Abstract
Although recent work has reemphasized the general importance of ontogeny in evolution, underlying developmental molecular mechanisms are largely undefined. What heritable ontogenetic mechanisms result in the evolution of new morphologies and functions? Such questions are particularly difficult in the nervous system, in which each of 10(11) neurons forms approximately equal to 10(4) specific interconnections. I propose that specific heritable, trophic interactions during development, which determine cell survival and pathway size, form a substrate for neural evolution. This model is based on the observation that neurons are vastly overproduced during ontogeny; neurons, their pathways and connections are dependent on target-derived trophic factors for developmental survival; and co-innervating, functionally and anatomically distinct neural populations compete for common trophic factors for survival. Focusing on sympathetic and sensory neurons, which require the target-derived, trophic protein nerve growth factor at different times for developmental survival, and which innervate common targets, different classes of ontogenetic evolutionary mechanisms may be characterized. Evolution may occur from heritable changes in the structure of trophic gene products or altered timing of expression. Molecular mechanisms underlying heterochrony are thereby described. The model is directly applicable to evolution of the brain and is testable in a variety of situations.
Full text
PDF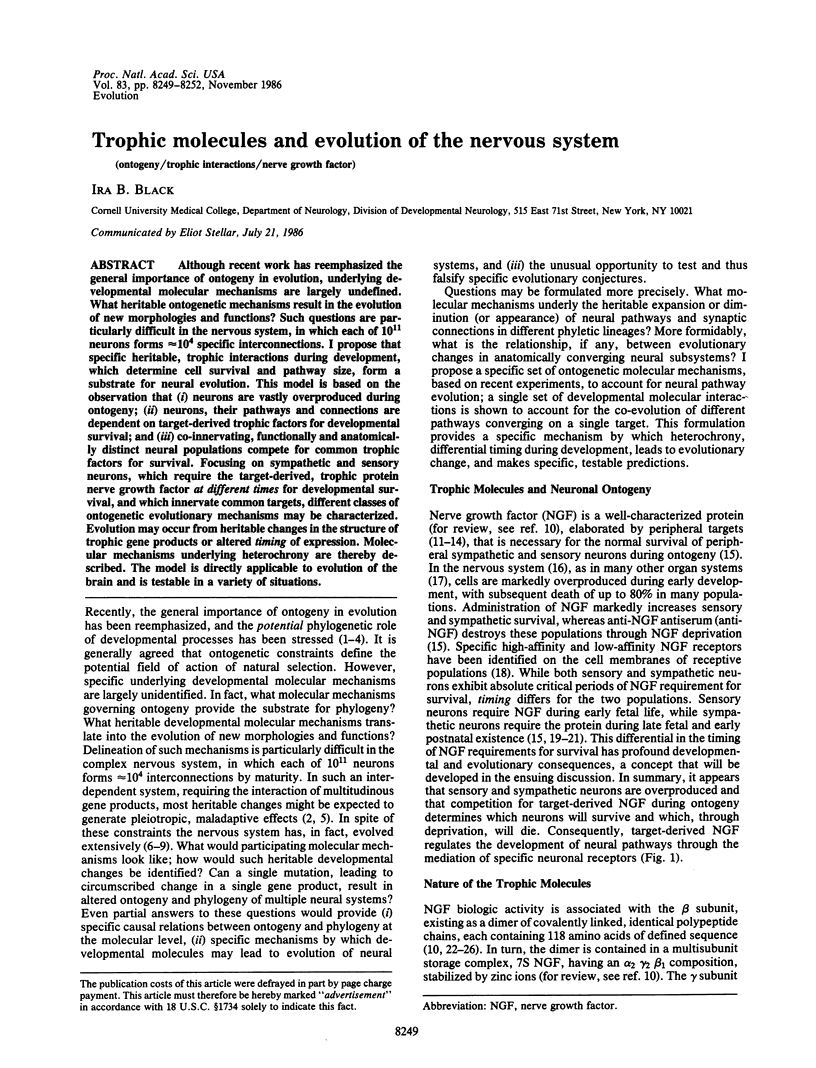
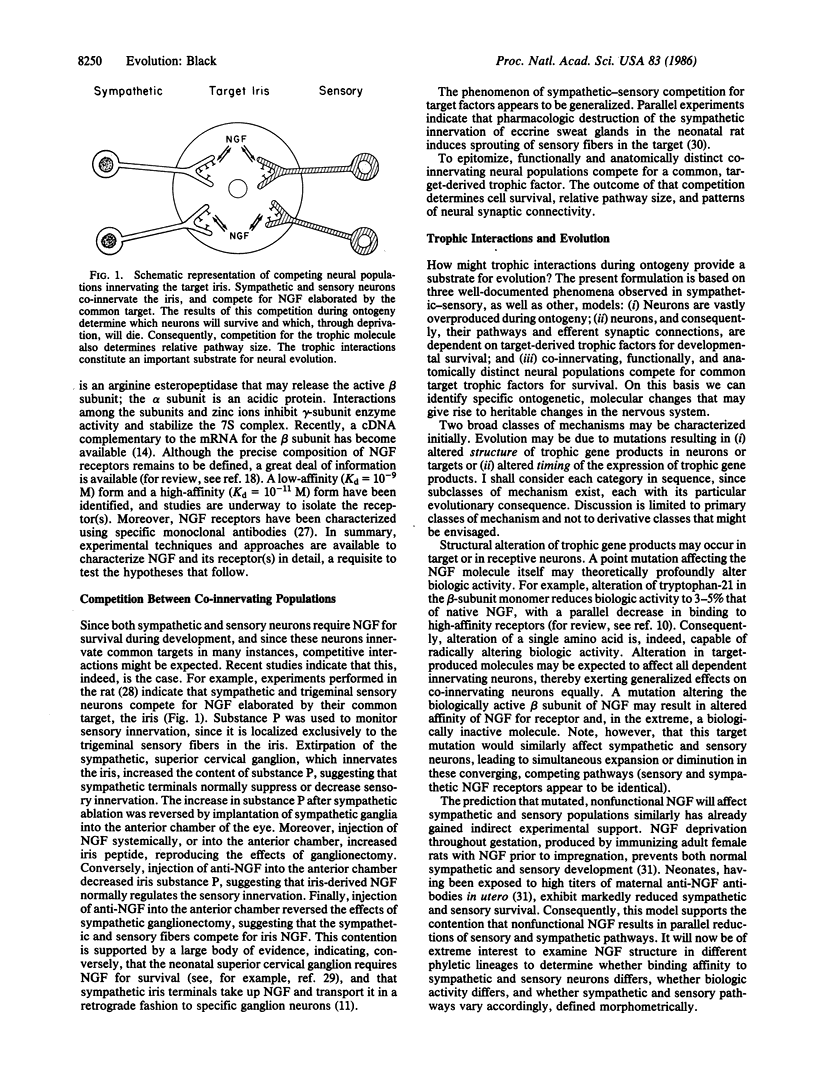
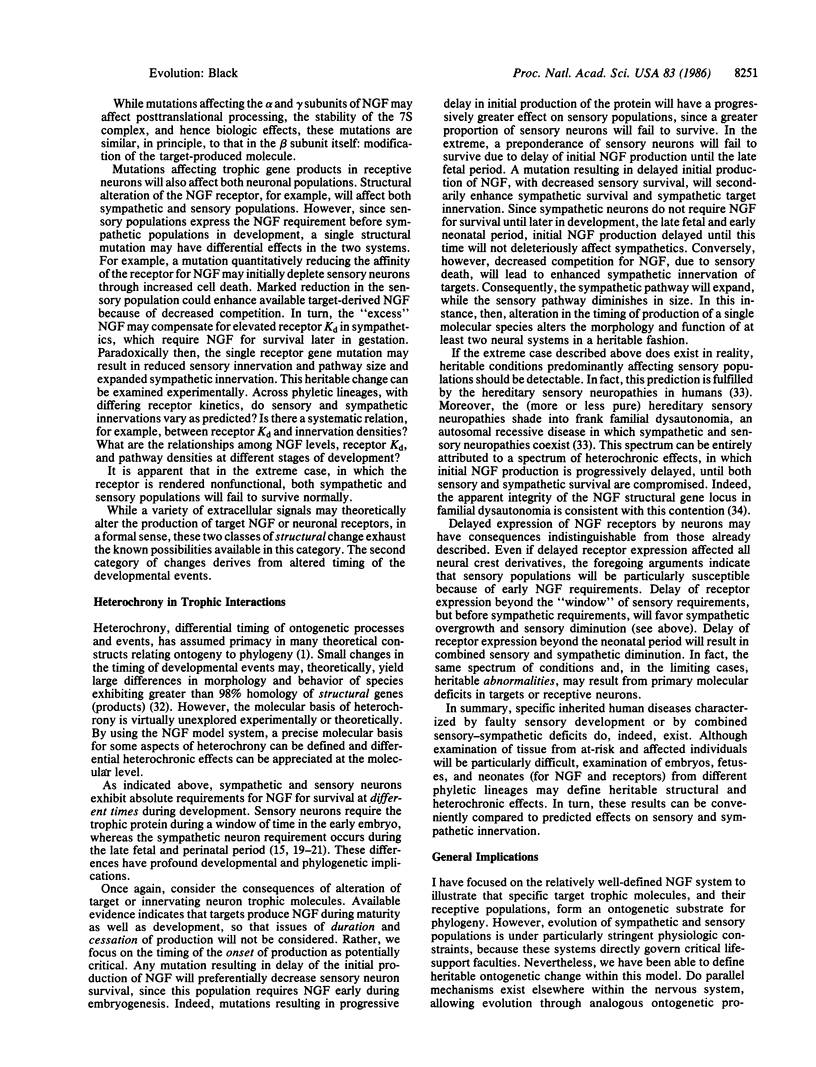
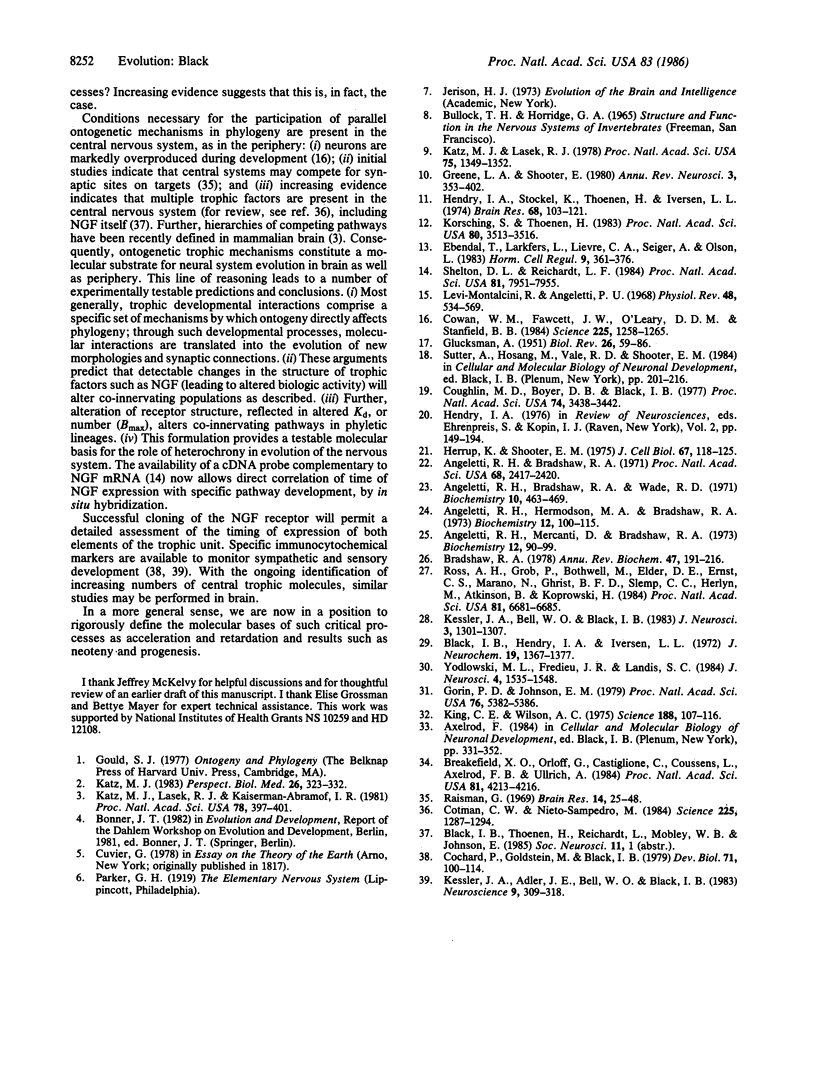
Selected References
These references are in PubMed. This may not be the complete list of references from this article.
- Angeletti R. H., Bradshaw R. A. Nerve growth factor from mouse submaxillary gland: amino acid sequence. Proc Natl Acad Sci U S A. 1971 Oct;68(10):2417–2420. doi: 10.1073/pnas.68.10.2417. [DOI] [PMC free article] [PubMed] [Google Scholar]
- Angeletti R. H., Bradshaw R. A., Wade R. D. Subunit structure and amino acid composition of mouse submaxillary gland nerve growth factor. Biochemistry. 1971 Feb 2;10(3):463–469. doi: 10.1021/bi00779a018. [DOI] [PubMed] [Google Scholar]
- Angeletti R. H., Hermodson M. A., Bradshaw R. A. Amino acid sequences of mouse 2.5S nerve growth factor. II. Isolation and characterization of the thermolytic and peptic peptides and the complete covalent structure. Biochemistry. 1973 Jan 2;12(1):100–115. doi: 10.1021/bi00725a018. [DOI] [PubMed] [Google Scholar]
- Angeletti R. H., Mercanti D., Bradshaw R. A. Amino acid sequences of mouse 2.5S nerve growth factor. I. Isolation and characterization of the soluble tryptic and chymotryptic peptides. Biochemistry. 1973 Jan 2;12(1):90–100. doi: 10.1021/bi00725a017. [DOI] [PubMed] [Google Scholar]
- Black I. B., Hendry I. A., Iversen L. L. Effects of surgical decentralization and nerve growth factor on the maturation of adrenergic neurons in a mouse sympathetic ganglion. J Neurochem. 1972 May;19(5):1367–1377. doi: 10.1111/j.1471-4159.1972.tb01461.x. [DOI] [PubMed] [Google Scholar]
- Bradshaw R. A. Nerve growth factor. Annu Rev Biochem. 1978;47:191–216. doi: 10.1146/annurev.bi.47.070178.001203. [DOI] [PubMed] [Google Scholar]
- Breakefield X. O., Orloff G., Castiglione C., Coussens L., Axelrod F. B., Ullrich A. Structural gene for beta-nerve growth factor not defective in familial dysautonomia. Proc Natl Acad Sci U S A. 1984 Jul;81(13):4213–4216. doi: 10.1073/pnas.81.13.4213. [DOI] [PMC free article] [PubMed] [Google Scholar]
- Cochard P., Goldstein M., Black I. B. Initial development of the noradrenergic phenotype in autonomic neuroblasts of the rat embryo in vivo. Dev Biol. 1979 Jul;71(1):100–114. doi: 10.1016/0012-1606(79)90085-x. [DOI] [PubMed] [Google Scholar]
- Cotman C. W., Nieto-Sampedro M. Cell biology of synaptic plasticity. Science. 1984 Sep 21;225(4668):1287–1294. doi: 10.1126/science.6382610. [DOI] [PubMed] [Google Scholar]
- Coughlin M. D., Boyer D. M., Black I. B. Embryologic development of a mouse sympathetic ganglion in vivo and in vitro. Proc Natl Acad Sci U S A. 1977 Aug;74(8):3438–3442. doi: 10.1073/pnas.74.8.3438. [DOI] [PMC free article] [PubMed] [Google Scholar]
- Cowan W. M., Fawcett J. W., O'Leary D. D., Stanfield B. B. Regressive events in neurogenesis. Science. 1984 Sep 21;225(4668):1258–1265. doi: 10.1126/science.6474175. [DOI] [PubMed] [Google Scholar]
- Gorin P. D., Johnson E. M. Experimental autoimmune model of nerve growth factor deprivation: effects on developing peripheral sympathetic and sensory neurons. Proc Natl Acad Sci U S A. 1979 Oct;76(10):5382–5386. doi: 10.1073/pnas.76.10.5382. [DOI] [PMC free article] [PubMed] [Google Scholar]
- Greene L. A., Shooter E. M. The nerve growth factor: biochemistry, synthesis, and mechanism of action. Annu Rev Neurosci. 1980;3:353–402. doi: 10.1146/annurev.ne.03.030180.002033. [DOI] [PubMed] [Google Scholar]
- Hendry I. A., Stöckel K., Thoenen H., Iversen L. L. The retrograde axonal transport of nerve growth factor. Brain Res. 1974 Mar 15;68(1):103–121. doi: 10.1016/0006-8993(74)90536-8. [DOI] [PubMed] [Google Scholar]
- Herrup K., Shooter E. M. Properties of the beta-nerve growth factor receptor in development. J Cell Biol. 1975 Oct;67(1):118–125. doi: 10.1083/jcb.67.1.118. [DOI] [PMC free article] [PubMed] [Google Scholar]
- Katz M. J., Lasek R. J. Evolution of the nervous system: role of ontogenetic mechanisms in the evolution of matching populations. Proc Natl Acad Sci U S A. 1978 Mar;75(3):1349–1352. doi: 10.1073/pnas.75.3.1349. [DOI] [PMC free article] [PubMed] [Google Scholar]
- Katz M. J., Lasek R. J., Kaiserman-Abramof I. R. Ontophyletics of the nervous system: eyeless mutants illustrate how ontogenetic buffer mechanisms channel evolution. Proc Natl Acad Sci U S A. 1981 Jan;78(1):397–401. doi: 10.1073/pnas.78.1.397. [DOI] [PMC free article] [PubMed] [Google Scholar]
- Kessler J. A., Adler J. E., Bell W. O., Black I. B. Substance P and somatostatin metabolism in sympathetic and special sensory ganglia in vitro. Neuroscience. 1983 Jun;9(2):309–318. doi: 10.1016/0306-4522(83)90296-8. [DOI] [PubMed] [Google Scholar]
- Kessler J. A., Bell W. O., Black I. B. Interactions between the sympathetic and sensory innervation of the iris. J Neurosci. 1983 Jun;3(6):1301–1307. doi: 10.1523/JNEUROSCI.03-06-01301.1983. [DOI] [PMC free article] [PubMed] [Google Scholar]
- King M. C., Wilson A. C. Evolution at two levels in humans and chimpanzees. Science. 1975 Apr 11;188(4184):107–116. doi: 10.1126/science.1090005. [DOI] [PubMed] [Google Scholar]
- Korsching S., Thoenen H. Nerve growth factor in sympathetic ganglia and corresponding target organs of the rat: correlation with density of sympathetic innervation. Proc Natl Acad Sci U S A. 1983 Jun;80(11):3513–3516. doi: 10.1073/pnas.80.11.3513. [DOI] [PMC free article] [PubMed] [Google Scholar]
- Levi-Montalcini R., Angeletti P. U. Nerve growth factor. Physiol Rev. 1968 Jul;48(3):534–569. doi: 10.1152/physrev.1968.48.3.534. [DOI] [PubMed] [Google Scholar]
- Raisman G. Neuronal plasticity in the septal nuclei of the adult rat. Brain Res. 1969 Jun;14(1):25–48. doi: 10.1016/0006-8993(69)90029-8. [DOI] [PubMed] [Google Scholar]
- Ross A. H., Grob P., Bothwell M., Elder D. E., Ernst C. S., Marano N., Ghrist B. F., Slemp C. C., Herlyn M., Atkinson B. Characterization of nerve growth factor receptor in neural crest tumors using monoclonal antibodies. Proc Natl Acad Sci U S A. 1984 Nov;81(21):6681–6685. doi: 10.1073/pnas.81.21.6681. [DOI] [PMC free article] [PubMed] [Google Scholar]
- Shelton D. L., Reichardt L. F. Expression of the beta-nerve growth factor gene correlates with the density of sympathetic innervation in effector organs. Proc Natl Acad Sci U S A. 1984 Dec;81(24):7951–7955. doi: 10.1073/pnas.81.24.7951. [DOI] [PMC free article] [PubMed] [Google Scholar]
- Yodlowski M. L., Fredieu J. R., Landis S. C. Neonatal 6-hydroxydopamine treatment eliminates cholinergic sympathetic innervation and induces sensory sprouting in rat sweat glands. J Neurosci. 1984 Jun;4(6):1535–1548. doi: 10.1523/JNEUROSCI.04-06-01535.1984. [DOI] [PMC free article] [PubMed] [Google Scholar]