Abstract
Gene 32 protein (g32P) isolated from bacteriophage T4-infected Escherichia coli and from an overproduction vector derived from the plasmid pKC30 contains 1 mol of tightly incorporated Zn(II) per mol of protein. A linear incorporation of three molar equivalents of p-hydroxymercuriphenylsulfonate (PMPS) results in a linear release of 1.1 mol of Zn(II) from the protein. Reversal of formation of the g32P-PMPS complex with thiol in the presence of EDTA results in a zinc-free apo-g32P. Cd(II) and Co(II) can be exchanged with the intrinsic Zn(II) ion. The Cd(II) protein shows a charge-transfer band at approximately 250 nm. The Co(II) protein shows a set of absorption bands typical of a tetrahedral Co(II) complex (epsilon max = 660 M-1 X cm-1 at 645 nm), and two intense charge-transfer bands are present at 355 nm (epsilon = 2,250 M-1 X cm-1) and 320 nm (epsilon = 3,175 M-1 X cm-1). These observations are consistent with three cysteines as ligands to the Zn(II) ion in g32P. Zn(II) g32P undergoes precise limited proteolysis by trypsin to produce the small fragments A and B and the core, g32P-(A + B). Under identical conditions, apo-g32P is hydrolyzed rapidly beyond the g32P-(A + B) stage to produce many proteolyzed fragments. Fluorescence quenching experiments show that at low protein concentration apo-g32P has markedly altered binding affinity for poly(dT) relative to native g32P. Three of the four cysteines of g32P are found in a tyrosine-rich sequence corresponding to residues 72-116 and implicated in DNA binding by 1H NMR investigations. Zn(II) appears to provide a conformational element contributing to DNA binding by coordinating the cysteine and possibly histidine side chains in the sequence -Cys-X3-His-X5-Cys-X2-Cys-, residues 77-90, located in the DNA binding domain of g32P.
Full text
PDF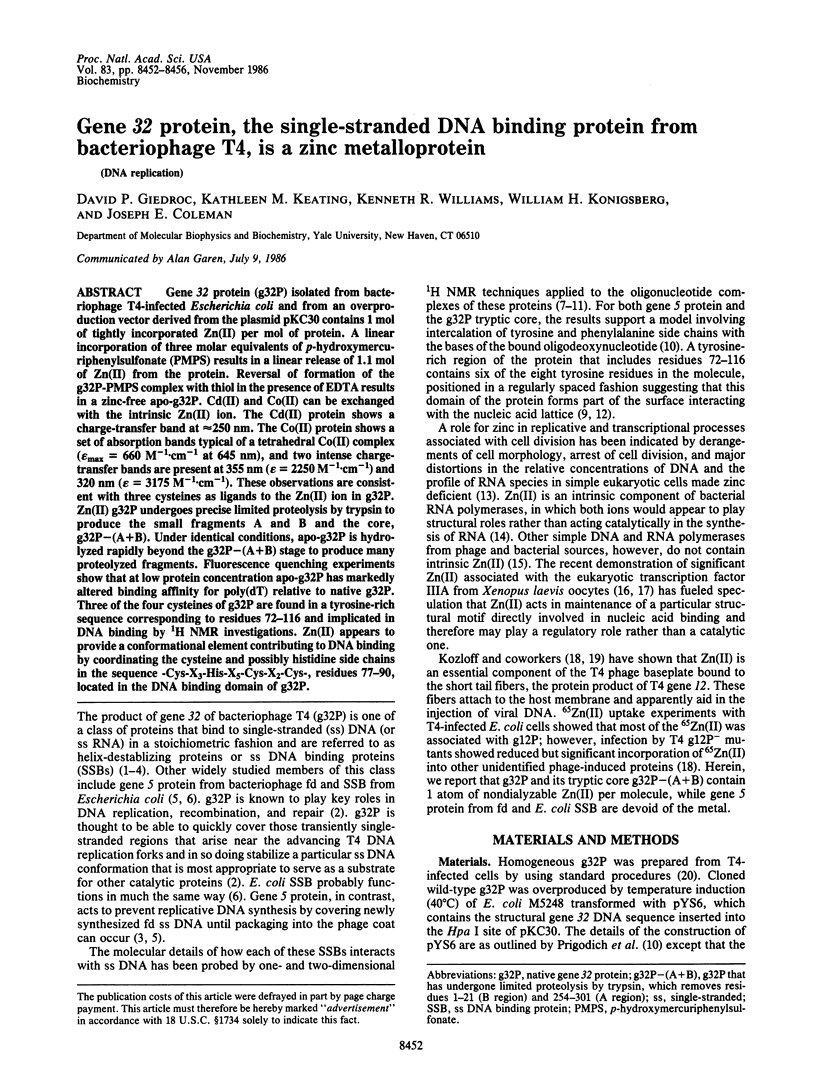
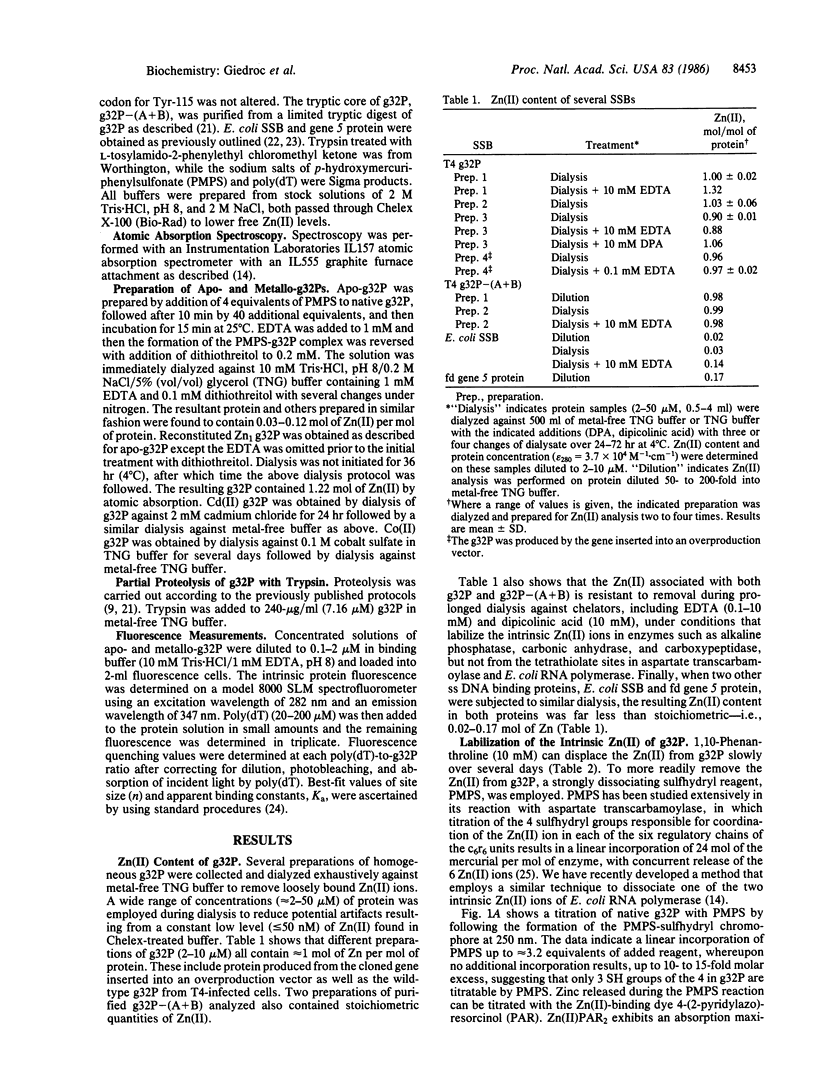
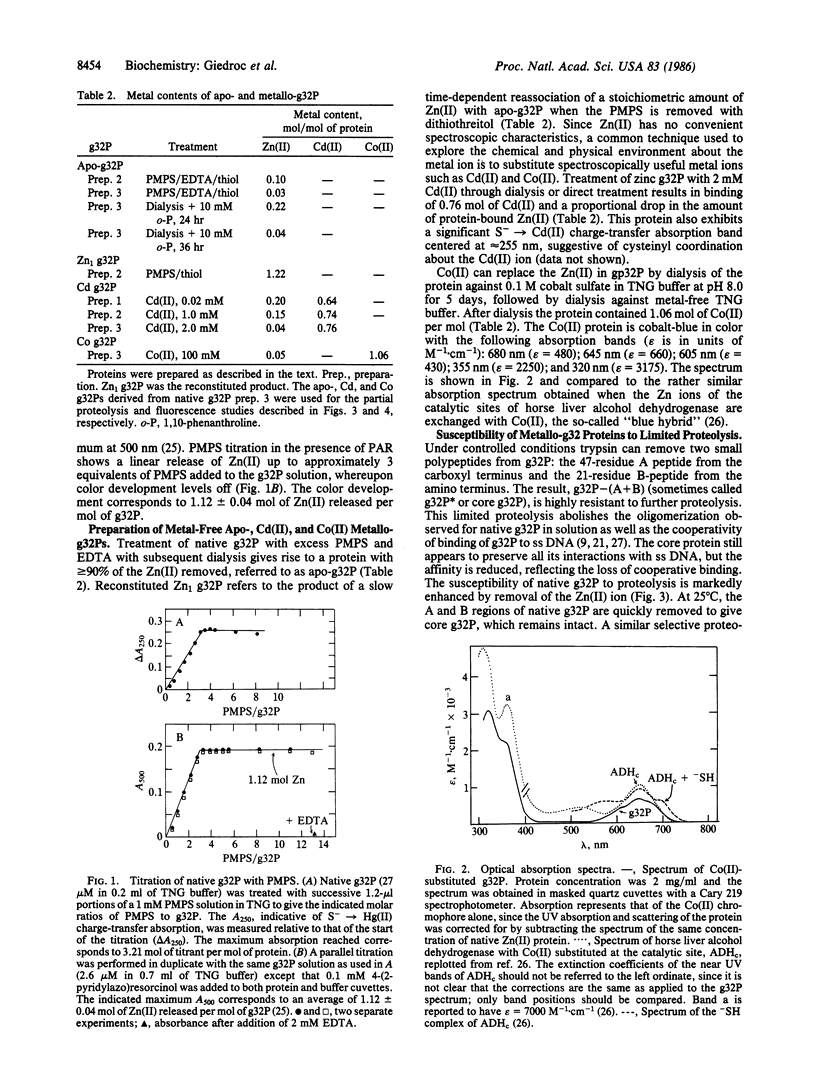
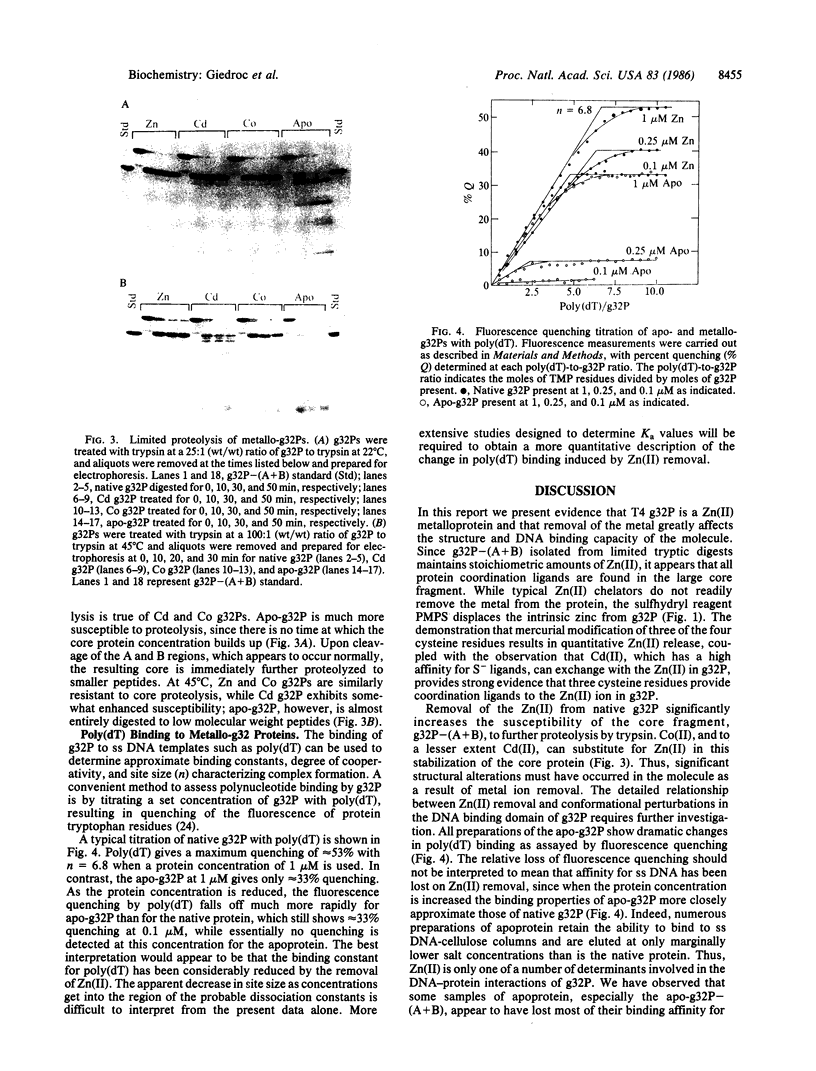
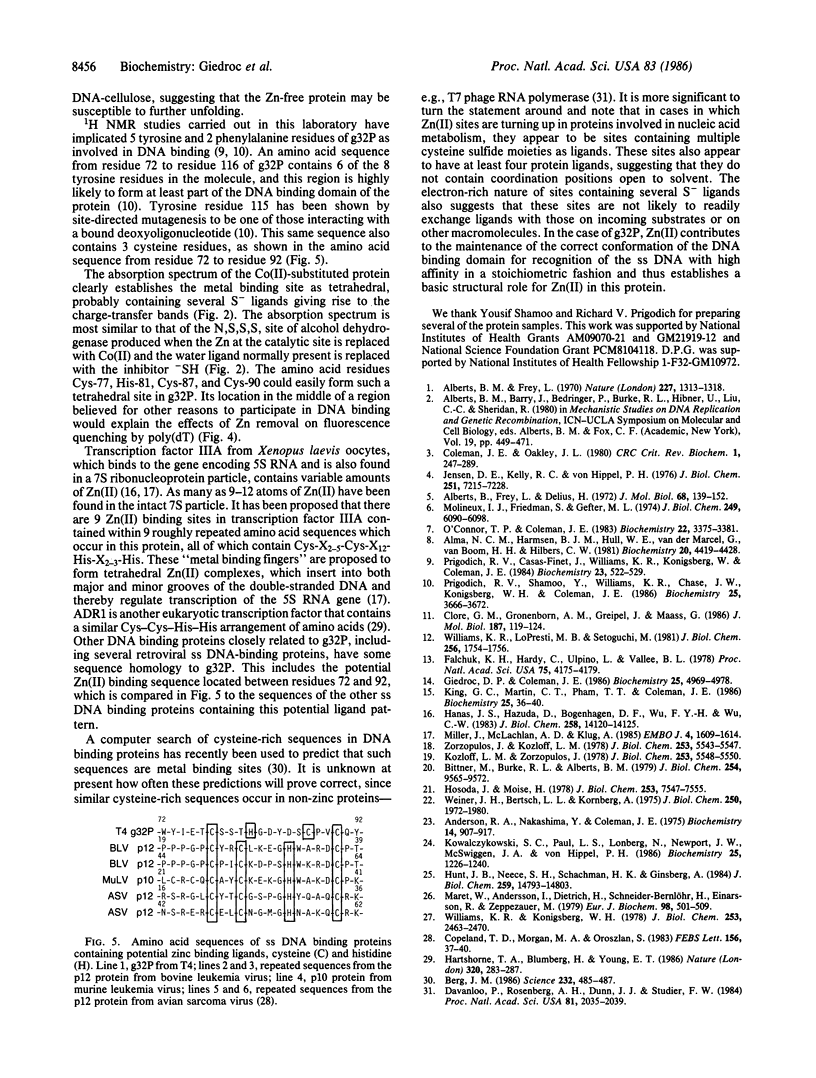
Images in this article
Selected References
These references are in PubMed. This may not be the complete list of references from this article.
- Alberts B. M., Frey L. T4 bacteriophage gene 32: a structural protein in the replication and recombination of DNA. Nature. 1970 Sep 26;227(5265):1313–1318. doi: 10.1038/2271313a0. [DOI] [PubMed] [Google Scholar]
- Alberts B., Frey L., Delius H. Isolation and characterization of gene 5 protein of filamentous bacterial viruses. J Mol Biol. 1972 Jul 14;68(1):139–152. doi: 10.1016/0022-2836(72)90269-0. [DOI] [PubMed] [Google Scholar]
- Alma N. C., Harmsen B. J., Hull W. E., van der Marel G., van Boom J. H., Hilbers C. W. Double-resonance experiments at 500 MHz on gene-5 protein and its complex with octadeoxyriboadenylic acid. Biochemistry. 1981 Jul 21;20(15):4419–4428. doi: 10.1021/bi00518a029. [DOI] [PubMed] [Google Scholar]
- Anderson R. A., Nakashima Y., Coleman J. E. Chemical modifications of functional residues of fd gene 5 DNA-binding protein. Biochemistry. 1975 Mar 11;14(5):907–917. doi: 10.1021/bi00676a006. [DOI] [PubMed] [Google Scholar]
- Berg J. M. Potential metal-binding domains in nucleic acid binding proteins. Science. 1986 Apr 25;232(4749):485–487. doi: 10.1126/science.2421409. [DOI] [PubMed] [Google Scholar]
- Bittner M., Burke R. L., Alberts B. M. Purification of the T4 gene 32 protein free from detectable deoxyribonuclease activities. J Biol Chem. 1979 Oct 10;254(19):9565–9572. [PubMed] [Google Scholar]
- Clore G. M., Gronenborn A. M., Greipel J., Maass G. Conformation of the DNA undecamer 5'd(A-A-G-T-G-T-G-A-T-A-T) bound to the single-stranded DNA binding protein of Escherichia coli. A time-dependent transferred nuclear Overhauser enhancement study. J Mol Biol. 1986 Jan 5;187(1):119–124. doi: 10.1016/0022-2836(86)90411-0. [DOI] [PubMed] [Google Scholar]
- Coleman J. E., Oakley J. L. Physical chemical studies of the structure and function of DNA binding (helix-destabilizing) proteins. CRC Crit Rev Biochem. 1980 Jan;7(3):247–289. doi: 10.3109/10409238009105463. [DOI] [PubMed] [Google Scholar]
- Copeland T. D., Morgan M. A., Oroszlan S. Complete amino acid sequence of the nucleic acid-binding protein of bovine leukemia virus. FEBS Lett. 1983 May 30;156(1):37–40. doi: 10.1016/0014-5793(83)80243-9. [DOI] [PubMed] [Google Scholar]
- Davanloo P., Rosenberg A. H., Dunn J. J., Studier F. W. Cloning and expression of the gene for bacteriophage T7 RNA polymerase. Proc Natl Acad Sci U S A. 1984 Apr;81(7):2035–2039. doi: 10.1073/pnas.81.7.2035. [DOI] [PMC free article] [PubMed] [Google Scholar]
- Falchuk K. H., Hardy C., Ulpino L., Vallee B. L. RNA metabolism, manganese, and RNA polymerases of zinc-sufficient and zinc-deficient Euglena gracilis. Proc Natl Acad Sci U S A. 1978 Sep;75(9):4175–4179. doi: 10.1073/pnas.75.9.4175. [DOI] [PMC free article] [PubMed] [Google Scholar]
- Giedroc D. P., Coleman J. E. Structural and functional differences between the two intrinsic zinc ions of Escherichia coli RNA polymerase. Biochemistry. 1986 Aug 26;25(17):4969–4978. doi: 10.1021/bi00365a037. [DOI] [PubMed] [Google Scholar]
- Hanas J. S., Hazuda D. J., Bogenhagen D. F., Wu F. Y., Wu C. W. Xenopus transcription factor A requires zinc for binding to the 5 S RNA gene. J Biol Chem. 1983 Dec 10;258(23):14120–14125. [PubMed] [Google Scholar]
- Hartshorne T. A., Blumberg H., Young E. T. Sequence homology of the yeast regulatory protein ADR1 with Xenopus transcription factor TFIIIA. Nature. 1986 Mar 20;320(6059):283–287. doi: 10.1038/320283a0. [DOI] [PubMed] [Google Scholar]
- Hosoda J., Moise H. Purification and physicochemical properties of limited proteolysis products of T4 helix destabilizing protein (gene 32 protein). J Biol Chem. 1978 Oct 25;253(20):7547–7558. [PubMed] [Google Scholar]
- Hunt J. B., Neece S. H., Schachman H. K., Ginsburg A. Mercurial-promoted Zn2+ release from Escherichia coli aspartate transcarbamoylase. J Biol Chem. 1984 Dec 10;259(23):14793–14803. [PubMed] [Google Scholar]
- Jensen D. E., Kelly R. C., von Hippel P. H. DNA "melting" proteins. II. Effects of bacteriophage T4 gene 32-protein binding on the conformation and stability of nucleic acid structures. J Biol Chem. 1976 Nov 25;251(22):7215–7228. [PubMed] [Google Scholar]
- King G. C., Martin C. T., Pham T. T., Coleman J. E. Transcription by T7 RNA polymerase is not zinc-dependent and is abolished on amidomethylation of cysteine-347. Biochemistry. 1986 Jan 14;25(1):36–40. doi: 10.1021/bi00349a006. [DOI] [PubMed] [Google Scholar]
- Kowalczykowski S. C., Paul L. S., Lonberg N., Newport J. W., McSwiggen J. A., von Hippel P. H. Cooperative and noncooperative binding of protein ligands to nucleic acid lattices: experimental approaches to the determination of thermodynamic parameters. Biochemistry. 1986 Mar 25;25(6):1226–1240. doi: 10.1021/bi00354a006. [DOI] [PubMed] [Google Scholar]
- Kozloff L. M., Zorzopulos J. Zinc uptake and incorporation into proteins in T4D bacteriophage-infected Escherichia coli. J Biol Chem. 1978 Aug 10;253(15):5548–5550. [PubMed] [Google Scholar]
- Maret W., Andersson I., Dietrich H., Schneider-Bernlöhr H., Einarsson R., Zeppezauer M. Site-specific substituted cobalt(II) horse liver alcohol dehydrogenases. Preparation and characterization in solution, crystalline and immobilized state. Eur J Biochem. 1979 Aug 1;98(2):501–512. doi: 10.1111/j.1432-1033.1979.tb13211.x. [DOI] [PubMed] [Google Scholar]
- Miller J., McLachlan A. D., Klug A. Repetitive zinc-binding domains in the protein transcription factor IIIA from Xenopus oocytes. EMBO J. 1985 Jun;4(6):1609–1614. doi: 10.1002/j.1460-2075.1985.tb03825.x. [DOI] [PMC free article] [PubMed] [Google Scholar]
- Molineux I. J., Friedman S., Gefter M. L. Purification and properties of the Escherichia coli deoxyribonucleic acid-unwinding protein. Effects on deoxyribonucleic acid synthesis in vitro. J Biol Chem. 1974 Oct 10;249(19):6090–6098. [PubMed] [Google Scholar]
- Prigodich R. V., Casas-Finet J., Williams K. R., Konigsberg W., Coleman J. E. 1H NMR (500 MHz) of gene 32 protein--oligonucleotide complexes. Biochemistry. 1984 Jan 31;23(3):522–529. doi: 10.1021/bi00298a019. [DOI] [PubMed] [Google Scholar]
- Prigodich R. V., Shamoo Y., Williams K. R., Chase J. W., Konigsberg W. H., Coleman J. E. 1H NMR (500 MHz) identification of aromatic residues of gene 32 protein involved in DNA binding by use of protein containing perdeuterated aromatic residues and by site-directed mutagenesis. Biochemistry. 1986 Jun 17;25(12):3666–3672. doi: 10.1021/bi00360a029. [DOI] [PubMed] [Google Scholar]
- Weiner J. H., Bertsch L. L., Kornberg A. The deoxyribonucleic acid unwinding protein of Escherichia coli. Properties and functions in replication. J Biol Chem. 1975 Mar 25;250(6):1972–1980. [PubMed] [Google Scholar]
- Williams K. R., Konigsberg W. Structural changes in the T4 gene 32 protein induced by DNA polynucleotides. J Biol Chem. 1978 Apr 10;253(7):2463–2470. [PubMed] [Google Scholar]
- Williams K. R., LoPresti M. B., Setoguchi M. Primary structure of the bacteriophage T4 DNA helix-destabilizing protein. J Biol Chem. 1981 Feb 25;256(4):1754–1762. [PubMed] [Google Scholar]
- Zorzopulos J., Kozloff L. M. Identification of T4D bacteriophage gene product 12 as the baseplate zinc metalloprotein. J Biol Chem. 1978 Aug 10;253(15):5543–5547. [PubMed] [Google Scholar]