Abstract
This paper identifies all statistically significant dyad symmetry combinations in the Epstein-Barr virus genome. The distribution of long dyad symmetry pairings emphasizes two regions, the 5' third of the 3.1-kilobase-pair (kbp) repeat and the oriP region, the latter essential for Epstein-Barr virus replication during latency. A 600-base-pair (bp) stretch in the 3.1-kbp repeat can establish an extended hairpin loop of stem length in excess of 208 bp of predominantly G + C stacking. Moreover, the 3.1-kbp repeat has the potential to form a wide variety of secondary structures based on juxtapositions of sizable palindromes, close dyad symmetry pairings, and direct repeats. The 3.1-kbp repeat presents several features that portend it as an important control region. The oriP region contains an abundance of statistically significant dyad symmetry combinations that strongly correlate with the "21 X 30 bp" tandem repeat units and four truncated copies of this repeat unit 1 kbp downstream. Each of the units centers on the same approximately 30-bp palindrome. Contrasts in the content and the secondary structure formations associated with the 3.1-kbp repeat units versus those of the oriP region are discussed in relation to viral or cellular function.
Full text
PDF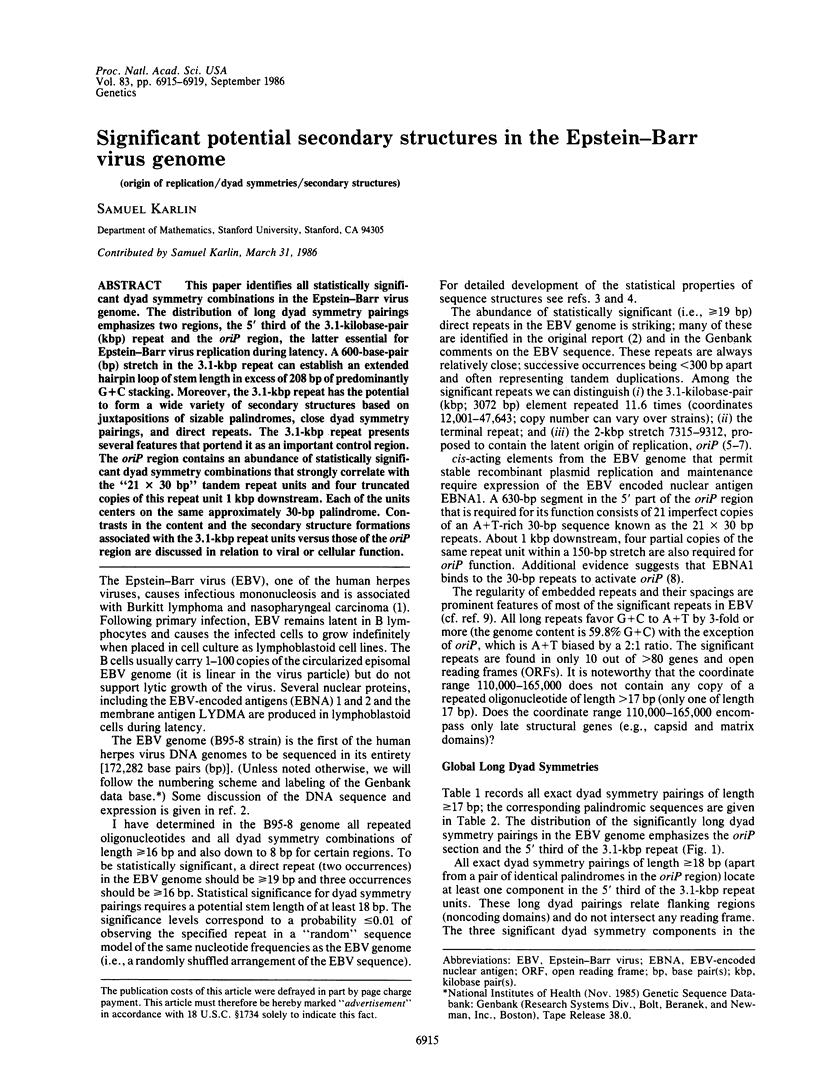
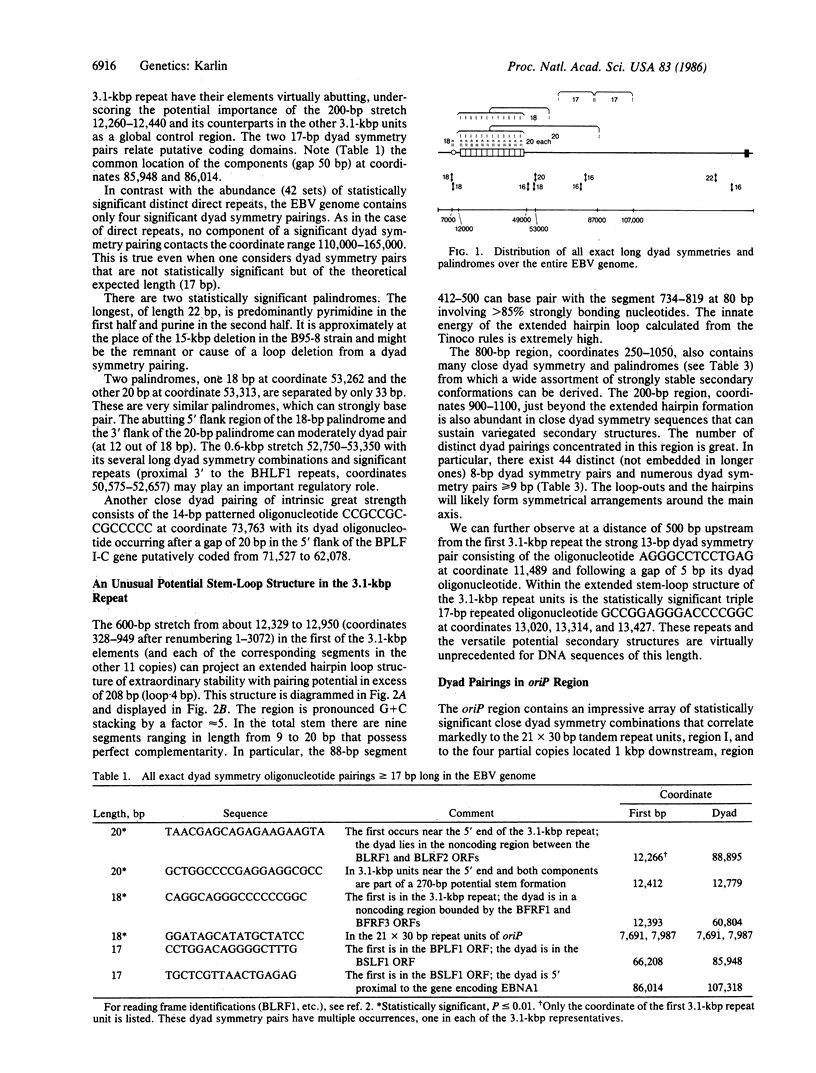
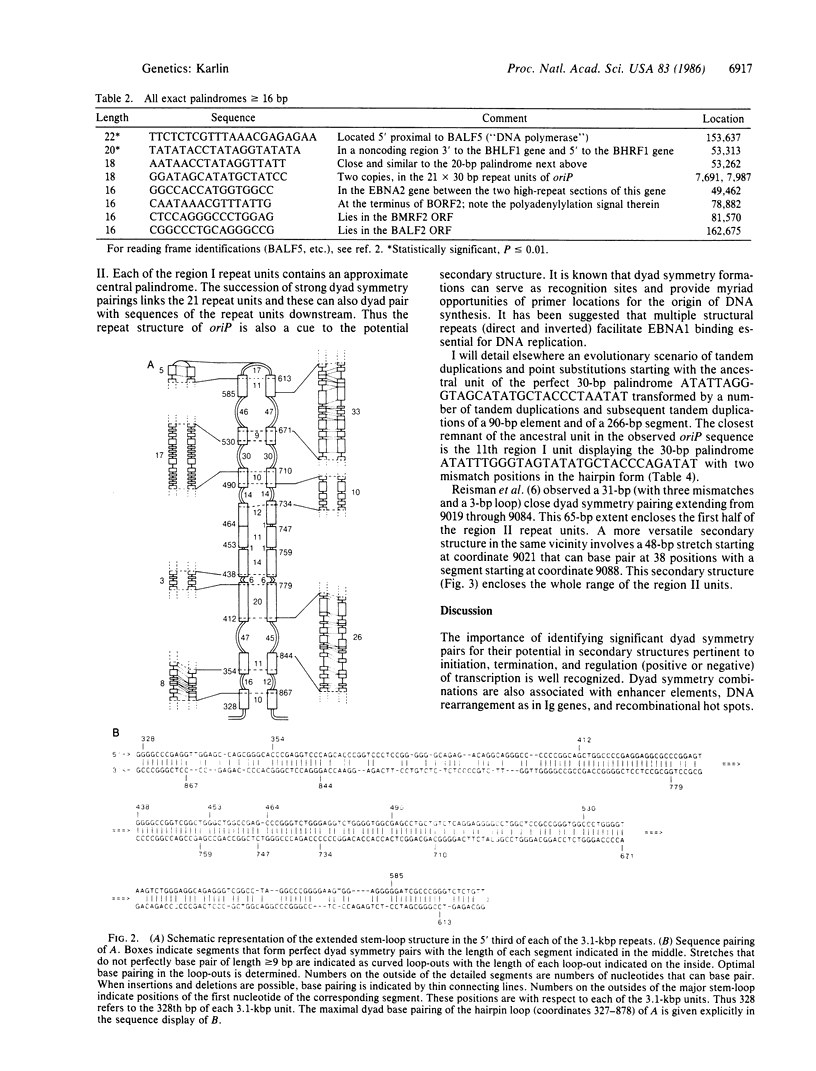
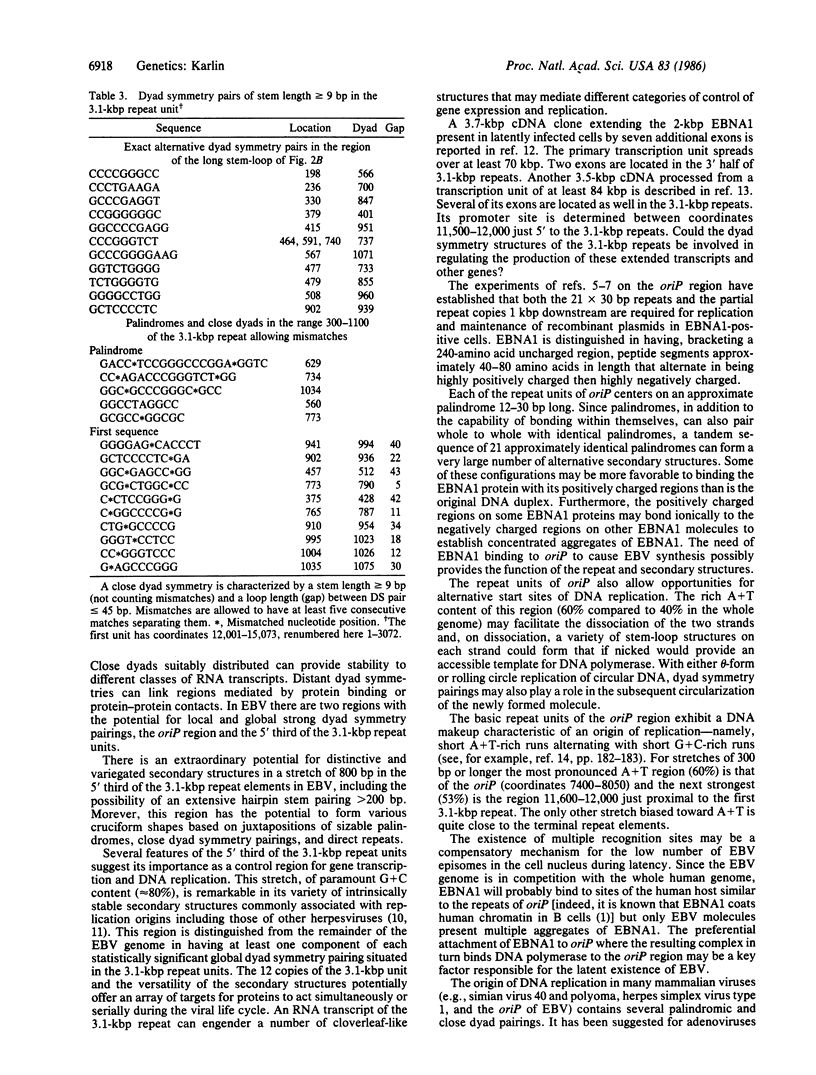
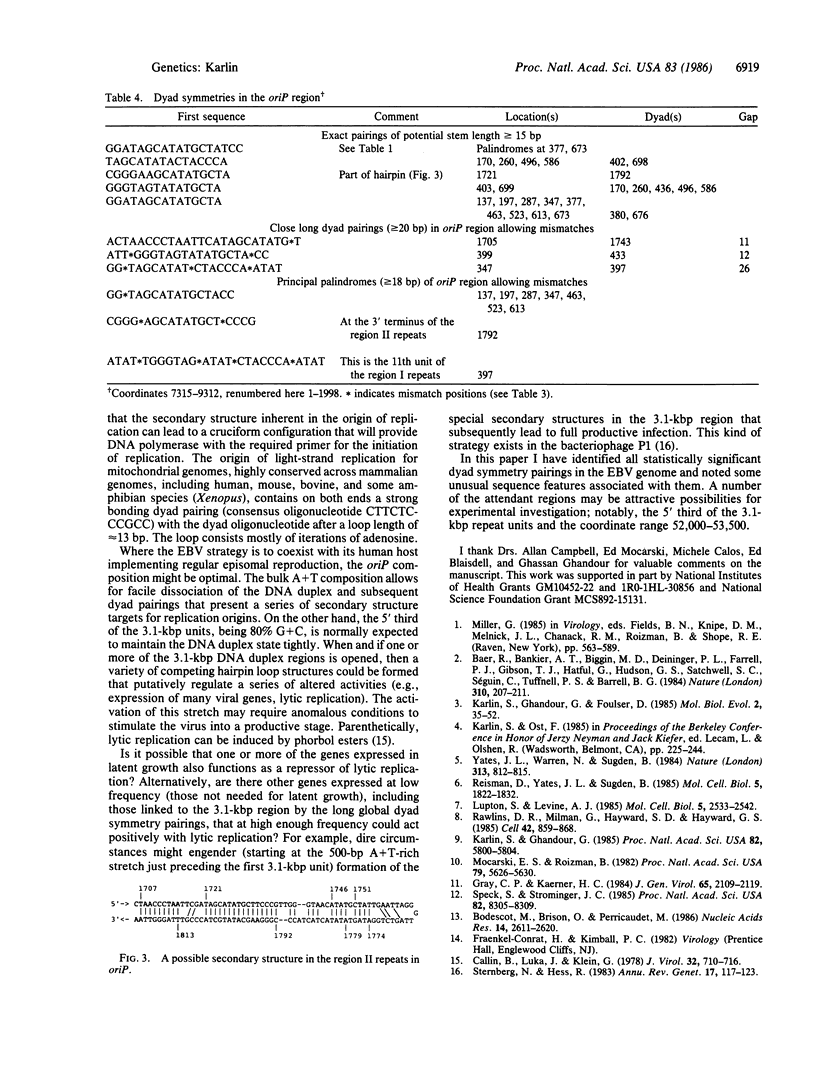
Selected References
These references are in PubMed. This may not be the complete list of references from this article.
- Baer R., Bankier A. T., Biggin M. D., Deininger P. L., Farrell P. J., Gibson T. J., Hatfull G., Hudson G. S., Satchwell S. C., Séguin C. DNA sequence and expression of the B95-8 Epstein-Barr virus genome. Nature. 1984 Jul 19;310(5974):207–211. doi: 10.1038/310207a0. [DOI] [PubMed] [Google Scholar]
- Bodescot M., Brison O., Perricaudet M. An Epstein-Barr virus transcription unit is at least 84 kilobases long. Nucleic Acids Res. 1986 Mar 25;14(6):2611–2620. doi: 10.1093/nar/14.6.2611. [DOI] [PMC free article] [PubMed] [Google Scholar]
- Gray C. P., Kaerner H. C. Sequence of the putative origin of replication in the UL region of herpes simplex virus type 1 ANG DNA. J Gen Virol. 1984 Dec;65(Pt 12):2109–2119. doi: 10.1099/0022-1317-65-12-2109. [DOI] [PubMed] [Google Scholar]
- Kallin B., Luka J., Klein G. Immunochemical characterization of Epstein-Barr virus-associated early and late antigens in n-butyrate-treated P3HR-1 cells. J Virol. 1979 Dec;32(3):710–716. doi: 10.1128/jvi.32.3.710-716.1979. [DOI] [PMC free article] [PubMed] [Google Scholar]
- Karlin S., Ghandour G. Comparative statistics for DNA and protein sequences: single sequence analysis. Proc Natl Acad Sci U S A. 1985 Sep;82(17):5800–5804. doi: 10.1073/pnas.82.17.5800. [DOI] [PMC free article] [PubMed] [Google Scholar]
- Karlin S., Ghandour G., Foulser D. E. DNA sequence comparisons of the human, mouse, and rabbit immunoglobulin kappa gene. Mol Biol Evol. 1985 Jan;2(1):35–52. doi: 10.1093/oxfordjournals.molbev.a040336. [DOI] [PubMed] [Google Scholar]
- Lupton S., Levine A. J. Mapping genetic elements of Epstein-Barr virus that facilitate extrachromosomal persistence of Epstein-Barr virus-derived plasmids in human cells. Mol Cell Biol. 1985 Oct;5(10):2533–2542. doi: 10.1128/mcb.5.10.2533. [DOI] [PMC free article] [PubMed] [Google Scholar]
- Mocarski E. S., Roizman B. Herpesvirus-dependent amplification and inversion of cell-associated viral thymidine kinase gene flanked by viral a sequences and linked to an origin of viral DNA replication. Proc Natl Acad Sci U S A. 1982 Sep;79(18):5626–5630. doi: 10.1073/pnas.79.18.5626. [DOI] [PMC free article] [PubMed] [Google Scholar]
- Rawlins D. R., Milman G., Hayward S. D., Hayward G. S. Sequence-specific DNA binding of the Epstein-Barr virus nuclear antigen (EBNA-1) to clustered sites in the plasmid maintenance region. Cell. 1985 Oct;42(3):859–868. doi: 10.1016/0092-8674(85)90282-x. [DOI] [PubMed] [Google Scholar]
- Reisman D., Yates J., Sugden B. A putative origin of replication of plasmids derived from Epstein-Barr virus is composed of two cis-acting components. Mol Cell Biol. 1985 Aug;5(8):1822–1832. doi: 10.1128/mcb.5.8.1822. [DOI] [PMC free article] [PubMed] [Google Scholar]
- Speck S. H., Strominger J. L. Analysis of the transcript encoding the latent Epstein-Barr virus nuclear antigen I: a potentially polycistronic message generated by long-range splicing of several exons. Proc Natl Acad Sci U S A. 1985 Dec;82(24):8305–8309. doi: 10.1073/pnas.82.24.8305. [DOI] [PMC free article] [PubMed] [Google Scholar]
- Sternberg N., Hoess R. The molecular genetics of bacteriophage P1. Annu Rev Genet. 1983;17:123–154. doi: 10.1146/annurev.ge.17.120183.001011. [DOI] [PubMed] [Google Scholar]
- Yates J. L., Warren N., Sugden B. Stable replication of plasmids derived from Epstein-Barr virus in various mammalian cells. 1985 Feb 28-Mar 6Nature. 313(6005):812–815. doi: 10.1038/313812a0. [DOI] [PubMed] [Google Scholar]