Abstract
A generalization of the Szabo-Karplus statistical mechanical model for hemoglobin cooperativity is formulated. The model fits the available thermodynamic and spectroscopic data with assumptions that are consistent with structural results and empirical energy function calculations. It provides a mechanism of hemoglobin cooperativity that is a generalization of the proposals of Monod, Wyman, and Changeux and of Perutz. The role of nonsalt-bridge related sources of constraints on ligand affinity and the mode of salt-bridge coupling to tertiary-quaternary structural changes are examined within the framework of the model. Analysis of proton release data for a range of pH values indicates that a pH-independent part of cooperativity must be present. The pH dependence of the first and last Adair constants point to partial linkage of salt bridges to ligation in the deoxy state and to a destabilized intra-beta-chain salt bridge in the unliganded oxy state.
Full text
PDF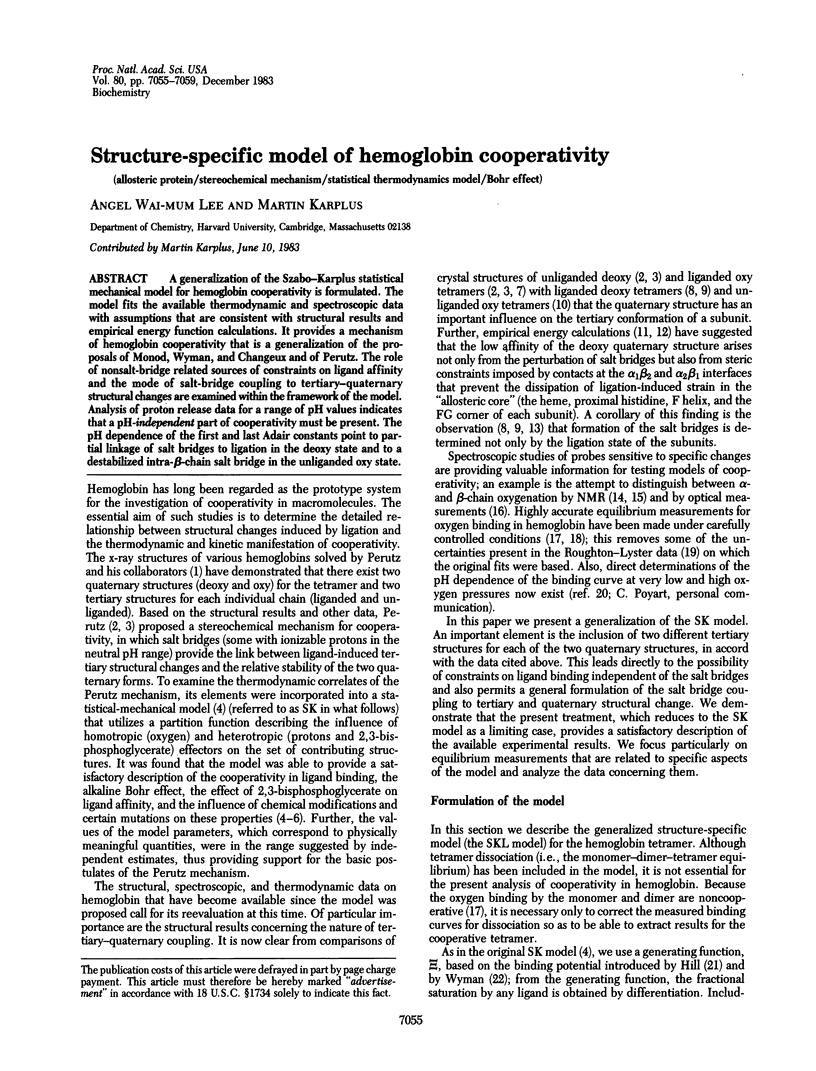
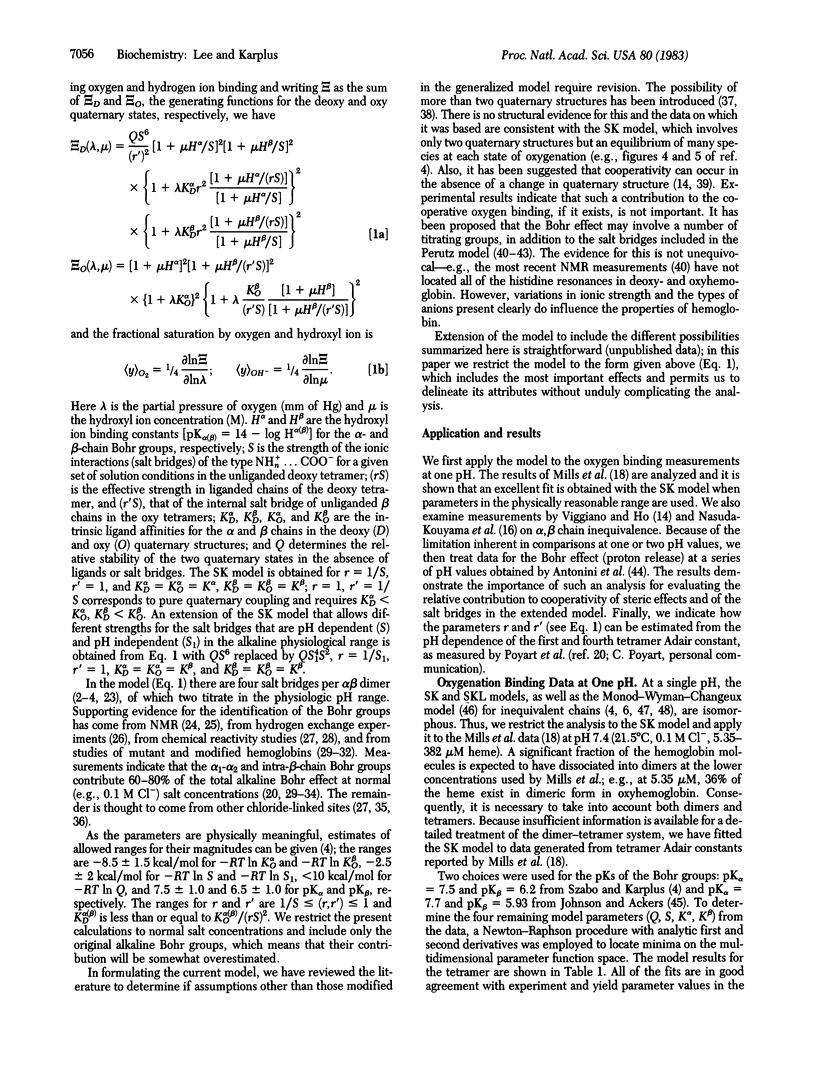
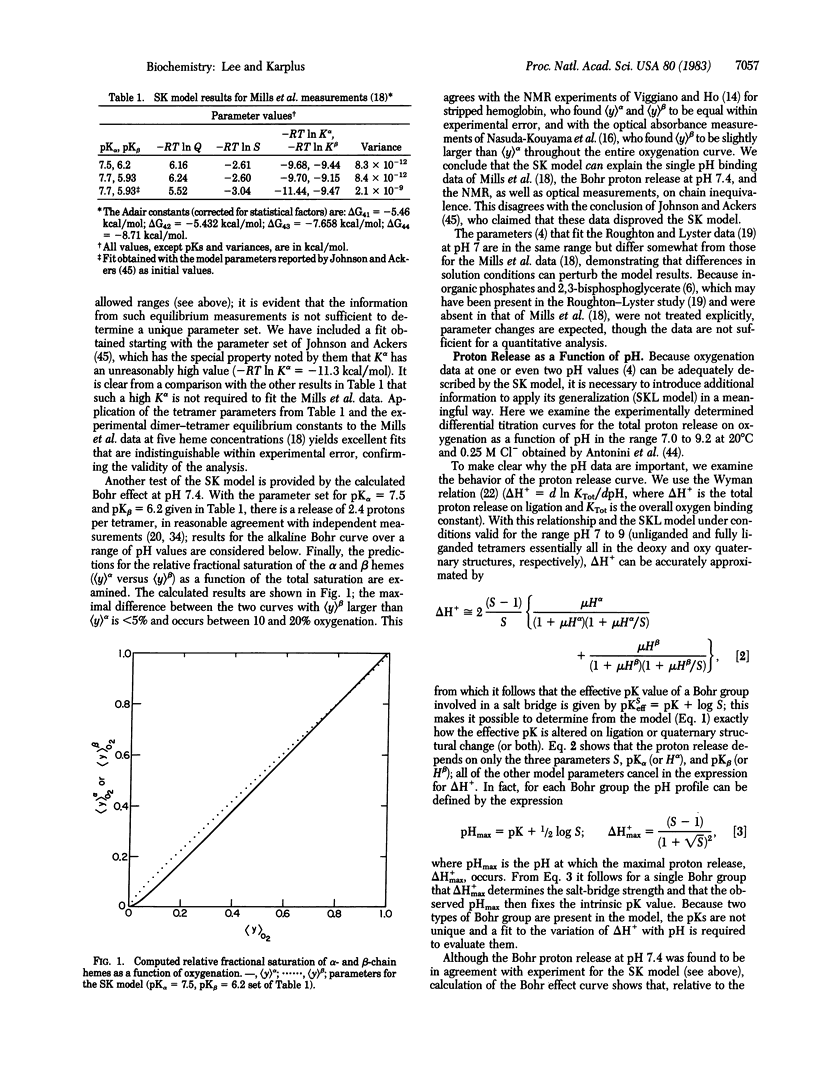
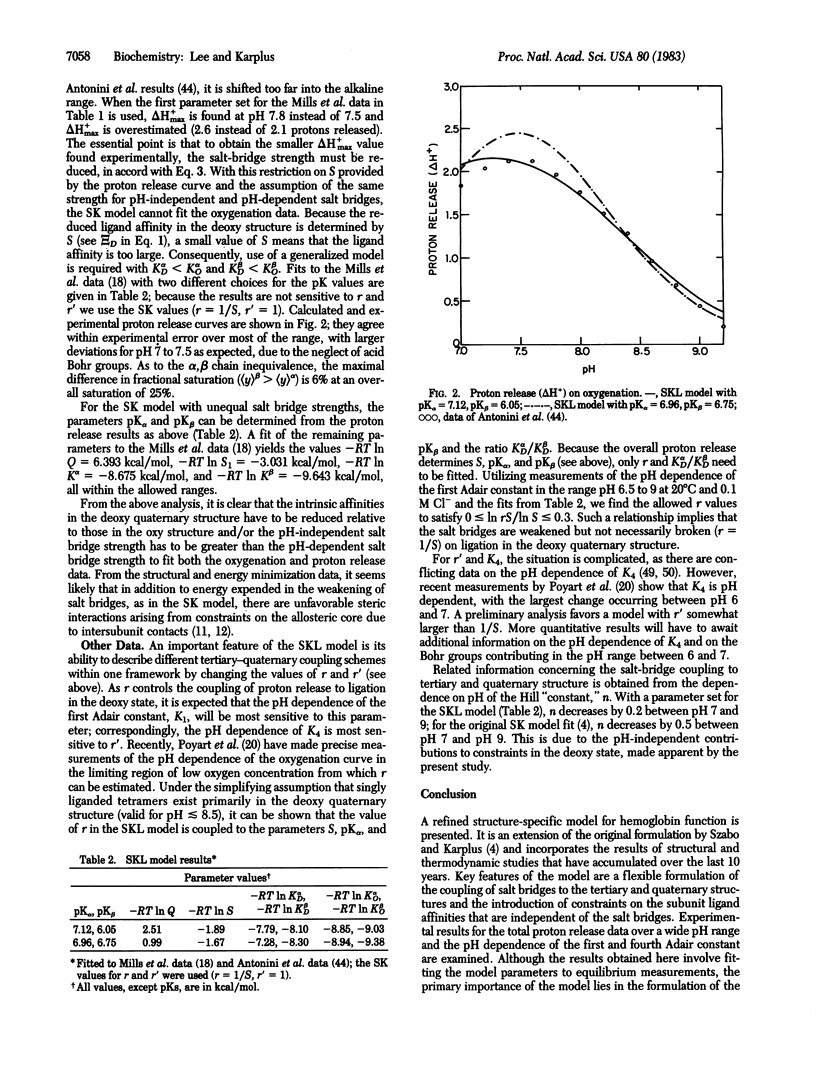
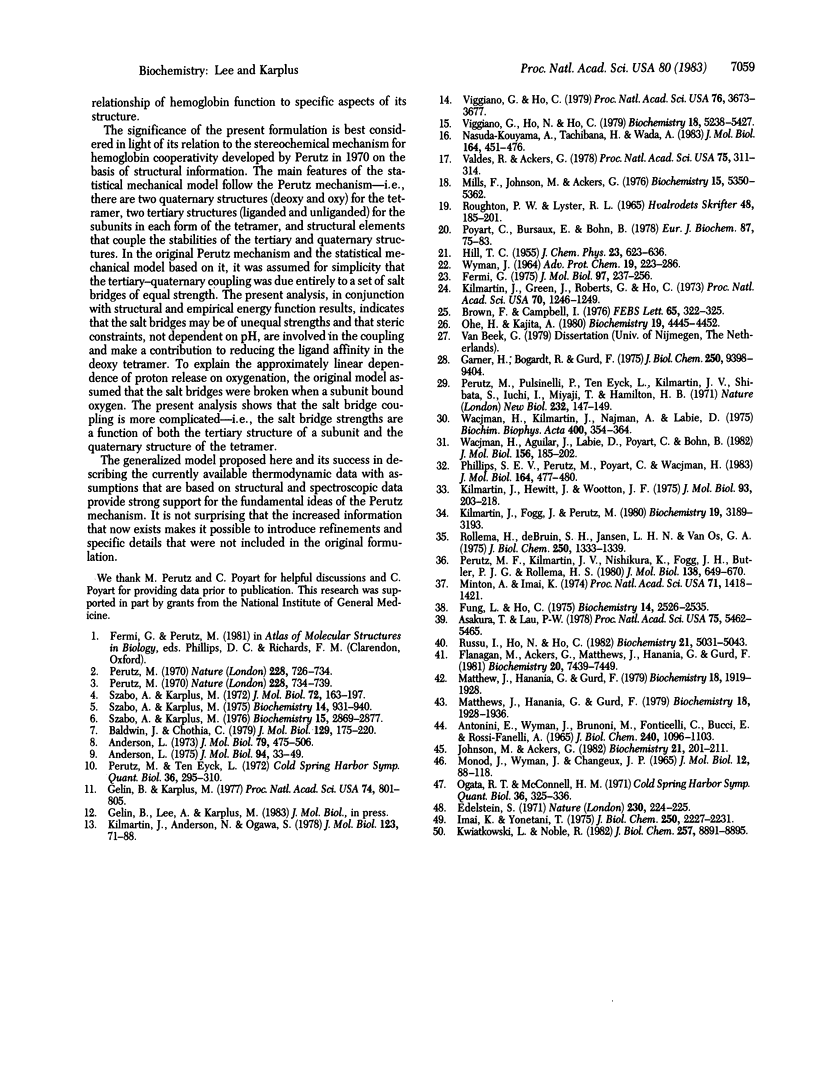
Selected References
These references are in PubMed. This may not be the complete list of references from this article.
- ANTONINI E., WYMAN J., BRUNORI M., FRONTICELLI C., BUCCI E., ROSSI-FANELLI A. STUDIES ON THE RELATIONS BETWEEN MOLECULAR AND FUNCTIONAL PROPERTIES OF HEMOGLOBIN. V. THE INFLUENCE OF TEMPERATURE ON THE BOHR EFFECT IN HUMAN AND IN HORSE HEMOGLOBIN. J Biol Chem. 1965 Mar;240:1096–1103. [PubMed] [Google Scholar]
- Anderson L. Intermediate structure of normal human haemoglobin: methaemoglobin in the deoxy quaternary conformation. J Mol Biol. 1973 Sep 25;79(3):495–506. doi: 10.1016/0022-2836(73)90401-4. [DOI] [PubMed] [Google Scholar]
- Anderson L. Structures of deoxy and carbonmonoxy haemoglobin Kansas in the deoxy quaternary conformation. J Mol Biol. 1975 May 5;94(1):33–49. doi: 10.1016/0022-2836(75)90403-9. [DOI] [PubMed] [Google Scholar]
- Asakura T., Lau P. W. Sequence of oxygen binding by hemoglobin. Proc Natl Acad Sci U S A. 1978 Nov;75(11):5462–5465. doi: 10.1073/pnas.75.11.5462. [DOI] [PMC free article] [PubMed] [Google Scholar]
- Baldwin J., Chothia C. Haemoglobin: the structural changes related to ligand binding and its allosteric mechanism. J Mol Biol. 1979 Apr 5;129(2):175–220. doi: 10.1016/0022-2836(79)90277-8. [DOI] [PubMed] [Google Scholar]
- Brown F. F., Campbell I. D. Cross correlation of titrating histidines in oxy- and deoxyhaemoglobin; an NMR study. FEBS Lett. 1976 Jun 15;65(3):322–326. doi: 10.1016/0014-5793(76)80139-1. [DOI] [PubMed] [Google Scholar]
- Edelstein S. J. Extensions of the allosteric model for haemoglobin. Nature. 1971 Mar 26;230(5291):224–227. doi: 10.1038/230224a0. [DOI] [PubMed] [Google Scholar]
- Fermi G. Three-dimensional fourier synthesis of human deoxyhaemoglobin at 2-5 A resolution: refinement of the atomic model. J Mol Biol. 1975 Sep 15;97(2):237–256. doi: 10.1016/s0022-2836(75)80037-4. [DOI] [PubMed] [Google Scholar]
- Flanagan M. A., Ackers G. K., Matthew J. B., Hanania G. I., Gurd F. R. Electrostatic contributions to the energetics of dimer-tetramer assembly in human hemoglobin: pH dependence and effect of specifically bound chloride ions. Biochemistry. 1981 Dec 22;20(26):7439–7449. doi: 10.1021/bi00529a018. [DOI] [PubMed] [Google Scholar]
- Fung L. W., Ho C. A proton nuclear magnetic resonance study of the quaternary structure of human homoglobins in water. Biochemistry. 1975 Jun 3;14(11):2526–2535. doi: 10.1021/bi00682a036. [DOI] [PubMed] [Google Scholar]
- Gelin B. R., Karplus M. Mechanism of tertiary structural change in hemoglobin. Proc Natl Acad Sci U S A. 1977 Mar;74(3):801–805. doi: 10.1073/pnas.74.3.801. [DOI] [PMC free article] [PubMed] [Google Scholar]
- Imai K., Yonetani T. PH dependence of the Adair constants of human hemoglobin. Nonuniform contribution of successive oxygen bindings to the alkaline Bohr effect. J Biol Chem. 1975 Mar 25;250(6):2227–2231. [PubMed] [Google Scholar]
- Johnson M. L., Ackers G. K. Thermodynamic analysis of human hemoglobins in terms of the Perutz mechanism: extensions of the Szabo--Karplus model to include subunit assembly. Biochemistry. 1982 Jan 19;21(2):201–211. doi: 10.1021/bi00531a001. [DOI] [PubMed] [Google Scholar]
- Kilmartin J. V., Anderson N. L. Response of the Bohr group salt bridges to ligation of the T state of haemoglobin Kansas. J Mol Biol. 1978 Jul 25;123(1):71–87. doi: 10.1016/0022-2836(78)90377-7. [DOI] [PubMed] [Google Scholar]
- Kilmartin J. V., Breen J. J., Roberts G. C., Ho C. Direct measurement of the pK values of an alkaline Bohr group in human hemoglobin. Proc Natl Acad Sci U S A. 1973 Apr;70(4):1246–1249. doi: 10.1073/pnas.70.4.1246. [DOI] [PMC free article] [PubMed] [Google Scholar]
- Kilmartin J. V., Fogg J. H., Perutz M. F. Role of C-terminal histidine in the alkaline Bohr effect of human hemoglobin. Biochemistry. 1980 Jul 8;19(14):3189–3183. doi: 10.1021/bi00555a013. [DOI] [PubMed] [Google Scholar]
- Kilmartin J. V., Hewitt J. A., Wootton J. F. Alteration of functional properties associated with the change in quaternary structure in unliganded haemoglobin. J Mol Biol. 1975 Apr 5;93(2):203–218. doi: 10.1016/0022-2836(75)90128-x. [DOI] [PubMed] [Google Scholar]
- Kwiatkowski L. D., Noble R. W. The contribution of histidine (HC3) (146 beta) to the R state Bohr effect of human hemoglobin. J Biol Chem. 1982 Aug 10;257(15):8891–8895. [PubMed] [Google Scholar]
- MONOD J., WYMAN J., CHANGEUX J. P. ON THE NATURE OF ALLOSTERIC TRANSITIONS: A PLAUSIBLE MODEL. J Mol Biol. 1965 May;12:88–118. doi: 10.1016/s0022-2836(65)80285-6. [DOI] [PubMed] [Google Scholar]
- Matthew J. B., Hanania G. I., Gurd F. R. Electrostatic effects in hemoglobin: Bohr effect and ionic strength dependence of individual groups. Biochemistry. 1979 May 15;18(10):1928–1936. doi: 10.1021/bi00577a012. [DOI] [PubMed] [Google Scholar]
- Matthew J. B., Hanania G. I., Gurd F. R. Electrostatic effects in hemoglobin: hydrogen ion equilibria in human deoxy- and oxyhemoglobin A. Biochemistry. 1979 May 15;18(10):1919–1928. doi: 10.1021/bi00577a011. [DOI] [PubMed] [Google Scholar]
- Mills F. C., Johnson M. L., Ackers G. K. Oxygenation-linked subunit interactions in human hemoglobin: experimental studies on the concentration dependence of oxygenation curves. Biochemistry. 1976 Nov 30;15(24):5350–5362. doi: 10.1021/bi00669a023. [DOI] [PubMed] [Google Scholar]
- Minton A. P., Imai K. The three-state model: a minimal allosteric description of homotropic and heterotropic effects in the binding of ligands to hemoglobin. Proc Natl Acad Sci U S A. 1974 Apr;71(4):1418–1421. doi: 10.1073/pnas.71.4.1418. [DOI] [PMC free article] [PubMed] [Google Scholar]
- Nasuda-Kouyama A., Tachibana H., Wada A. Preference of oxygenation between alpha and beta subunits of haemoglobin. Results of multidimensional spectroscopic observation. J Mol Biol. 1983 Mar 5;164(3):451–476. doi: 10.1016/0022-2836(83)90061-x. [DOI] [PubMed] [Google Scholar]
- Ogata R. T., McConnell H. M. The binding of a spin-labeled triphosphate to hemoglobin. Cold Spring Harb Symp Quant Biol. 1972;36:325–336. doi: 10.1101/sqb.1972.036.01.043. [DOI] [PubMed] [Google Scholar]
- Perutz M. F., Kilmartin J. V., Nishikura K., Fogg J. H., Butler P. J., Rollema H. S. Identification of residues contributing to the Bohr effect of human haemoglobin. J Mol Biol. 1980 Apr 15;138(3):649–668. doi: 10.1016/s0022-2836(80)80022-2. [DOI] [PubMed] [Google Scholar]
- Perutz M. F., Pulsinelli P., Eyck L. T., Kilmartin J. V., Shibata S., Iuchi I., Miyaji T., Hamilton H. B. Haemoglobin Hiroshima and the mechanism of the alkaline Bohr effect. Nat New Biol. 1971 Aug 4;232(31):147–149. doi: 10.1038/newbio232147a0. [DOI] [PubMed] [Google Scholar]
- Perutz M. F. Stereochemistry of cooperative effects in haemoglobin. Nature. 1970 Nov 21;228(5273):726–739. doi: 10.1038/228726a0. [DOI] [PubMed] [Google Scholar]
- Perutz M. F., TenEyck L. F. Stereochemistry of cooperative effects in hemoglobin. Cold Spring Harb Symp Quant Biol. 1972;36:295–310. doi: 10.1101/sqb.1972.036.01.040. [DOI] [PubMed] [Google Scholar]
- Phillips S. E., Perutz M. F., Poyart C., Wajcman H. Structure and function of haemoglobin Barcelona Asp FG1(94) beta leads to His. J Mol Biol. 1983 Mar 5;164(3):477–480. doi: 10.1016/0022-2836(83)90062-1. [DOI] [PubMed] [Google Scholar]
- Poyart C. F., Bursaux E., Bohn B. An estimation of the first binding constant of O2 to human hemoglobin A. Eur J Biochem. 1978 Jun 1;87(1):75–83. doi: 10.1111/j.1432-1033.1978.tb12353.x. [DOI] [PubMed] [Google Scholar]
- Rollema H. S., de Bruin S. H., Janssen L. H., van Os G. A. The effect of potassium chloride on the Bohr effect of human hemoglobin. J Biol Chem. 1975 Feb 25;250(4):1333–1339. [PubMed] [Google Scholar]
- Russu I. M., Ho N. T., Ho C. A proton nuclear magnetic resonance investigation of histidyl residues in human normal adult hemoglobin. Biochemistry. 1982 Sep 28;21(20):5031–5043. doi: 10.1021/bi00263a029. [DOI] [PubMed] [Google Scholar]
- Szabo A., Karplus M. A mathematical model for structure-function relations in hemoglobin. J Mol Biol. 1972 Dec 14;72(1):163–197. doi: 10.1016/0022-2836(72)90077-0. [DOI] [PubMed] [Google Scholar]
- Szabo A., Karplus M. Analysis of cooperativity in hemoglobin. Valency hybrids, oxidation, and methemoglobin replacement reactions. Biochemistry. 1975 Mar 11;14(5):931–940. doi: 10.1021/bi00676a009. [DOI] [PubMed] [Google Scholar]
- Szabo A., Karplus M. Analysis of the interaction of organic phosphates with hemoglobin. Biochemistry. 1976 Jun 29;15(13):2869–2877. doi: 10.1021/bi00658a026. [DOI] [PubMed] [Google Scholar]
- Valdes R., Jr, Ackers G. K. Self-association of hemoglobin betaSH chains is linked to oxygenation. Proc Natl Acad Sci U S A. 1978 Jan;75(1):311–314. doi: 10.1073/pnas.75.1.311. [DOI] [PMC free article] [PubMed] [Google Scholar]
- Viggiano G., Ho C. Proton nuclear magnetic resonance investigation of structural changes associated with cooperative oxygenation of human adult hemoglobin. Proc Natl Acad Sci U S A. 1979 Aug;76(8):3673–3677. doi: 10.1073/pnas.76.8.3673. [DOI] [PMC free article] [PubMed] [Google Scholar]
- Viggiano G., Ho N. T., Ho C. Proton nuclear magnetic resonance and biochemical studies of oxygenation of human adult hemoglobin in deuterium oxide. Biochemistry. 1979 Nov 13;18(23):5238–5247. doi: 10.1021/bi00590a031. [DOI] [PubMed] [Google Scholar]
- WYMAN J., Jr LINKED FUNCTIONS AND RECIPROCAL EFFECTS IN HEMOGLOBIN: A SECOND LOOK. Adv Protein Chem. 1964;19:223–286. doi: 10.1016/s0065-3233(08)60190-4. [DOI] [PubMed] [Google Scholar]
- Wajcman H., Aguilar i Bascompte J. L., Labie D., Poyart C., Bohn B. Structural and functional studies of hemoglobin Barcelona (alpha 2 beta 2 94 Asp (FG1) replaced by His). Consequences of altering an important intrachain salt bridge involved in the alkaline Bohr effect. J Mol Biol. 1982 Mar 25;156(1):185–202. doi: 10.1016/0022-2836(82)90466-1. [DOI] [PubMed] [Google Scholar]
- Wajcman H., Kilmartin J. V., Najman A., Labie D. Hemoglobin Cochin-Port-Royal: consequences of the replacement of the beta chain C-terminal by an arginine. Biochim Biophys Acta. 1975 Aug 19;400(2):354–364. doi: 10.1016/0005-2795(75)90191-9. [DOI] [PubMed] [Google Scholar]