phys Plasmas Fluids Relat Interdiscip Topics. 1996 Apr;53(4):3738–3744. doi: 10.1103/physreve.53.3738. [DOI] [PubMed] [Google Scholar]
- Chandler D., Weeks J. D., Andersen H. C. Van der waals picture of liquids, solids, and phase transformations. Science. 1983 May 20;220(4599):787–794. doi: 10.1126/science.220.4599.787. [DOI] [PubMed] [Google Scholar]
- Chandler D. Gaussian field model of fluids with an application to polymeric fluids. Phys Rev E Stat Phys Plasmas Fluids Relat Interdiscip Topics. 1993 Oct;48(4):2898–2905. doi: 10.1103/physreve.48.2898. [DOI] [PubMed] [Google Scholar]
- Cléry C., Renault F., Masson P. Pressure-induced molten globule state of cholinesterase. FEBS Lett. 1995 Aug 21;370(3):212–214. doi: 10.1016/0014-5793(95)00787-a. [DOI] [PubMed] [Google Scholar]
- Colombo M. F., Rau D. C., Parsegian V. A. Protein solvation in allosteric regulation: a water effect on hemoglobin. Science. 1992 May 1;256(5057):655–659. doi: 10.1126/science.1585178. [DOI] [PubMed] [Google Scholar]
- Colombo M. F., Rau D. C., Parsegian V. A. Reevaluation of chloride's regulation of hemoglobin oxygen uptake: the neglected contribution of protein hydration in allosterism. Proc Natl Acad Sci U S A. 1994 Oct 25;91(22):10517–10520. doi: 10.1073/pnas.91.22.10517. [DOI] [PMC free article] [PubMed] [Google Scholar]
- Di Primo C., Sligar S. G., Hoa G. H., Douzou P. A critical role of protein-bound water in the catalytic cycle of cytochrome P-450 camphor. FEBS Lett. 1992 Nov 9;312(2-3):252–254. doi: 10.1016/0014-5793(92)80946-e. [DOI] [PubMed] [Google Scholar]
- Dill K. A. Dominant forces in protein folding. Biochemistry. 1990 Aug 7;29(31):7133–7155. doi: 10.1021/bi00483a001. [DOI] [PubMed] [Google Scholar]
- Edsall J. T., McKenzie H. A. Water and proteins. II. The location and dynamics of water in protein systems and its relation to their stability and properties. Adv Biophys. 1983;16:53–183. doi: 10.1016/0065-227x(83)90008-4. [DOI] [PubMed] [Google Scholar]
- Eisenberg D., McLachlan A. D. Solvation energy in protein folding and binding. Nature. 1986 Jan 16;319(6050):199–203. doi: 10.1038/319199a0. [DOI] [PubMed] [Google Scholar]
- Eriksson A. E., Baase W. A., Zhang X. J., Heinz D. W., Blaber M., Baldwin E. P., Matthews B. W. Response of a protein structure to cavity-creating mutations and its relation to the hydrophobic effect. Science. 1992 Jan 10;255(5041):178–183. doi: 10.1126/science.1553543. [DOI] [PubMed] [Google Scholar]
- Garde S, Hummer G, García AE, Pratt LR, Paulaitis ME. Hydrophobic hydration: Inhomogeneous water structure near nonpolar molecular solutes. Phys Rev E Stat Phys Plasmas Fluids Relat Interdiscip Topics. 1996 May;53(5):R4310–R4313. doi: 10.1103/physreve.53.r4310. [DOI] [PubMed] [Google Scholar]
- Garner M. M., Rau D. C. Water release associated with specific binding of gal repressor. EMBO J. 1995 Mar 15;14(6):1257–1263. doi: 10.1002/j.1460-2075.1995.tb07109.x. [DOI] [PMC free article] [PubMed] [Google Scholar]
- Hermann R. B. Use of solvent cavity area and number of packed solvent molecules around a solute in regard to hydrocarbon solubilities and hydrophobic interactions. Proc Natl Acad Sci U S A. 1977 Oct;74(10):4144–4145. doi: 10.1073/pnas.74.10.4144. [DOI] [PMC free article] [PubMed] [Google Scholar]
- Holtzer A. Does Flory-Huggins theory help in interpreting solute partitioning experiments? Biopolymers. 1994 Mar;34(3):315–320. doi: 10.1002/bip.360340303. [DOI] [PubMed] [Google Scholar]
- Holtzer A. The use of Flory-Huggins theory in interpreting partitioning of solutes between organic liquids and water. Biopolymers. 1992 Jun;32(6):711–715. doi: 10.1002/bip.360320611. [DOI] [PubMed] [Google Scholar]
- Jonas J., Jonas A. High-pressure NMR spectroscopy of proteins and membranes. Annu Rev Biophys Biomol Struct. 1994;23:287–318. doi: 10.1146/annurev.bb.23.060194.001443. [DOI] [PubMed] [Google Scholar]
- KAUZMANN W. Some factors in the interpretation of protein denaturation. Adv Protein Chem. 1959;14:1–63. doi: 10.1016/s0065-3233(08)60608-7. [DOI] [PubMed] [Google Scholar]
- Lee B. Relation between volume correction and the standard state. Biophys Chem. 1994 Aug;51(2-3):263–269. doi: 10.1016/0301-4622(94)00047-6. [DOI] [PubMed] [Google Scholar]
- Madan B., Lee B. Role of hydrogen bonds in hydrophobicity: the free energy of cavity formation in water models with and without the hydrogen bonds. Biophys Chem. 1994 Aug;51(2-3):279–289. doi: 10.1016/0301-4622(94)00049-2. [DOI] [PubMed] [Google Scholar]
- Maiorov V. N., Crippen G. M. Contact potential that recognizes the correct folding of globular proteins. J Mol Biol. 1992 Oct 5;227(3):876–888. doi: 10.1016/0022-2836(92)90228-c. [DOI] [PubMed] [Google Scholar]
- Makhatadze G. I., Privalov P. L. Contribution of hydration to protein folding thermodynamics. I. The enthalpy of hydration. J Mol Biol. 1993 Jul 20;232(2):639–659. doi: 10.1006/jmbi.1993.1416. [DOI] [PubMed] [Google Scholar]
- Nicholls A., Sharp K. A., Honig B. Protein folding and association: insights from the interfacial and thermodynamic properties of hydrocarbons. Proteins. 1991;11(4):281–296. doi: 10.1002/prot.340110407. [DOI] [PubMed] [Google Scholar]
- Peng X., Jonas J., Silva J. L. High-pressure NMR study of the dissociation of Arc repressor. Biochemistry. 1994 Jul 12;33(27):8323–8329. doi: 10.1021/bi00193a020. [DOI] [PubMed] [Google Scholar]
- Pohorille A., Pratt L. R. Cavities in molecular liquids and the theory of hydrophobic solubilities. J Am Chem Soc. 1990;112(13):5066–5074. doi: 10.1021/ja00169a011. [DOI] [PubMed] [Google Scholar]
- Pratt L. R., Pohorille A. Theory of hydrophobicity: transient cavities in molecular liquids. Proc Natl Acad Sci U S A. 1992 Apr;89:2995–2999. doi: 10.1073/pnas.89.7.2995. [DOI] [PMC free article] [PubMed] [Google Scholar]
- Privalov P. L. Cold denaturation of proteins. Crit Rev Biochem Mol Biol. 1990;25(4):281–305. doi: 10.3109/10409239009090612. [DOI] [PubMed] [Google Scholar]
- Privalov P. L., Makhatadze G. I. Contribution of hydration to protein folding thermodynamics. II. The entropy and Gibbs energy of hydration. J Mol Biol. 1993 Jul 20;232(2):660–679. doi: 10.1006/jmbi.1993.1417. [DOI] [PubMed] [Google Scholar]
- Rand R. P., Fuller N. L., Butko P., Francis G., Nicholls P. Measured change in protein solvation with substrate binding and turnover. Biochemistry. 1993 Jun 15;32(23):5925–5929. doi: 10.1021/bi00074a001. [DOI] [PubMed] [Google Scholar]
- Richards F. M. Areas, volumes, packing and protein structure. Annu Rev Biophys Bioeng. 1977;6:151–176. doi: 10.1146/annurev.bb.06.060177.001055. [DOI] [PubMed] [Google Scholar]
- Ringe D. What makes a binding site a binding site? Curr Opin Struct Biol. 1995 Dec;5(6):825–829. doi: 10.1016/0959-440x(95)80017-4. [DOI] [PubMed] [Google Scholar]
- Robinson C. R., Sligar S. G. Heterogeneity in molecular recognition by restriction endonucleases: osmotic and hydrostatic pressure effects on BamHI, Pvu II, and EcoRV specificity. Proc Natl Acad Sci U S A. 1995 Apr 11;92(8):3444–3448. doi: 10.1073/pnas.92.8.3444. [DOI] [PMC free article] [PubMed] [Google Scholar]
- Robinson C. R., Sligar S. G. Hydrostatic pressure reverses osmotic pressure effects on the specificity of EcoRI-DNA interactions. Biochemistry. 1994 Apr 5;33(13):3787–3793. doi: 10.1021/bi00179a001. [DOI] [PubMed] [Google Scholar]
- Robinson C. R., Sligar S. G. Molecular recognition mediated by bound water. A mechanism for star activity of the restriction endonuclease EcoRI. J Mol Biol. 1993 Nov 20;234(2):302–306. doi: 10.1006/jmbi.1993.1586. [DOI] [PubMed] [Google Scholar]
- Sharp K. A., Nicholls A., Fine R. F., Honig B. Reconciling the magnitude of the microscopic and macroscopic hydrophobic effects. Science. 1991 Apr 5;252(5002):106–109. doi: 10.1126/science.2011744. [DOI] [PubMed] [Google Scholar]
- Sharp K. A., Nicholls A., Friedman R., Honig B. Extracting hydrophobic free energies from experimental data: relationship to protein folding and theoretical models. Biochemistry. 1991 Oct 8;30(40):9686–9697. doi: 10.1021/bi00104a017. [DOI] [PubMed] [Google Scholar]
- Sidorova NYu, Rau D. C. The osmotic sensitivity of netropsin analogue binding to DNA. Biopolymers. 1995 Apr;35(4):377–384. doi: 10.1002/bip.360350405. [DOI] [PubMed] [Google Scholar]
- Vidugiris G. J., Markley J. L., Royer C. A. Evidence for a molten globule-like transition state in protein folding from determination of activation volumes. Biochemistry. 1995 Apr 18;34(15):4909–4912. doi: 10.1021/bi00015a001. [DOI] [PubMed] [Google Scholar]
- Zhang J., Peng X., Jonas A., Jonas J. NMR study of the cold, heat, and pressure unfolding of ribonuclease A. Biochemistry. 1995 Jul 11;34(27):8631–8641. doi: 10.1021/bi00027a012. [DOI] [PubMed] [Google Scholar]