Abstract
Despite the biological and medical importance of signal transduction via Ras proteins and despite considerable kinetic and structural studies of wild-type and mutant Ras proteins, the mechanism of Ras-catalyzed GTP hydrolysis remains controversial. We take a different approach to this problem: the uncatalyzed hydrolysis of GTP is analyzed, and the understanding derived is applied to the Ras-catalyzed reaction. Evaluation of previous mechanistic proposals from this chemical perspective suggests that proton abstraction from the attacking water by a general base and stabilization of charge development on the gamma-phosphoryl oxygen atoms would not be catalytic. Rather, this analysis focuses attention on the GDP leaving group, including the beta-gamma bridge oxygen of GTP, the atom that undergoes the largest change in charge in going from the ground state to the transition state. This leads to a new catalytic proposal in which a hydrogen bond from the backbone amide of Gly-13 to this bridge oxygen is strengthened in the transition state relative to the ground state, within an active site that provides a template complementary to the transition state. Strengthened transition state interactions of the active site lysine, Lys-16, with the beta-nonbridging phosphoryl oxygens and a network of interactions that positions the nucleophilic water molecule and gamma-phosphoryl group with respect to one another may also contribute to catalysis. It is speculated that a significant fraction of the GAP-activated GTPase activity of Ras arises from an additional interaction of the beta-gamma bridge oxygen with an Arg side chain that is provided in trans by GAP. The conclusions for Ras and related G proteins are expected to apply more widely to other enzymes that catalyze phosphoryl (-PO(3)2-) transfer, including kinases and phosphatases.
Full text
PDF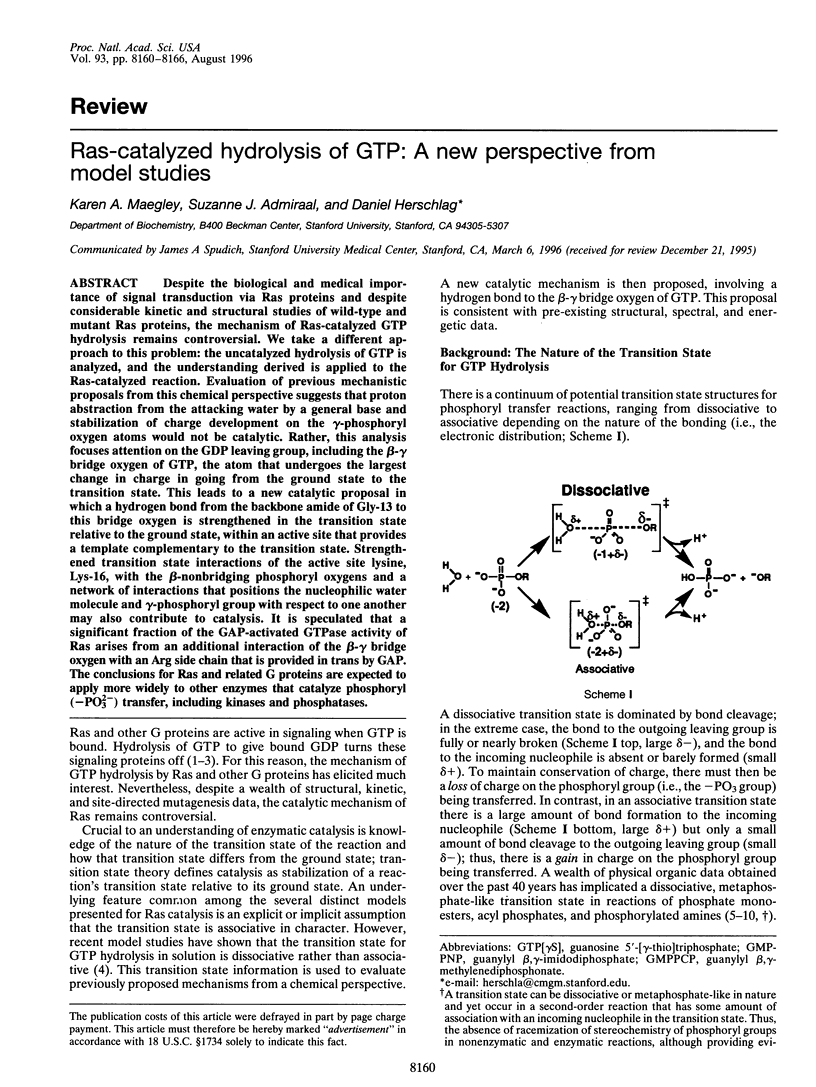
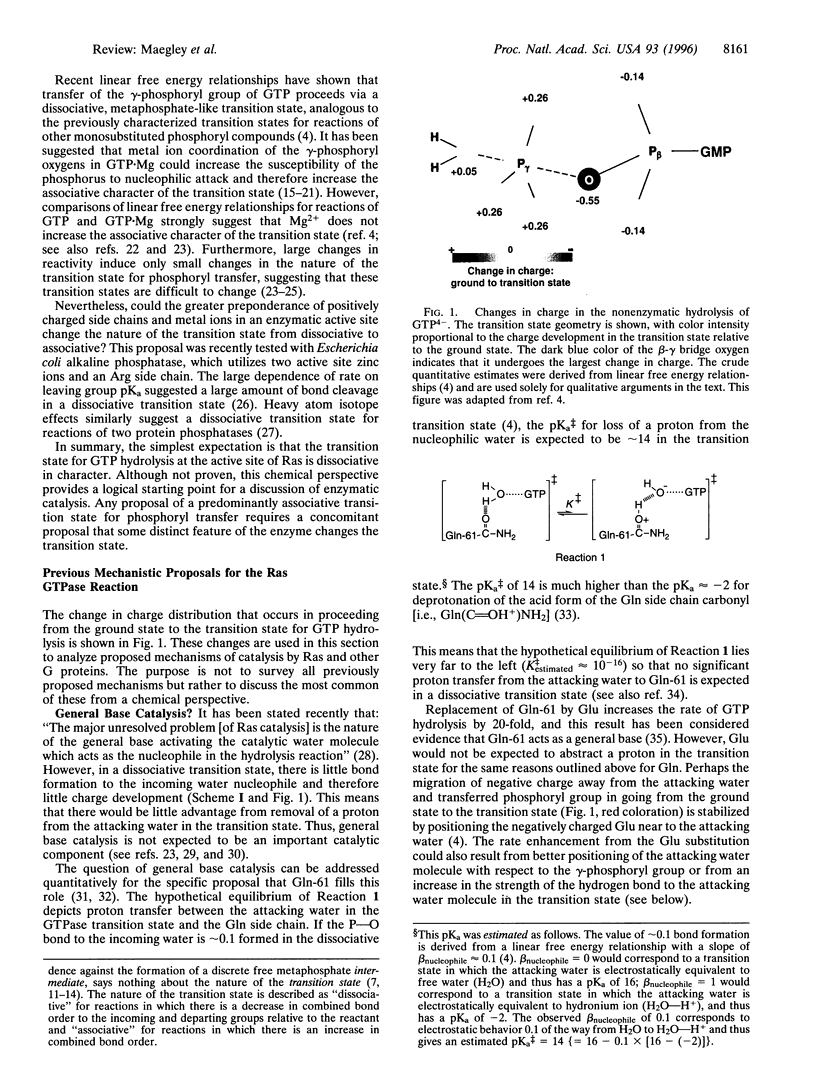
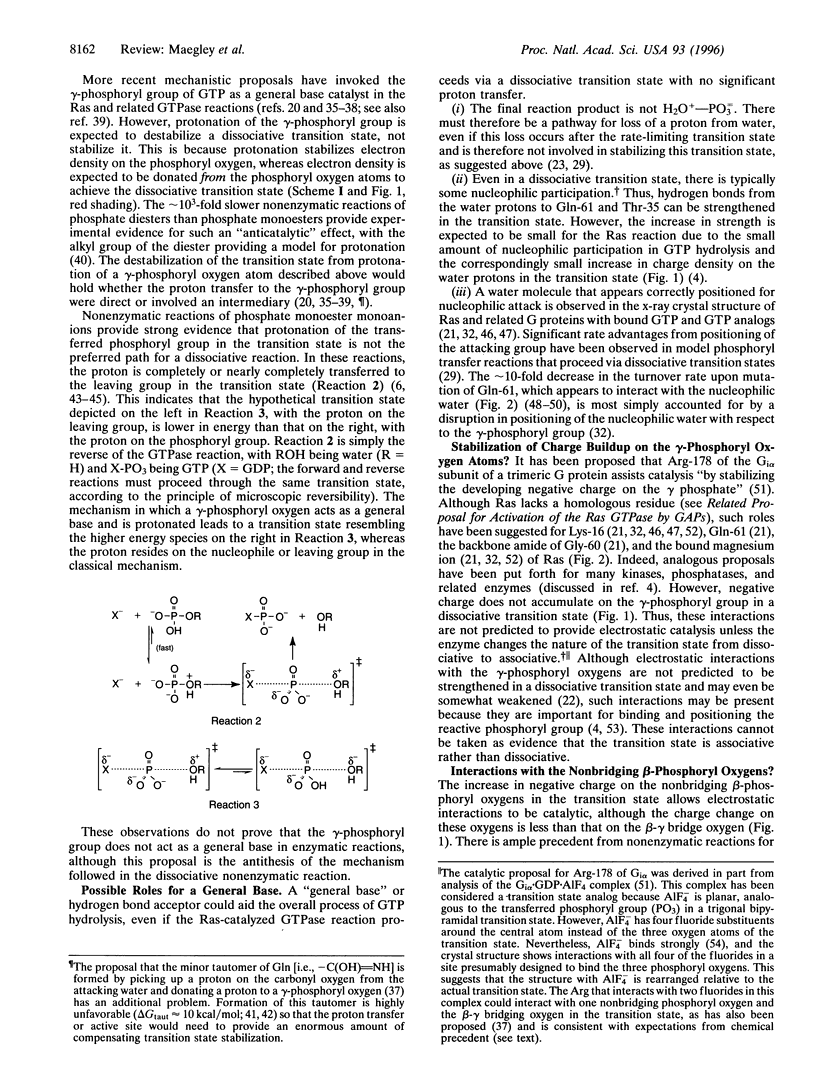
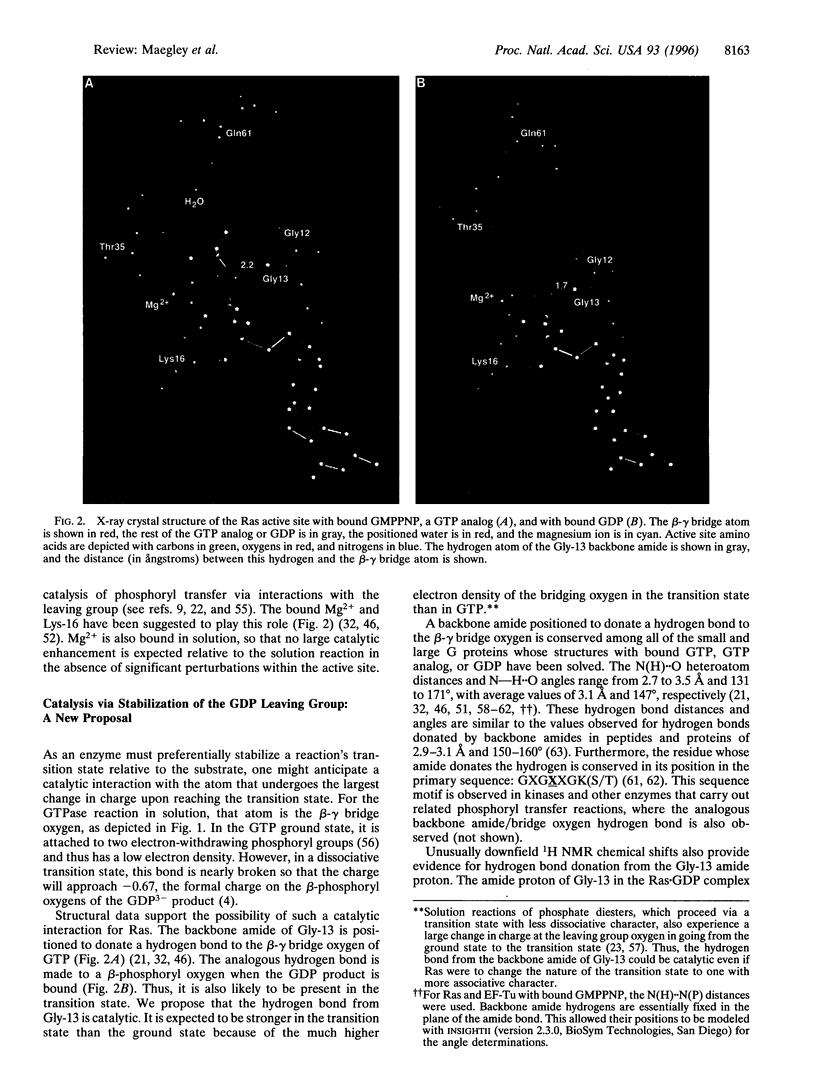
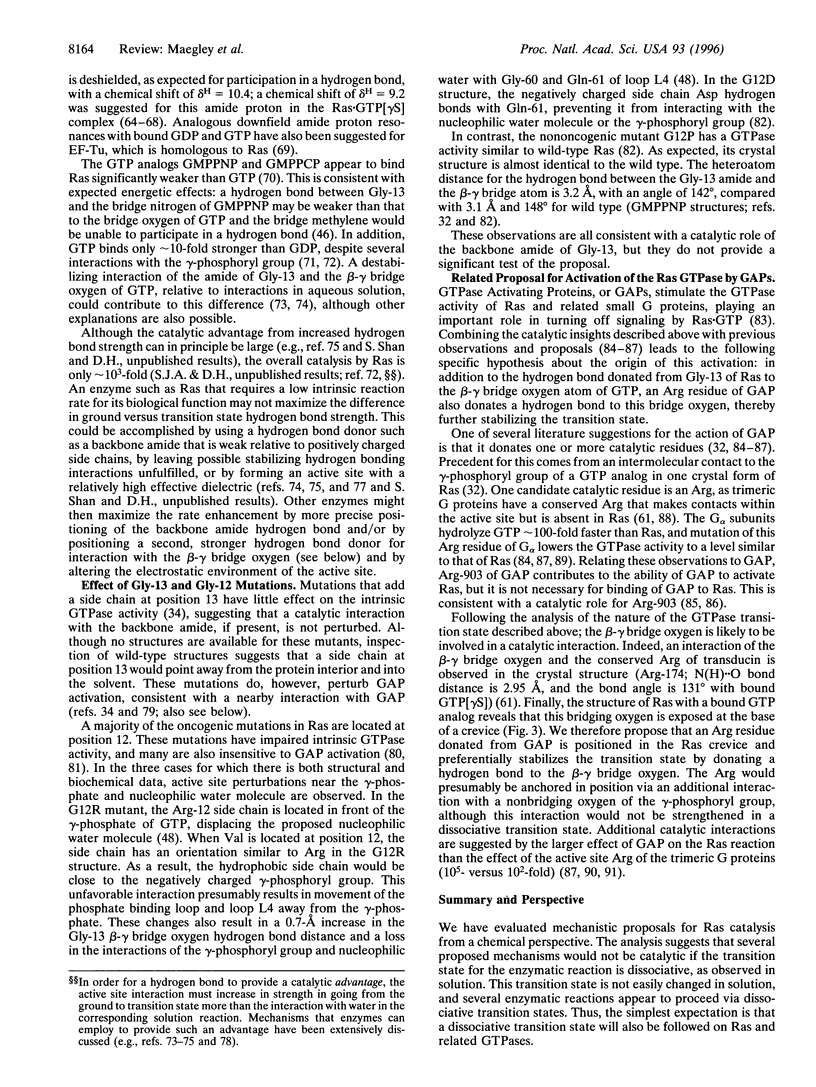
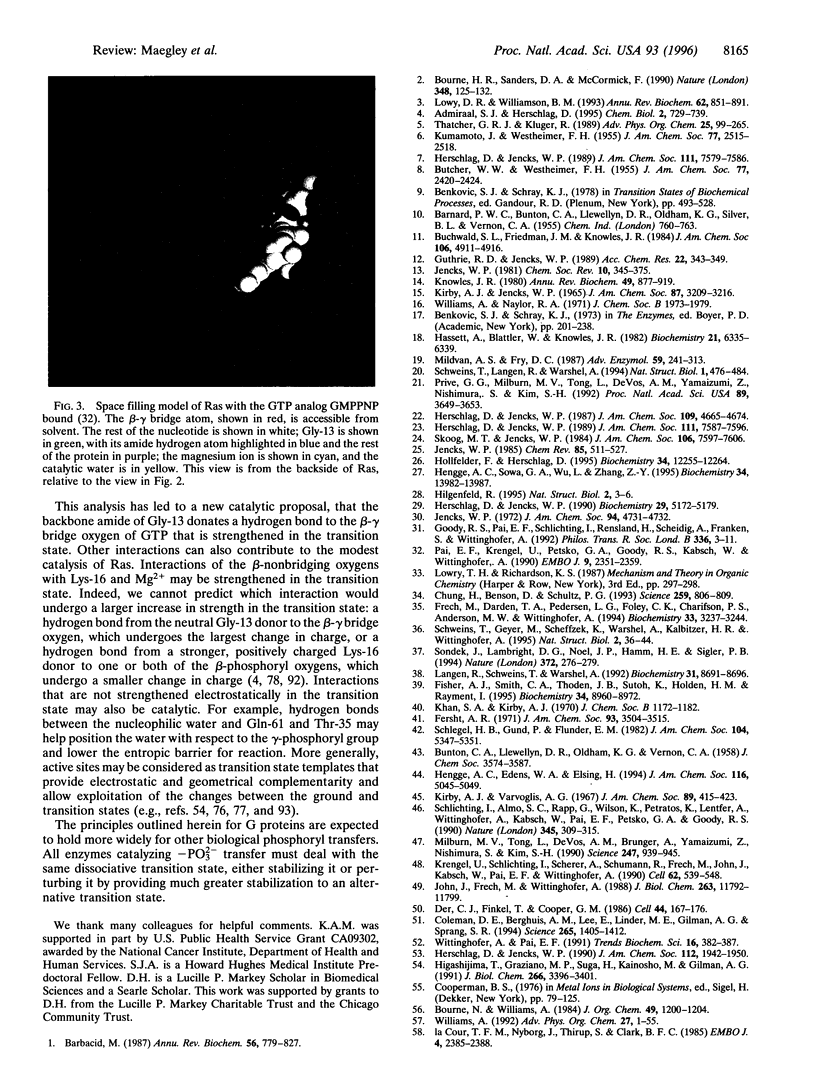
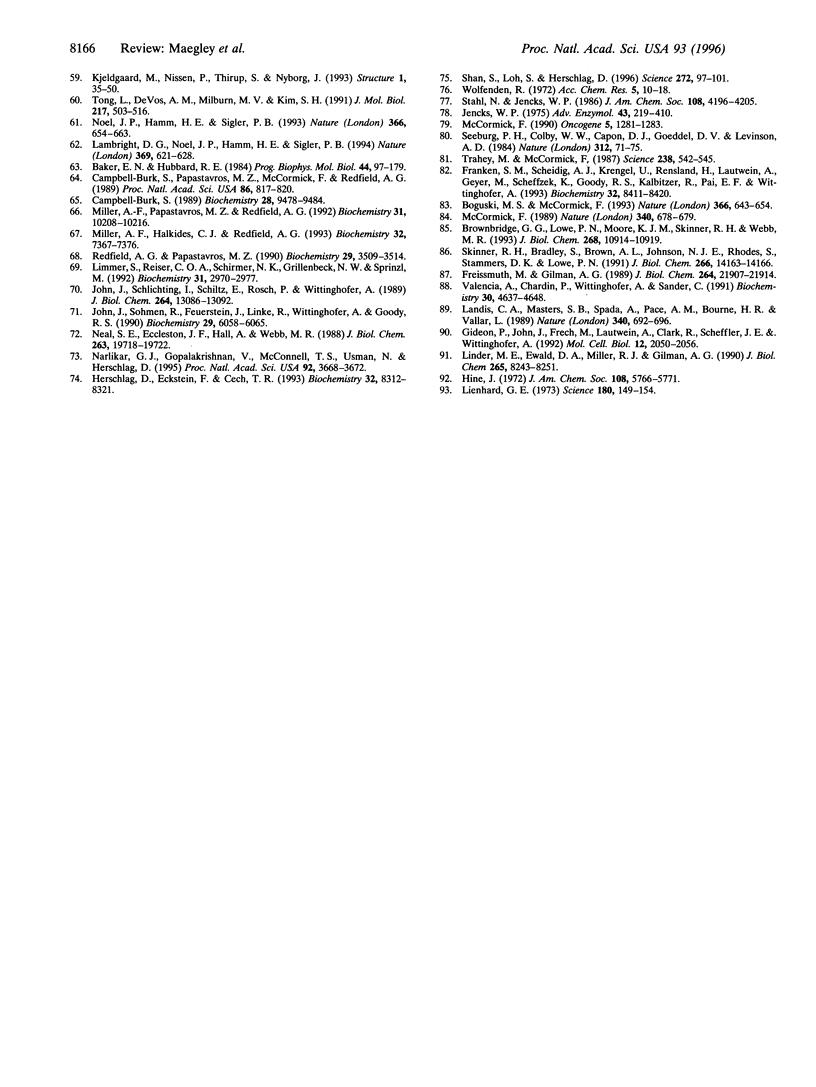
Images in this article
Selected References
These references are in PubMed. This may not be the complete list of references from this article.
- Admiraal S. J., Herschlag D. Mapping the transition state for ATP hydrolysis: implications for enzymatic catalysis. Chem Biol. 1995 Nov;2(11):729–739. doi: 10.1016/1074-5521(95)90101-9. [DOI] [PubMed] [Google Scholar]
- Baker E. N., Hubbard R. E. Hydrogen bonding in globular proteins. Prog Biophys Mol Biol. 1984;44(2):97–179. doi: 10.1016/0079-6107(84)90007-5. [DOI] [PubMed] [Google Scholar]
- Barbacid M. ras genes. Annu Rev Biochem. 1987;56:779–827. doi: 10.1146/annurev.bi.56.070187.004023. [DOI] [PubMed] [Google Scholar]
- Boguski M. S., McCormick F. Proteins regulating Ras and its relatives. Nature. 1993 Dec 16;366(6456):643–654. doi: 10.1038/366643a0. [DOI] [PubMed] [Google Scholar]
- Bourne H. R., Sanders D. A., McCormick F. The GTPase superfamily: a conserved switch for diverse cell functions. Nature. 1990 Nov 8;348(6297):125–132. doi: 10.1038/348125a0. [DOI] [PubMed] [Google Scholar]
- Brownbridge G. G., Lowe P. N., Moore K. J., Skinner R. H., Webb M. R. Interaction of GTPase activating proteins (GAPs) with p21ras measured by a novel fluorescence anisotropy method. Essential role of Arg-903 of GAP in activation of GTP hydrolysis on p21ras. J Biol Chem. 1993 May 25;268(15):10914–10919. [PubMed] [Google Scholar]
- Burk S. C., Papastavros M. Z., McCormick F., Redfield A. G. Identification of resonances from an oncogenic activating locus of human N-RAS-encoded p21 protein using isotope-edited NMR. Proc Natl Acad Sci U S A. 1989 Feb;86(3):817–820. doi: 10.1073/pnas.86.3.817. [DOI] [PMC free article] [PubMed] [Google Scholar]
- Campbell-Burk S. Structural and dynamic differences between normal and transforming N-ras gene products: a 31P and isotope-edited 1H NMR study. Biochemistry. 1989 Nov 28;28(24):9478–9484. doi: 10.1021/bi00450a035. [DOI] [PubMed] [Google Scholar]
- Chung H. H., Benson D. R., Schultz P. G. Probing the structure and mechanism of Ras protein with an expanded genetic code. Science. 1993 Feb 5;259(5096):806–809. doi: 10.1126/science.8430333. [DOI] [PubMed] [Google Scholar]
- Coleman D. E., Berghuis A. M., Lee E., Linder M. E., Gilman A. G., Sprang S. R. Structures of active conformations of Gi alpha 1 and the mechanism of GTP hydrolysis. Science. 1994 Sep 2;265(5177):1405–1412. doi: 10.1126/science.8073283. [DOI] [PubMed] [Google Scholar]
- Der C. J., Finkel T., Cooper G. M. Biological and biochemical properties of human rasH genes mutated at codon 61. Cell. 1986 Jan 17;44(1):167–176. doi: 10.1016/0092-8674(86)90495-2. [DOI] [PubMed] [Google Scholar]
- Fersht A. R. Acyl-transfer reactions of amides and esters with alcohols and thiols. A reference system for the serine and cysteine proteinases. Concerning the N protonation of amides and amide-imidate equilibria. J Am Chem Soc. 1971 Jul 14;93(14):3504–3515. doi: 10.1021/ja00743a035. [DOI] [PubMed] [Google Scholar]
- Fisher A. J., Smith C. A., Thoden J. B., Smith R., Sutoh K., Holden H. M., Rayment I. X-ray structures of the myosin motor domain of Dictyostelium discoideum complexed with MgADP.BeFx and MgADP.AlF4-. Biochemistry. 1995 Jul 18;34(28):8960–8972. doi: 10.1021/bi00028a004. [DOI] [PubMed] [Google Scholar]
- Franken S. M., Scheidig A. J., Krengel U., Rensland H., Lautwein A., Geyer M., Scheffzek K., Goody R. S., Kalbitzer H. R., Pai E. F. Three-dimensional structures and properties of a transforming and a nontransforming glycine-12 mutant of p21H-ras. Biochemistry. 1993 Aug 24;32(33):8411–8420. doi: 10.1021/bi00084a005. [DOI] [PubMed] [Google Scholar]
- Frech M., Darden T. A., Pedersen L. G., Foley C. K., Charifson P. S., Anderson M. W., Wittinghofer A. Role of glutamine-61 in the hydrolysis of GTP by p21H-ras: an experimental and theoretical study. Biochemistry. 1994 Mar 22;33(11):3237–3244. doi: 10.1021/bi00177a014. [DOI] [PubMed] [Google Scholar]
- Freissmuth M., Gilman A. G. Mutations of GS alpha designed to alter the reactivity of the protein with bacterial toxins. Substitutions at ARG187 result in loss of GTPase activity. J Biol Chem. 1989 Dec 25;264(36):21907–21914. [PubMed] [Google Scholar]
- Gideon P., John J., Frech M., Lautwein A., Clark R., Scheffler J. E., Wittinghofer A. Mutational and kinetic analyses of the GTPase-activating protein (GAP)-p21 interaction: the C-terminal domain of GAP is not sufficient for full activity. Mol Cell Biol. 1992 May;12(5):2050–2056. doi: 10.1128/mcb.12.5.2050. [DOI] [PMC free article] [PubMed] [Google Scholar]
- Goody R. S., Pai E. F., Schlichting I., Rensland H., Scheidig A., Franken S., Wittinghofer A. Studies on the structure and mechanism of H-ras p21. Philos Trans R Soc Lond B Biol Sci. 1992 Apr 29;336(1276):3–11. doi: 10.1098/rstb.1992.0037. [DOI] [PubMed] [Google Scholar]
- Hassett A., Blättler W., Knowles J. R. Pyruvate kinase: is the mechanism of phospho transfer associative or dissociative? Biochemistry. 1982 Dec 7;21(25):6335–6340. doi: 10.1021/bi00268a002. [DOI] [PubMed] [Google Scholar]
- Hengge A. C., Sowa G. A., Wu L., Zhang Z. Y. Nature of the transition state of the protein-tyrosine phosphatase-catalyzed reaction. Biochemistry. 1995 Oct 31;34(43):13982–13987. doi: 10.1021/bi00043a003. [DOI] [PubMed] [Google Scholar]
- Herschlag D., Eckstein F., Cech T. R. The importance of being ribose at the cleavage site in the Tetrahymena ribozyme reaction. Biochemistry. 1993 Aug 17;32(32):8312–8321. doi: 10.1021/bi00083a035. [DOI] [PubMed] [Google Scholar]
- Herschlag D., Jencks W. P. Catalysis of the hydrolysis of phosphorylated pyridines by Mg(OH)+: a possible model for enzymatic phosphoryl transfer. Biochemistry. 1990 May 29;29(21):5172–5179. doi: 10.1021/bi00473a025. [DOI] [PubMed] [Google Scholar]
- Higashijima T., Graziano M. P., Suga H., Kainosho M., Gilman A. G. 19F and 31P NMR spectroscopy of G protein alpha subunits. Mechanism of activation by Al3+ and F-. J Biol Chem. 1991 Feb 25;266(6):3396–3401. [PubMed] [Google Scholar]
- Hilgenfeld R. How do the GTPases really work? Nat Struct Biol. 1995 Jan;2(1):3–6. doi: 10.1038/nsb0195-3. [DOI] [PubMed] [Google Scholar]
- Hollfelder F., Herschlag D. The nature of the transition state for enzyme-catalyzed phosphoryl transfer. Hydrolysis of O-aryl phosphorothioates by alkaline phosphatase. Biochemistry. 1995 Sep 26;34(38):12255–12264. doi: 10.1021/bi00038a021. [DOI] [PubMed] [Google Scholar]
- Jencks W. P. Binding energy, specificity, and enzymic catalysis: the circe effect. Adv Enzymol Relat Areas Mol Biol. 1975;43:219–410. doi: 10.1002/9780470122884.ch4. [DOI] [PubMed] [Google Scholar]
- John J., Frech M., Wittinghofer A. Biochemical properties of Ha-ras encoded p21 mutants and mechanism of the autophosphorylation reaction. J Biol Chem. 1988 Aug 25;263(24):11792–11799. [PubMed] [Google Scholar]
- John J., Schlichting I., Schiltz E., Rösch P., Wittinghofer A. C-terminal truncation of p21H preserves crucial kinetic and structural properties. J Biol Chem. 1989 Aug 5;264(22):13086–13092. [PubMed] [Google Scholar]
- John J., Sohmen R., Feuerstein J., Linke R., Wittinghofer A., Goody R. S. Kinetics of interaction of nucleotides with nucleotide-free H-ras p21. Biochemistry. 1990 Jun 26;29(25):6058–6065. doi: 10.1021/bi00477a025. [DOI] [PubMed] [Google Scholar]
- Kjeldgaard M., Nissen P., Thirup S., Nyborg J. The crystal structure of elongation factor EF-Tu from Thermus aquaticus in the GTP conformation. Structure. 1993 Sep 15;1(1):35–50. doi: 10.1016/0969-2126(93)90007-4. [DOI] [PubMed] [Google Scholar]
- Knowles J. R. Enzyme-catalyzed phosphoryl transfer reactions. Annu Rev Biochem. 1980;49:877–919. doi: 10.1146/annurev.bi.49.070180.004305. [DOI] [PubMed] [Google Scholar]
- Krengel U., Schlichting I., Scherer A., Schumann R., Frech M., John J., Kabsch W., Pai E. F., Wittinghofer A. Three-dimensional structures of H-ras p21 mutants: molecular basis for their inability to function as signal switch molecules. Cell. 1990 Aug 10;62(3):539–548. doi: 10.1016/0092-8674(90)90018-a. [DOI] [PubMed] [Google Scholar]
- Lambright D. G., Noel J. P., Hamm H. E., Sigler P. B. Structural determinants for activation of the alpha-subunit of a heterotrimeric G protein. Nature. 1994 Jun 23;369(6482):621–628. doi: 10.1038/369621a0. [DOI] [PubMed] [Google Scholar]
- Landis C. A., Masters S. B., Spada A., Pace A. M., Bourne H. R., Vallar L. GTPase inhibiting mutations activate the alpha chain of Gs and stimulate adenylyl cyclase in human pituitary tumours. Nature. 1989 Aug 31;340(6236):692–696. doi: 10.1038/340692a0. [DOI] [PubMed] [Google Scholar]
- Langen R., Schweins T., Warshel A. On the mechanism of guanosine triphosphate hydrolysis in ras p21 proteins. Biochemistry. 1992 Sep 22;31(37):8691–8696. doi: 10.1021/bi00152a002. [DOI] [PubMed] [Google Scholar]
- Lienhard G. E. Enzymatic catalysis and transition-state theory. Science. 1973 Apr 15;180(4082):149–154. doi: 10.1126/science.180.4082.149. [DOI] [PubMed] [Google Scholar]
- Limmer S., Reiser C. O., Schirmer N. K., Grillenbeck N. W., Sprinzl M. Nucleotide binding and GTP hydrolysis by elongation factor Tu from Thermus thermophilus as monitored by proton NMR. Biochemistry. 1992 Mar 24;31(11):2970–2977. doi: 10.1021/bi00126a018. [DOI] [PubMed] [Google Scholar]
- Linder M. E., Ewald D. A., Miller R. J., Gilman A. G. Purification and characterization of Go alpha and three types of Gi alpha after expression in Escherichia coli. J Biol Chem. 1990 May 15;265(14):8243–8251. [PubMed] [Google Scholar]
- Lowy D. R., Willumsen B. M. Function and regulation of ras. Annu Rev Biochem. 1993;62:851–891. doi: 10.1146/annurev.bi.62.070193.004223. [DOI] [PubMed] [Google Scholar]
- McCormick F. Gasp: not just another oncogene. Nature. 1989 Aug 31;340(6236):678–679. doi: 10.1038/340678a0. [DOI] [PubMed] [Google Scholar]
- McCormick F. The world according to GAP. Oncogene. 1990 Sep;5(9):1281–1283. [PubMed] [Google Scholar]
- Milburn M. V., Tong L., deVos A. M., Brünger A., Yamaizumi Z., Nishimura S., Kim S. H. Molecular switch for signal transduction: structural differences between active and inactive forms of protooncogenic ras proteins. Science. 1990 Feb 23;247(4945):939–945. doi: 10.1126/science.2406906. [DOI] [PubMed] [Google Scholar]
- Mildvan A. S., Fry D. C. NMR studies of the mechanism of enzyme action. Adv Enzymol Relat Areas Mol Biol. 1987;59:241–313. doi: 10.1002/9780470123058.ch6. [DOI] [PubMed] [Google Scholar]
- Miller A. F., Halkides C. J., Redfield A. G. An NMR comparison of the changes produced by different guanosine 5'-triphosphate analogs in wild-type and oncogenic mutant p21ras. Biochemistry. 1993 Jul 27;32(29):7367–7376. doi: 10.1021/bi00080a006. [DOI] [PubMed] [Google Scholar]
- Miller A. F., Papastavros M. Z., Redfield A. G. NMR studies of the conformational change in human N-p21ras produced by replacement of bound GDP with the GTP analog GTP gamma S. Biochemistry. 1992 Oct 27;31(42):10208–10216. doi: 10.1021/bi00157a007. [DOI] [PubMed] [Google Scholar]
- Narlikar G. J., Gopalakrishnan V., McConnell T. S., Usman N., Herschlag D. Use of binding energy by an RNA enzyme for catalysis by positioning and substrate destabilization. Proc Natl Acad Sci U S A. 1995 Apr 25;92(9):3668–3672. doi: 10.1073/pnas.92.9.3668. [DOI] [PMC free article] [PubMed] [Google Scholar]
- Neal S. E., Eccleston J. F., Hall A., Webb M. R. Kinetic analysis of the hydrolysis of GTP by p21N-ras. The basal GTPase mechanism. J Biol Chem. 1988 Dec 25;263(36):19718–19722. [PubMed] [Google Scholar]
- Noel J. P., Hamm H. E., Sigler P. B. The 2.2 A crystal structure of transducin-alpha complexed with GTP gamma S. Nature. 1993 Dec 16;366(6456):654–663. doi: 10.1038/366654a0. [DOI] [PubMed] [Google Scholar]
- Pai E. F., Krengel U., Petsko G. A., Goody R. S., Kabsch W., Wittinghofer A. Refined crystal structure of the triphosphate conformation of H-ras p21 at 1.35 A resolution: implications for the mechanism of GTP hydrolysis. EMBO J. 1990 Aug;9(8):2351–2359. doi: 10.1002/j.1460-2075.1990.tb07409.x. [DOI] [PMC free article] [PubMed] [Google Scholar]
- Privé G. G., Milburn M. V., Tong L., de Vos A. M., Yamaizumi Z., Nishimura S., Kim S. H. X-ray crystal structures of transforming p21 ras mutants suggest a transition-state stabilization mechanism for GTP hydrolysis. Proc Natl Acad Sci U S A. 1992 Apr 15;89(8):3649–3653. doi: 10.1073/pnas.89.8.3649. [DOI] [PMC free article] [PubMed] [Google Scholar]
- Redfield A. G., Papastavros M. Z. NMR study of the phosphoryl binding loop in purine nucleotide proteins: evidence for strong hydrogen bonding in human N-ras p21. Biochemistry. 1990 Apr 10;29(14):3509–3514. doi: 10.1021/bi00466a013. [DOI] [PubMed] [Google Scholar]
- Schlichting I., Almo S. C., Rapp G., Wilson K., Petratos K., Lentfer A., Wittinghofer A., Kabsch W., Pai E. F., Petsko G. A. Time-resolved X-ray crystallographic study of the conformational change in Ha-Ras p21 protein on GTP hydrolysis. Nature. 1990 May 24;345(6273):309–315. doi: 10.1038/345309a0. [DOI] [PubMed] [Google Scholar]
- Schweins T., Geyer M., Scheffzek K., Warshel A., Kalbitzer H. R., Wittinghofer A. Substrate-assisted catalysis as a mechanism for GTP hydrolysis of p21ras and other GTP-binding proteins. Nat Struct Biol. 1995 Jan;2(1):36–44. doi: 10.1038/nsb0195-36. [DOI] [PubMed] [Google Scholar]
- Schweins T., Langen R., Warshel A. Why have mutagenesis studies not located the general base in ras p21. Nat Struct Biol. 1994 Jul;1(7):476–484. doi: 10.1038/nsb0794-476. [DOI] [PubMed] [Google Scholar]
- Seeburg P. H., Colby W. W., Capon D. J., Goeddel D. V., Levinson A. D. Biological properties of human c-Ha-ras1 genes mutated at codon 12. Nature. 1984 Nov 1;312(5989):71–75. doi: 10.1038/312071a0. [DOI] [PubMed] [Google Scholar]
- Shan S. O., Loh S., Herschlag D. The energetics of hydrogen bonds in model systems: implications for enzymatic catalysis. Science. 1996 Apr 5;272(5258):97–101. doi: 10.1126/science.272.5258.97. [DOI] [PubMed] [Google Scholar]
- Skinner R. H., Bradley S., Brown A. L., Johnson N. J., Rhodes S., Stammers D. K., Lowe P. N. Use of the Glu-Glu-Phe C-terminal epitope for rapid purification of the catalytic domain of normal and mutant ras GTPase-activating proteins. J Biol Chem. 1991 Aug 5;266(22):14163–14166. [PubMed] [Google Scholar]
- Sondek J., Lambright D. G., Noel J. P., Hamm H. E., Sigler P. B. GTPase mechanism of Gproteins from the 1.7-A crystal structure of transducin alpha-GDP-AIF-4. Nature. 1994 Nov 17;372(6503):276–279. doi: 10.1038/372276a0. [DOI] [PubMed] [Google Scholar]
- Tong L. A., de Vos A. M., Milburn M. V., Kim S. H. Crystal structures at 2.2 A resolution of the catalytic domains of normal ras protein and an oncogenic mutant complexed with GDP. J Mol Biol. 1991 Feb 5;217(3):503–516. doi: 10.1016/0022-2836(91)90753-s. [DOI] [PubMed] [Google Scholar]
- Trahey M., McCormick F. A cytoplasmic protein stimulates normal N-ras p21 GTPase, but does not affect oncogenic mutants. Science. 1987 Oct 23;238(4826):542–545. doi: 10.1126/science.2821624. [DOI] [PubMed] [Google Scholar]
- Valencia A., Chardin P., Wittinghofer A., Sander C. The ras protein family: evolutionary tree and role of conserved amino acids. Biochemistry. 1991 May 14;30(19):4637–4648. doi: 10.1021/bi00233a001. [DOI] [PubMed] [Google Scholar]
- Vento S., Hegarty J. E., Bottazzo G., Macchia E., Williams R., Eddleston A. L. Antigen specific suppressor cell function in autoimmune chronic active hepatitis. Lancet. 1984 Jun 2;1(8388):1200–1204. doi: 10.1016/s0140-6736(84)91691-x. [DOI] [PubMed] [Google Scholar]
- Wittinghofer A., Pai E. F. The structure of Ras protein: a model for a universal molecular switch. Trends Biochem Sci. 1991 Oct;16(10):382–387. doi: 10.1016/0968-0004(91)90156-p. [DOI] [PubMed] [Google Scholar]
- la Cour T. F., Nyborg J., Thirup S., Clark B. F. Structural details of the binding of guanosine diphosphate to elongation factor Tu from E. coli as studied by X-ray crystallography. EMBO J. 1985 Sep;4(9):2385–2388. doi: 10.1002/j.1460-2075.1985.tb03943.x. [DOI] [PMC free article] [PubMed] [Google Scholar]