Abstract
Sequence-specific interactions between aminoacyl-tRNA synthetases and their cognate tRNAs both ensure accurate RNA recognition and prevent the binding of noncognate substrates. Here we show for Escherichia coli glutaminyl-tRNA synthetase (GlnRS; EC 6.1.1.18) that the accuracy of tRNA recognition also determines the efficiency of cognate amino acid recognition. Steady-state kinetics revealed that interactions between tRNA identity nucleotides and their recognition sites in the enzyme modulate the amino acid affinity of GlnRS. Perturbation of any of the protein-RNA interactions through mutation of either component led to considerable changes in glutamine affinity with the most marked effects seen at the discriminator base, the 10:25 base pair, and the anticodon. Reexamination of the identity set of tRNA(Gln) in the light of these results indicates that its constituents can be differentiated based upon biochemical function and their contribution to the apparent Gibbs' free energy of tRNA binding. Interactions with the acceptor stem act as strong determinants of tRNA specificity, with the discriminator base positioning the 3' end. The 10:25 base pair and U35 are apparently the major binding sites to GlnRS, with G36 contributing both to binding and recognition. Furthermore, we show that E. coli tryptophanyl-tRNA synthetase also displays tRNA-dependent changes in tryptophan affinity when charging a noncognate tRNA. The ability of tRNA to optimize amino acid recognition reveals a novel mechanism for maintaining translational fidelity and also provides a strong basis for the coevolution of tRNAs and their cognate synthetases.
Full text
PDF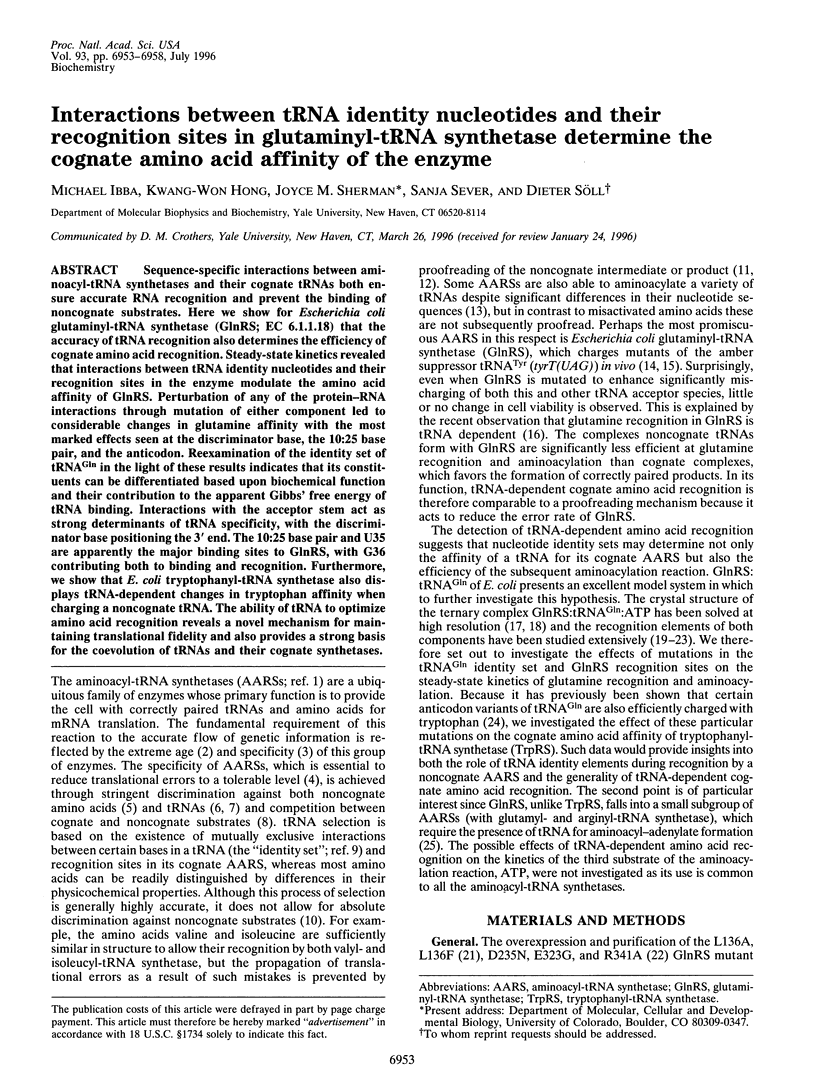
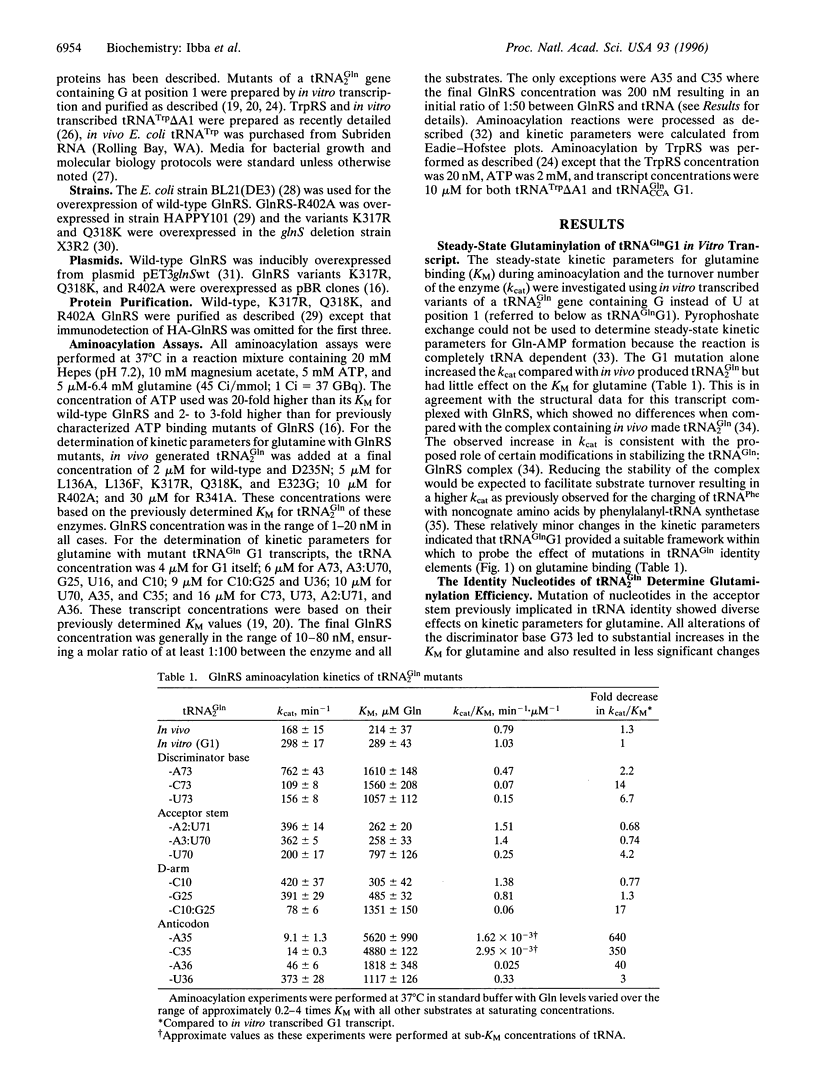
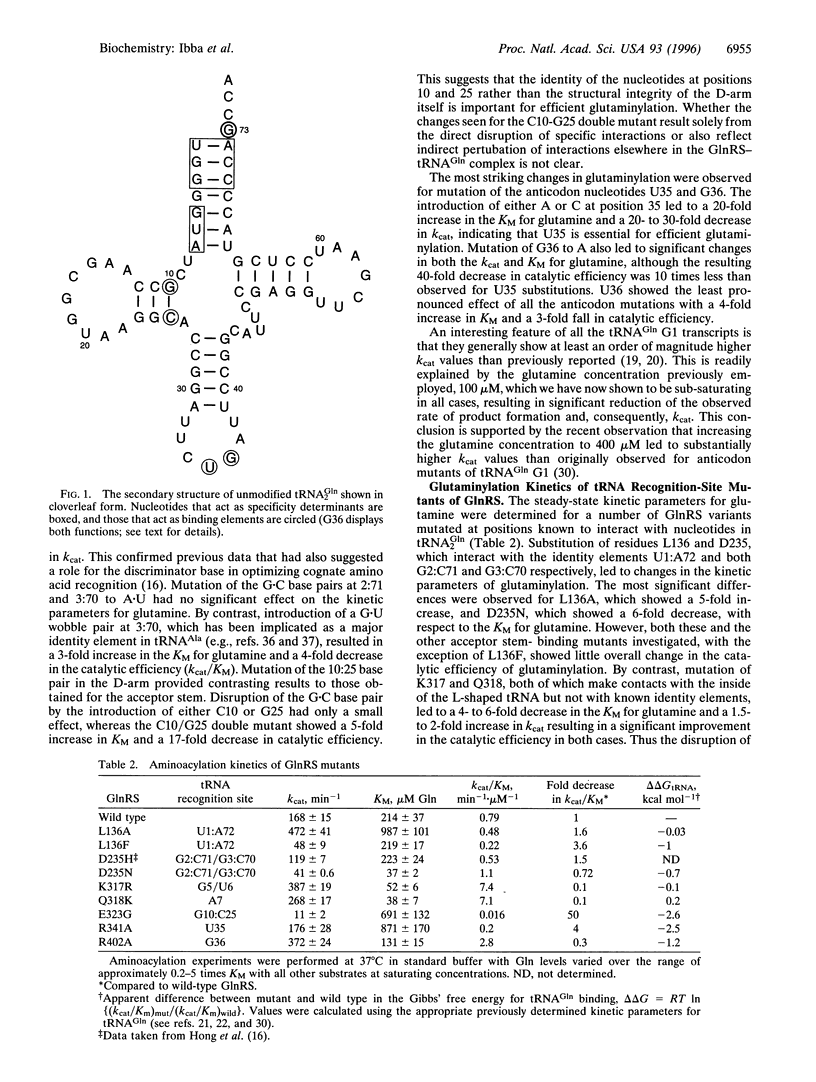
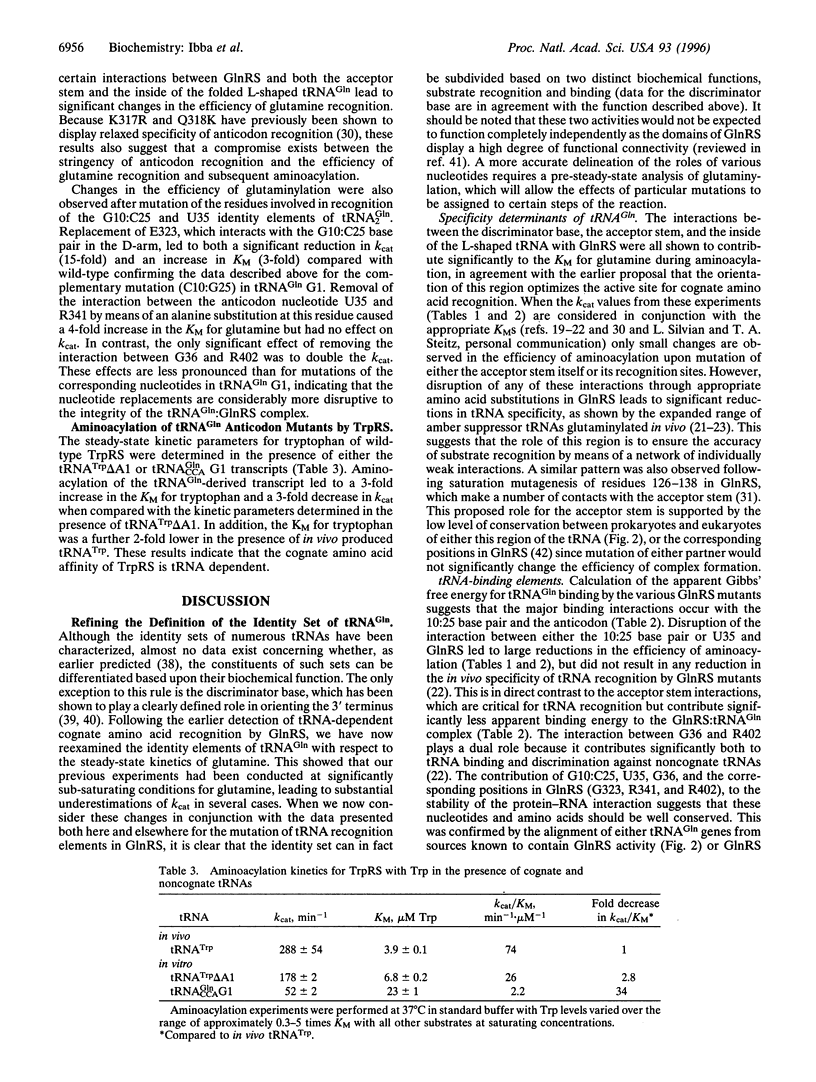
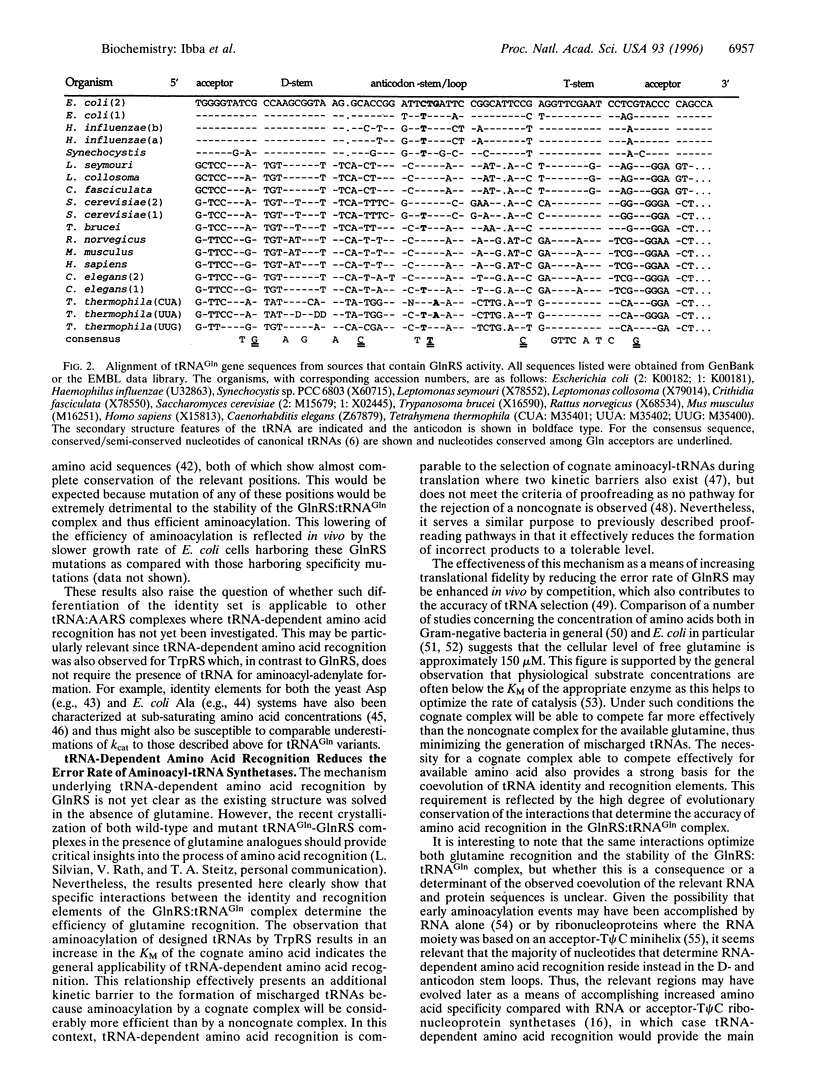
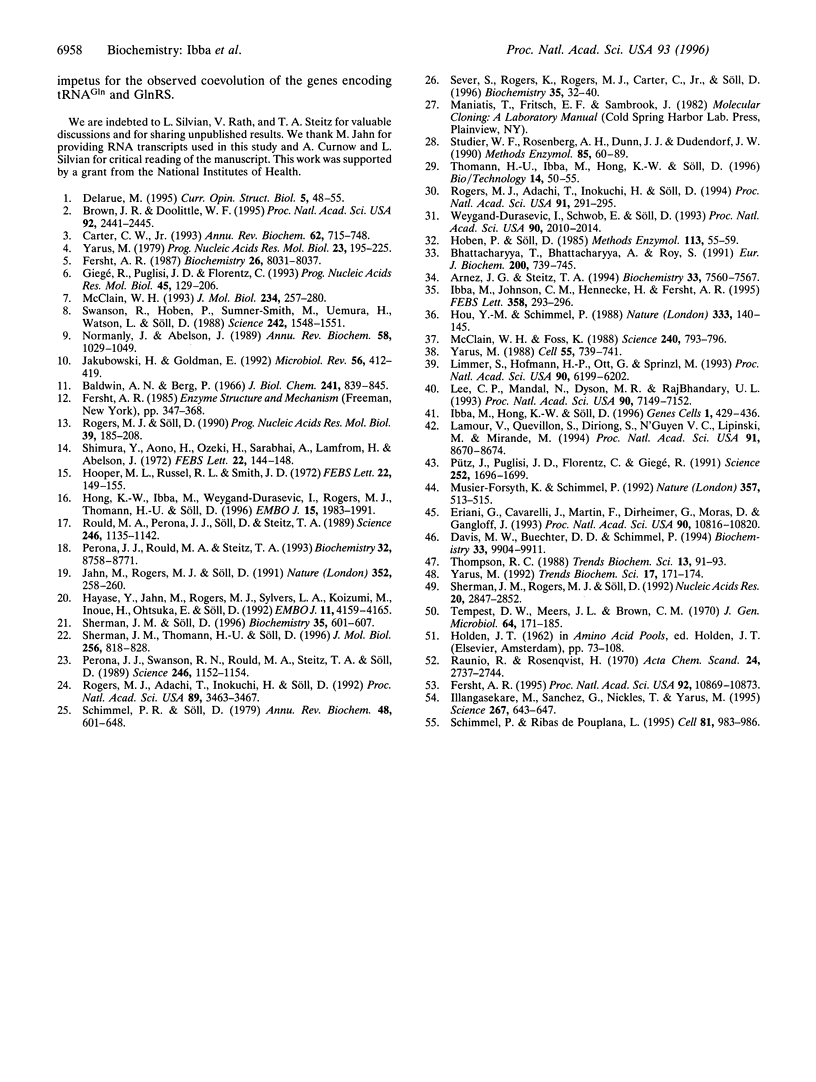
Selected References
These references are in PubMed. This may not be the complete list of references from this article.
- Arnez J. G., Steitz T. A. Crystal structure of unmodified tRNA(Gln) complexed with glutaminyl-tRNA synthetase and ATP suggests a possible role for pseudo-uridines in stabilization of RNA structure. Biochemistry. 1994 Jun 21;33(24):7560–7567. doi: 10.1021/bi00190a008. [DOI] [PubMed] [Google Scholar]
- Baldwin A. N., Berg P. Transfer ribonucleic acid-induced hydrolysis of valyladenylate bound to isoleucyl ribonucleic acid synthetase. J Biol Chem. 1966 Feb 25;241(4):839–845. [PubMed] [Google Scholar]
- Bhattacharyya T., Bhattacharyya A., Roy S. A fluorescence spectroscopic study of glutaminyl-tRNA synthetase from Escherichia coli and its implications for the enzyme mechanism. Eur J Biochem. 1991 Sep 15;200(3):739–745. doi: 10.1111/j.1432-1033.1991.tb16239.x. [DOI] [PubMed] [Google Scholar]
- Brown J. R., Doolittle W. F. Root of the universal tree of life based on ancient aminoacyl-tRNA synthetase gene duplications. Proc Natl Acad Sci U S A. 1995 Mar 28;92(7):2441–2445. doi: 10.1073/pnas.92.7.2441. [DOI] [PMC free article] [PubMed] [Google Scholar]
- Carter C. W., Jr Cognition, mechanism, and evolutionary relationships in aminoacyl-tRNA synthetases. Annu Rev Biochem. 1993;62:715–748. doi: 10.1146/annurev.bi.62.070193.003435. [DOI] [PubMed] [Google Scholar]
- Davis M. W., Buechter D. D., Schimmel P. Functional dissection of a predicted class-defining motif in a class II tRNA synthetase of unknown structure. Biochemistry. 1994 Aug 23;33(33):9904–9911. doi: 10.1021/bi00199a012. [DOI] [PubMed] [Google Scholar]
- Delarue M. Aminoacyl-tRNA synthetases. Curr Opin Struct Biol. 1995 Feb;5(1):48–55. doi: 10.1016/0959-440x(95)80008-o. [DOI] [PubMed] [Google Scholar]
- Eriani G., Cavarelli J., Martin F., Dirheimer G., Moras D., Gangloff J. Role of dimerization in yeast aspartyl-tRNA synthetase and importance of the class II invariant proline. Proc Natl Acad Sci U S A. 1993 Nov 15;90(22):10816–10820. doi: 10.1073/pnas.90.22.10816. [DOI] [PMC free article] [PubMed] [Google Scholar]
- Fersht A. R. Dissection of the structure and activity of the tyrosyl-tRNA synthetase by site-directed mutagenesis. Biochemistry. 1987 Dec 15;26(25):8031–8037. doi: 10.1021/bi00399a001. [DOI] [PubMed] [Google Scholar]
- Fersht A. R. Optimization of rates of protein folding: the nucleation-condensation mechanism and its implications. Proc Natl Acad Sci U S A. 1995 Nov 21;92(24):10869–10873. doi: 10.1073/pnas.92.24.10869. [DOI] [PMC free article] [PubMed] [Google Scholar]
- Giegé R., Puglisi J. D., Florentz C. tRNA structure and aminoacylation efficiency. Prog Nucleic Acid Res Mol Biol. 1993;45:129–206. doi: 10.1016/s0079-6603(08)60869-7. [DOI] [PubMed] [Google Scholar]
- Hayase Y., Jahn M., Rogers M. J., Sylvers L. A., Koizumi M., Inoue H., Ohtsuka E., Söll D. Recognition of bases in Escherichia coli tRNA(Gln) by glutaminyl-tRNA synthetase: a complete identity set. EMBO J. 1992 Nov;11(11):4159–4165. doi: 10.1002/j.1460-2075.1992.tb05509.x. [DOI] [PMC free article] [PubMed] [Google Scholar]
- Hoben P., Söll D. Glutaminyl-tRNA synthetase of Escherichia coli. Methods Enzymol. 1985;113:55–59. doi: 10.1016/s0076-6879(85)13011-9. [DOI] [PubMed] [Google Scholar]
- Hong K. W., Ibba M., Weygand-Durasevic I., Rogers M. J., Thomann H. U., Söll D. Transfer RNA-dependent cognate amino acid recognition by an aminoacyl-tRNA synthetase. EMBO J. 1996 Apr 15;15(8):1983–1991. [PMC free article] [PubMed] [Google Scholar]
- Hooper M. L., Russell R. L., Smith J. D. Mischarging in mutant tyrosine transfer RNAs. FEBS Lett. 1972 Apr 15;22(1):149–155. doi: 10.1016/0014-5793(72)80241-2. [DOI] [PubMed] [Google Scholar]
- Hou Y. M., Schimmel P. A simple structural feature is a major determinant of the identity of a transfer RNA. Nature. 1988 May 12;333(6169):140–145. doi: 10.1038/333140a0. [DOI] [PubMed] [Google Scholar]
- Ibba M., Johnson C. M., Hennecke H., Fersht A. R. Increased rates of tRNA charging through modification of the enzyme-aminoacyl-adenylate complex of phenylalanyl-tRNA synthetase. FEBS Lett. 1995 Jan 30;358(3):293–296. doi: 10.1016/0014-5793(94)01454-9. [DOI] [PubMed] [Google Scholar]
- Illangasekare M., Sanchez G., Nickles T., Yarus M. Aminoacyl-RNA synthesis catalyzed by an RNA. Science. 1995 Feb 3;267(5198):643–647. doi: 10.1126/science.7530860. [DOI] [PubMed] [Google Scholar]
- Jahn M., Rogers M. J., Söll D. Anticodon and acceptor stem nucleotides in tRNA(Gln) are major recognition elements for E. coli glutaminyl-tRNA synthetase. Nature. 1991 Jul 18;352(6332):258–260. doi: 10.1038/352258a0. [DOI] [PubMed] [Google Scholar]
- Jakubowski H., Goldman E. Editing of errors in selection of amino acids for protein synthesis. Microbiol Rev. 1992 Sep;56(3):412–429. doi: 10.1128/mr.56.3.412-429.1992. [DOI] [PMC free article] [PubMed] [Google Scholar]
- Kanamori A., Brown D. D. The analysis of complex developmental programmes: amphibian metamorphosis. Genes Cells. 1996 May;1(5):429–435. doi: 10.1046/j.1365-2443.1996.d01-251.x. [DOI] [PubMed] [Google Scholar]
- Lamour V., Quevillon S., Diriong S., N'Guyen V. C., Lipinski M., Mirande M. Evolution of the Glx-tRNA synthetase family: the glutaminyl enzyme as a case of horizontal gene transfer. Proc Natl Acad Sci U S A. 1994 Aug 30;91(18):8670–8674. doi: 10.1073/pnas.91.18.8670. [DOI] [PMC free article] [PubMed] [Google Scholar]
- Lee C. P., Mandal N., Dyson M. R., RajBhandary U. L. The discriminator base influences tRNA structure at the end of the acceptor stem and possibly its interaction with proteins. Proc Natl Acad Sci U S A. 1993 Aug 1;90(15):7149–7152. doi: 10.1073/pnas.90.15.7149. [DOI] [PMC free article] [PubMed] [Google Scholar]
- Limmer S., Hofmann H. P., Ott G., Sprinzl M. The 3'-terminal end (NCCA) of tRNA determines the structure and stability of the aminoacyl acceptor stem. Proc Natl Acad Sci U S A. 1993 Jul 1;90(13):6199–6202. doi: 10.1073/pnas.90.13.6199. [DOI] [PMC free article] [PubMed] [Google Scholar]
- McClain W. H., Foss K. Changing the identity of a tRNA by introducing a G-U wobble pair near the 3' acceptor end. Science. 1988 May 6;240(4853):793–796. doi: 10.1126/science.2452483. [DOI] [PubMed] [Google Scholar]
- McClain W. H. Rules that govern tRNA identity in protein synthesis. J Mol Biol. 1993 Nov 20;234(2):257–280. doi: 10.1006/jmbi.1993.1582. [DOI] [PubMed] [Google Scholar]
- Musier-Forsyth K., Schimmel P. Functional contacts of a transfer RNA synthetase with 2'-hydroxyl groups in the RNA minor groove. Nature. 1992 Jun 11;357(6378):513–515. doi: 10.1038/357513a0. [DOI] [PubMed] [Google Scholar]
- Normanly J., Abelson J. tRNA identity. Annu Rev Biochem. 1989;58:1029–1049. doi: 10.1146/annurev.bi.58.070189.005121. [DOI] [PubMed] [Google Scholar]
- Perona J. J., Rould M. A., Steitz T. A. Structural basis for transfer RNA aminoacylation by Escherichia coli glutaminyl-tRNA synthetase. Biochemistry. 1993 Aug 31;32(34):8758–8771. doi: 10.1021/bi00085a006. [DOI] [PubMed] [Google Scholar]
- Perona J. J., Swanson R. N., Rould M. A., Steitz T. A., Söll D. Structural basis for misaminoacylation by mutant E. coli glutaminyl-tRNA synthetase enzymes. Science. 1989 Dec 1;246(4934):1152–1154. doi: 10.1126/science.2686030. [DOI] [PubMed] [Google Scholar]
- Pütz J., Puglisi J. D., Florentz C., Giegé R. Identity elements for specific aminoacylation of yeast tRNA(Asp) by cognate aspartyl-tRNA synthetase. Science. 1991 Jun 21;252(5013):1696–1699. doi: 10.1126/science.2047878. [DOI] [PubMed] [Google Scholar]
- Raunio R., Rosenqvist H. Amino acid pool of Escherichia coli during the different phases of growth. Acta Chem Scand. 1970;24(8):2737–2744. doi: 10.3891/acta.chem.scand.24-2737. [DOI] [PubMed] [Google Scholar]
- Rogers M. J., Adachi T., Inokuchi H., Söll D. Functional communication in the recognition of tRNA by Escherichia coli glutaminyl-tRNA synthetase. Proc Natl Acad Sci U S A. 1994 Jan 4;91(1):291–295. doi: 10.1073/pnas.91.1.291. [DOI] [PMC free article] [PubMed] [Google Scholar]
- Rogers M. J., Adachi T., Inokuchi H., Söll D. Switching tRNA(Gln) identity from glutamine to tryptophan. Proc Natl Acad Sci U S A. 1992 Apr 15;89(8):3463–3467. doi: 10.1073/pnas.89.8.3463. [DOI] [PMC free article] [PubMed] [Google Scholar]
- Rogers M. J., Söll D. Inaccuracy and the recognition of tRNA. Prog Nucleic Acid Res Mol Biol. 1990;39:185–208. doi: 10.1016/s0079-6603(08)60627-3. [DOI] [PubMed] [Google Scholar]
- Rould M. A., Perona J. J., Söll D., Steitz T. A. Structure of E. coli glutaminyl-tRNA synthetase complexed with tRNA(Gln) and ATP at 2.8 A resolution. Science. 1989 Dec 1;246(4934):1135–1142. doi: 10.1126/science.2479982. [DOI] [PubMed] [Google Scholar]
- Schimmel P. R., Söll D. Aminoacyl-tRNA synthetases: general features and recognition of transfer RNAs. Annu Rev Biochem. 1979;48:601–648. doi: 10.1146/annurev.bi.48.070179.003125. [DOI] [PubMed] [Google Scholar]
- Schimmel P., Ribas de Pouplana L. Transfer RNA: from minihelix to genetic code. Cell. 1995 Jun 30;81(7):983–986. doi: 10.1016/s0092-8674(05)80002-9. [DOI] [PubMed] [Google Scholar]
- Sever S., Rogers K., Rogers M. J., Carter C., Jr, Söll D. Escherichia coli tryptophanyl-tRNA synthetase mutants selected for tryptophan auxotrophy implicate the dimer interface in optimizing amino acid binding. Biochemistry. 1996 Jan 9;35(1):32–40. doi: 10.1021/bi952103d. [DOI] [PubMed] [Google Scholar]
- Sherman J. M., Rogers M. J., Söll D. Competition of aminoacyl-tRNA synthetases for tRNA ensures the accuracy of aminoacylation. Nucleic Acids Res. 1992 Jun 11;20(11):2847–2852. doi: 10.1093/nar/20.11.2847. [DOI] [PMC free article] [PubMed] [Google Scholar]
- Sherman J. M., Söll D. Aminoacyl-tRNA synthetases optimize both cognate tRNA recognition and discrimination against noncognate tRNAs. Biochemistry. 1996 Jan 16;35(2):601–607. doi: 10.1021/bi951602b. [DOI] [PubMed] [Google Scholar]
- Sherman J. M., Thomann H. U., Söll D. Functional connectivity between tRNA binding domains in glutaminyl-tRNA synthetase. J Mol Biol. 1996 Mar 15;256(5):818–828. doi: 10.1006/jmbi.1996.0128. [DOI] [PubMed] [Google Scholar]
- Shimura Y., Aono H., Ozeki H., Sarabhai A., Lamfrom H., Abelson J. Mutant tyrosine tRNA of altered amino acid specificity. FEBS Lett. 1972 Apr 15;22(1):144–148. doi: 10.1016/0014-5793(72)80240-0. [DOI] [PubMed] [Google Scholar]
- Studier F. W., Rosenberg A. H., Dunn J. J., Dubendorff J. W. Use of T7 RNA polymerase to direct expression of cloned genes. Methods Enzymol. 1990;185:60–89. doi: 10.1016/0076-6879(90)85008-c. [DOI] [PubMed] [Google Scholar]
- Swanson R., Hoben P., Sumner-Smith M., Uemura H., Watson L., Söll D. Accuracy of in vivo aminoacylation requires proper balance of tRNA and aminoacyl-tRNA synthetase. Science. 1988 Dec 16;242(4885):1548–1551. doi: 10.1126/science.3144042. [DOI] [PubMed] [Google Scholar]
- Tempest D. W., Meers J. L., Brown C. M. Influence of environment on the content and composition of microbial free amino acid pools. J Gen Microbiol. 1970 Dec;64(2):171–185. doi: 10.1099/00221287-64-2-171. [DOI] [PubMed] [Google Scholar]
- Thomann H. U., Ibba M., Hong K. W., Söll D. Homologous expression and purification of mutants of an essential protein by reverse epitope-tagging. Biotechnology (N Y) 1996 Jan;14(1):50–55. doi: 10.1038/nbt0196-50. [DOI] [PubMed] [Google Scholar]
- Thompson R. C. EFTu provides an internal kinetic standard for translational accuracy. Trends Biochem Sci. 1988 Mar;13(3):91–93. doi: 10.1016/0968-0004(88)90047-3. [DOI] [PubMed] [Google Scholar]
- Weygand-Durasević I., Schwob E., Söll D. Acceptor end binding domain interactions ensure correct aminoacylation of transfer RNA. Proc Natl Acad Sci U S A. 1993 Mar 1;90(5):2010–2014. doi: 10.1073/pnas.90.5.2010. [DOI] [PMC free article] [PubMed] [Google Scholar]
- Yarus M. Proofreading, NTPases and translation: successful increase in specificity. Trends Biochem Sci. 1992 May;17(5):171–174. doi: 10.1016/0968-0004(92)90257-a. [DOI] [PubMed] [Google Scholar]
- Yarus M. The accuracy of translation. Prog Nucleic Acid Res Mol Biol. 1979;23:195–225. doi: 10.1016/s0079-6603(08)60134-8. [DOI] [PubMed] [Google Scholar]
- Yarus M. tRNA identity: a hair of the dogma that bit us. Cell. 1988 Dec 2;55(5):739–741. doi: 10.1016/0092-8674(88)90127-4. [DOI] [PubMed] [Google Scholar]