Abstract
Glial fibrillary acidic protein (GFAP) is a member of the family of intermediate filament structural proteins and is found predominantly in astrocytes of the central nervous system (CNS). To assess the function of GFAP, we created GFAP-null mice using gene targeting in embryonic stem cells. The GFAP-null mice have normal development and fertility, and show no gross alterations in behavior or CNS morphology. Astrocytes are present in the CNS of the mutant mice, but contain a severely reduced number of intermediate filaments. Since astrocyte processes contact synapses and may modulate synaptic function, we examined whether the GFAP-null mice were altered in long-term potentiation in the CA1 region of the hippocampus. The GFAP-null mice displayed enhanced long-term potentiation of both population spike amplitude and excitatory post-synaptic potential slope compared to control mice. These data suggest that GFAP is important for astrocyte-neuronal interactions, and that astrocyte processes play a vital role in modulating synaptic efficacy in the CNS. These mice therefore represent a direct demonstration that a primary defect in astrocytes influences neuronal physiology.
Full text
PDF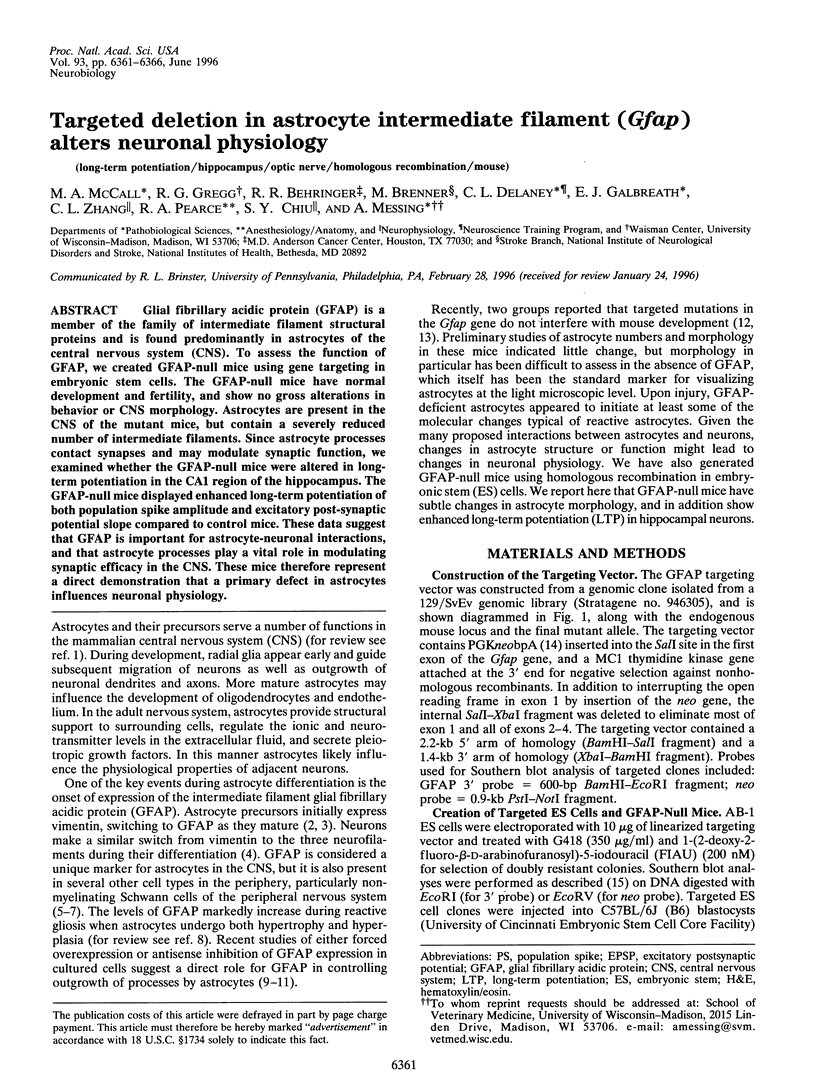
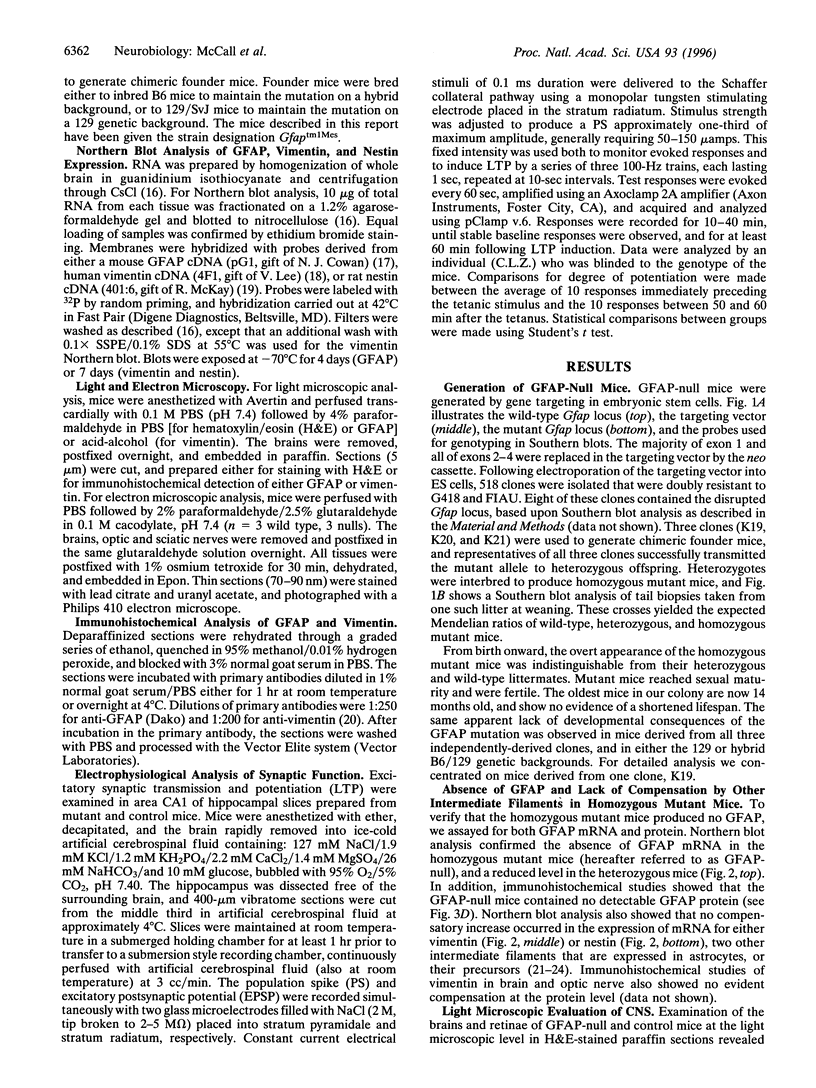
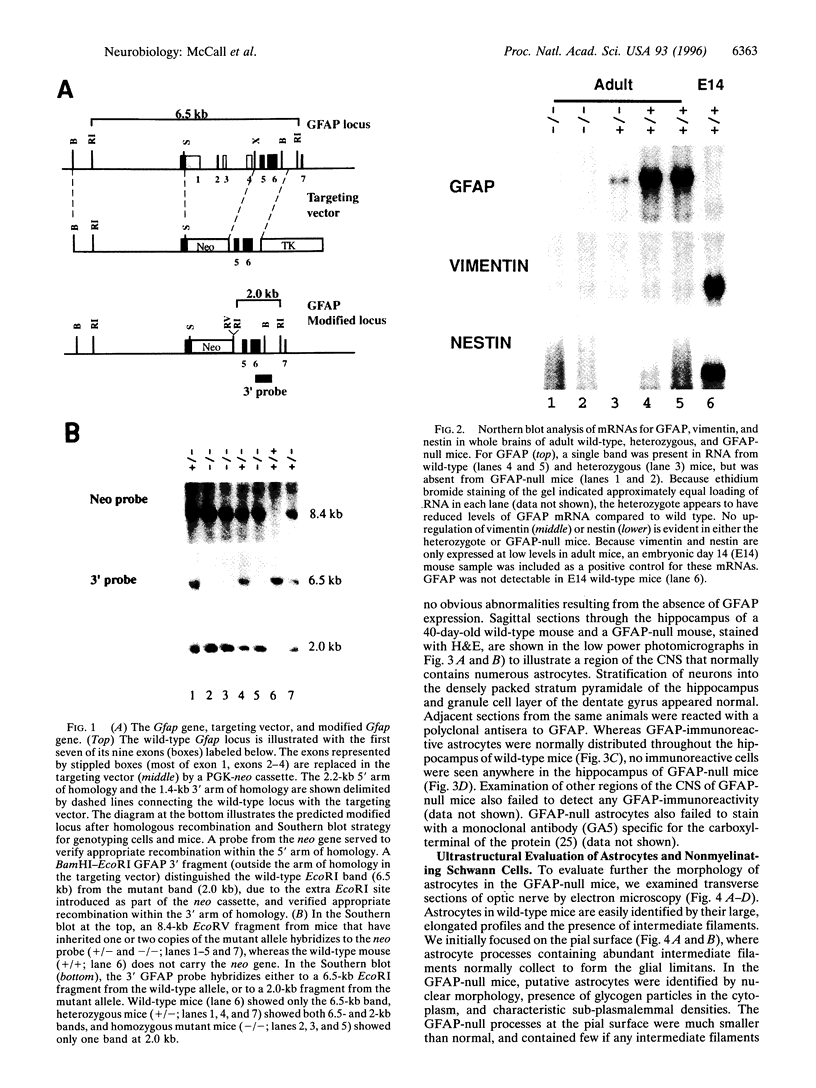
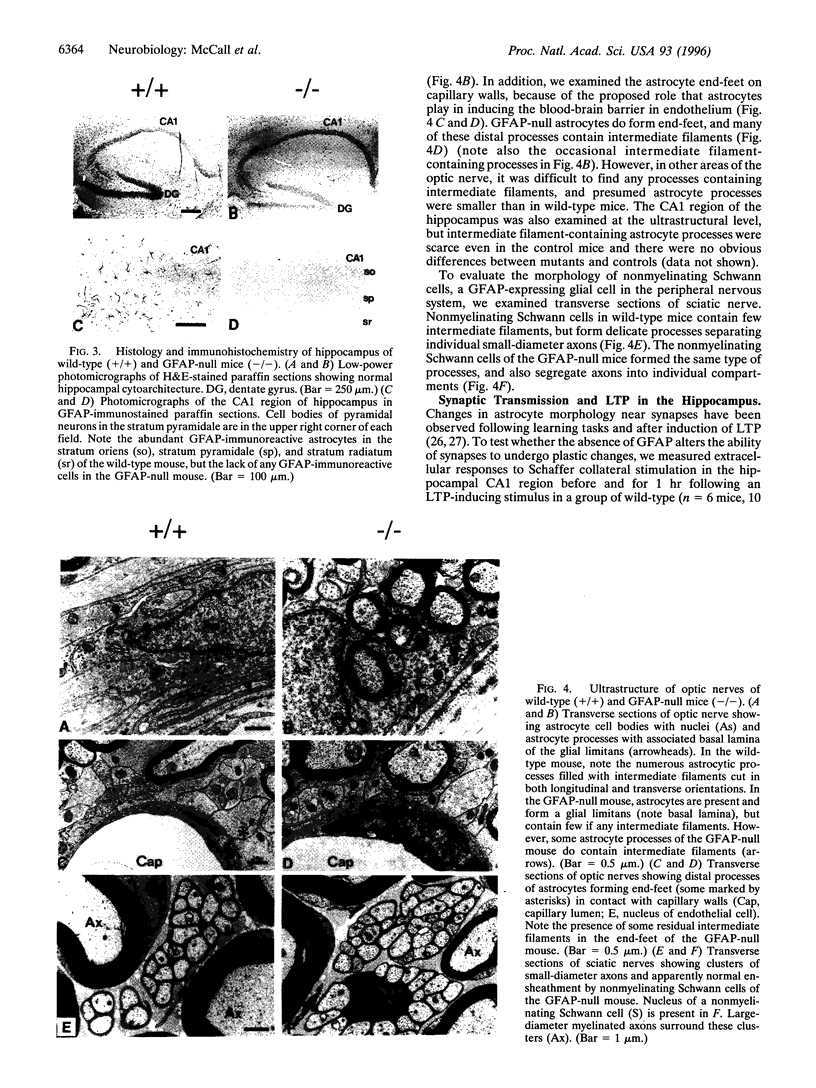
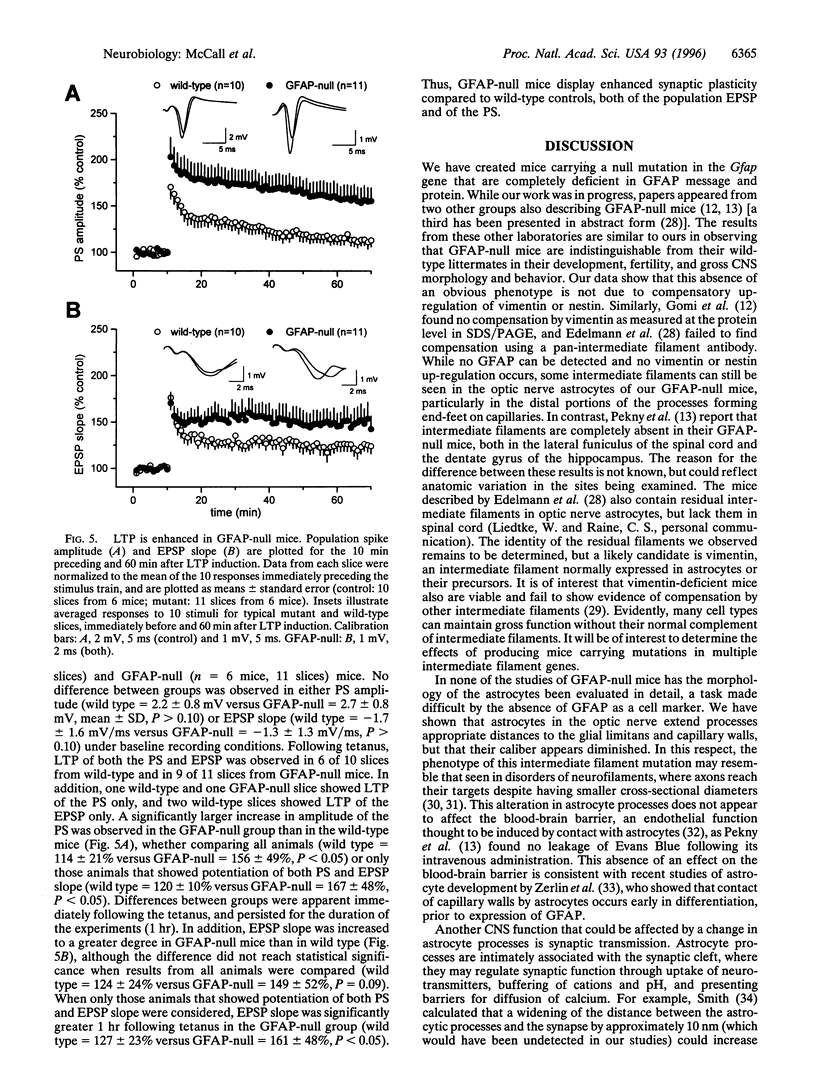
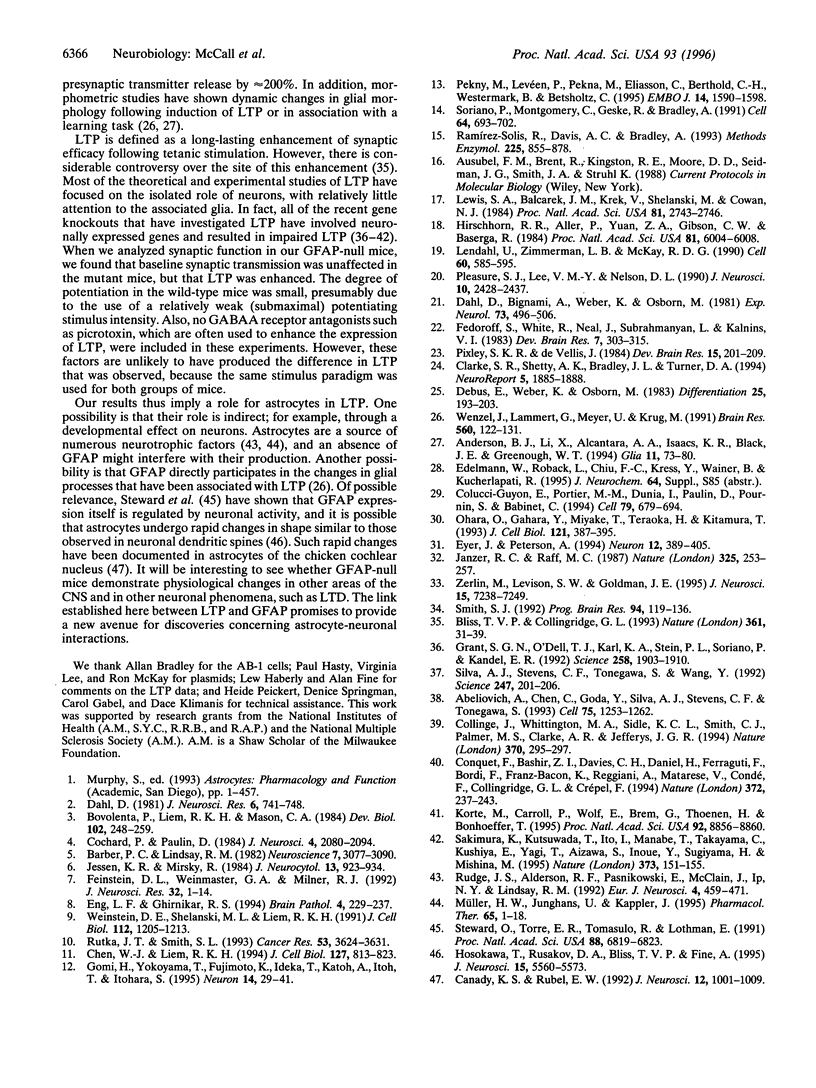
Images in this article
Selected References
These references are in PubMed. This may not be the complete list of references from this article.
- Abeliovich A., Chen C., Goda Y., Silva A. J., Stevens C. F., Tonegawa S. Modified hippocampal long-term potentiation in PKC gamma-mutant mice. Cell. 1993 Dec 31;75(7):1253–1262. doi: 10.1016/0092-8674(93)90613-u. [DOI] [PubMed] [Google Scholar]
- Anderson B. J., Li X., Alcantara A. A., Isaacs K. R., Black J. E., Greenough W. T. Glial hypertrophy is associated with synaptogenesis following motor-skill learning, but not with angiogenesis following exercise. Glia. 1994 May;11(1):73–80. doi: 10.1002/glia.440110110. [DOI] [PubMed] [Google Scholar]
- Barber P. C., Lindsay R. M. Schwann cells of the olfactory nerves contain glial fibrillary acidic protein and resemble astrocytes. Neuroscience. 1982;7(12):3077–3090. doi: 10.1016/0306-4522(82)90231-7. [DOI] [PubMed] [Google Scholar]
- Bliss T. V., Collingridge G. L. A synaptic model of memory: long-term potentiation in the hippocampus. Nature. 1993 Jan 7;361(6407):31–39. doi: 10.1038/361031a0. [DOI] [PubMed] [Google Scholar]
- Bovolenta P., Liem R. K., Mason C. A. Development of cerebellar astroglia: transitions in form and cytoskeletal content. Dev Biol. 1984 Mar;102(1):248–259. doi: 10.1016/0012-1606(84)90189-1. [DOI] [PubMed] [Google Scholar]
- Canady K. S., Rubel E. W. Rapid and reversible astrocytic reaction to afferent activity blockade in chick cochlear nucleus. J Neurosci. 1992 Mar;12(3):1001–1009. doi: 10.1523/JNEUROSCI.12-03-01001.1992. [DOI] [PMC free article] [PubMed] [Google Scholar]
- Chen W. J., Liem R. K. Reexpression of glial fibrillary acidic protein rescues the ability of astrocytoma cells to form processes in response to neurons. J Cell Biol. 1994 Nov;127(3):813–823. doi: 10.1083/jcb.127.3.813. [DOI] [PMC free article] [PubMed] [Google Scholar]
- Clarke S. R., Shetty A. K., Bradley J. L., Turner D. A. Reactive astrocytes express the embryonic intermediate neurofilament nestin. Neuroreport. 1994 Oct 3;5(15):1885–1888. doi: 10.1097/00001756-199410000-00011. [DOI] [PubMed] [Google Scholar]
- Cochard P., Paulin D. Initial expression of neurofilaments and vimentin in the central and peripheral nervous system of the mouse embryo in vivo. J Neurosci. 1984 Aug;4(8):2080–2094. doi: 10.1523/JNEUROSCI.04-08-02080.1984. [DOI] [PMC free article] [PubMed] [Google Scholar]
- Collinge J., Whittington M. A., Sidle K. C., Smith C. J., Palmer M. S., Clarke A. R., Jefferys J. G. Prion protein is necessary for normal synaptic function. Nature. 1994 Jul 28;370(6487):295–297. doi: 10.1038/370295a0. [DOI] [PubMed] [Google Scholar]
- Colucci-Guyon E., Portier M. M., Dunia I., Paulin D., Pournin S., Babinet C. Mice lacking vimentin develop and reproduce without an obvious phenotype. Cell. 1994 Nov 18;79(4):679–694. doi: 10.1016/0092-8674(94)90553-3. [DOI] [PubMed] [Google Scholar]
- Conquet F., Bashir Z. I., Davies C. H., Daniel H., Ferraguti F., Bordi F., Franz-Bacon K., Reggiani A., Matarese V., Condé F. Motor deficit and impairment of synaptic plasticity in mice lacking mGluR1. Nature. 1994 Nov 17;372(6503):237–243. doi: 10.1038/372237a0. [DOI] [PubMed] [Google Scholar]
- Dahl D., Bignami A., Weber K., Osborn M. Filament proteins in rat optic nerves undergoing Wallerian degeneration: localization of vimentin, the fibroblastic 100-A filament protein, in normal and reactive astrocytes. Exp Neurol. 1981 Aug;73(2):496–506. doi: 10.1016/0014-4886(81)90283-1. [DOI] [PubMed] [Google Scholar]
- Dahl D. The vimentin-GFA protein transition in rat neuroglia cytoskeleton occurs at the time of myelination. J Neurosci Res. 1981;6(6):741–748. doi: 10.1002/jnr.490060608. [DOI] [PubMed] [Google Scholar]
- Debus E., Weber K., Osborn M. Monoclonal antibodies specific for glial fibrillary acidic (GFA) protein and for each of the neurofilament triplet polypeptides. Differentiation. 1983;25(2):193–203. doi: 10.1111/j.1432-0436.1984.tb01355.x. [DOI] [PubMed] [Google Scholar]
- Eng L. F., Ghirnikar R. S. GFAP and astrogliosis. Brain Pathol. 1994 Jul;4(3):229–237. doi: 10.1111/j.1750-3639.1994.tb00838.x. [DOI] [PubMed] [Google Scholar]
- Eyer J., Peterson A. Neurofilament-deficient axons and perikaryal aggregates in viable transgenic mice expressing a neurofilament-beta-galactosidase fusion protein. Neuron. 1994 Feb;12(2):389–405. doi: 10.1016/0896-6273(94)90280-1. [DOI] [PubMed] [Google Scholar]
- Fedoroff S., White R., Neal J., Subrahmanyan L., Kalnins V. I. Astrocyte cell lineage. II. Mouse fibrous astrocytes and reactive astrocytes in cultures have vimentin- and GFP-containing intermediate filaments. Brain Res. 1983 Apr;283(2-3):303–315. doi: 10.1016/0165-3806(83)90187-6. [DOI] [PubMed] [Google Scholar]
- Feinstein D. L., Weinmaster G. A., Milner R. J. Isolation of cDNA clones encoding rat glial fibrillary acidic protein: expression in astrocytes and in Schwann cells. J Neurosci Res. 1992 May;32(1):1–14. doi: 10.1002/jnr.490320102. [DOI] [PubMed] [Google Scholar]
- Gomi H., Yokoyama T., Fujimoto K., Ikeda T., Katoh A., Itoh T., Itohara S. Mice devoid of the glial fibrillary acidic protein develop normally and are susceptible to scrapie prions. Neuron. 1995 Jan;14(1):29–41. doi: 10.1016/0896-6273(95)90238-4. [DOI] [PubMed] [Google Scholar]
- Grant S. G., O'Dell T. J., Karl K. A., Stein P. L., Soriano P., Kandel E. R. Impaired long-term potentiation, spatial learning, and hippocampal development in fyn mutant mice. Science. 1992 Dec 18;258(5090):1903–1910. doi: 10.1126/science.1361685. [DOI] [PubMed] [Google Scholar]
- Hirschhorn R. R., Aller P., Yuan Z. A., Gibson C. W., Baserga R. Cell-cycle-specific cDNAs from mammalian cells temperature sensitive for growth. Proc Natl Acad Sci U S A. 1984 Oct;81(19):6004–6008. doi: 10.1073/pnas.81.19.6004. [DOI] [PMC free article] [PubMed] [Google Scholar]
- Hosokawa T., Rusakov D. A., Bliss T. V., Fine A. Repeated confocal imaging of individual dendritic spines in the living hippocampal slice: evidence for changes in length and orientation associated with chemically induced LTP. J Neurosci. 1995 Aug;15(8):5560–5573. doi: 10.1523/JNEUROSCI.15-08-05560.1995. [DOI] [PMC free article] [PubMed] [Google Scholar]
- Janzer R. C., Raff M. C. Astrocytes induce blood-brain barrier properties in endothelial cells. Nature. 1987 Jan 15;325(6101):253–257. doi: 10.1038/325253a0. [DOI] [PubMed] [Google Scholar]
- Jessen K. R., Mirsky R. Nonmyelin-forming Schwann cells coexpress surface proteins and intermediate filaments not found in myelin-forming cells: a study of Ran-2, A5E3 antigen and glial fibrillary acidic protein. J Neurocytol. 1984 Dec;13(6):923–934. doi: 10.1007/BF01148594. [DOI] [PubMed] [Google Scholar]
- Korte M., Carroll P., Wolf E., Brem G., Thoenen H., Bonhoeffer T. Hippocampal long-term potentiation is impaired in mice lacking brain-derived neurotrophic factor. Proc Natl Acad Sci U S A. 1995 Sep 12;92(19):8856–8860. doi: 10.1073/pnas.92.19.8856. [DOI] [PMC free article] [PubMed] [Google Scholar]
- Lendahl U., Zimmerman L. B., McKay R. D. CNS stem cells express a new class of intermediate filament protein. Cell. 1990 Feb 23;60(4):585–595. doi: 10.1016/0092-8674(90)90662-x. [DOI] [PubMed] [Google Scholar]
- Lewis S. A., Balcarek J. M., Krek V., Shelanski M., Cowan N. J. Sequence of a cDNA clone encoding mouse glial fibrillary acidic protein: structural conservation of intermediate filaments. Proc Natl Acad Sci U S A. 1984 May;81(9):2743–2746. doi: 10.1073/pnas.81.9.2743. [DOI] [PMC free article] [PubMed] [Google Scholar]
- Müller H. W., Junghans U., Kappler J. Astroglial neurotrophic and neurite-promoting factors. Pharmacol Ther. 1995 Jan;65(1):1–18. doi: 10.1016/0163-7258(94)00047-7. [DOI] [PubMed] [Google Scholar]
- Ohara O., Gahara Y., Miyake T., Teraoka H., Kitamura T. Neurofilament deficiency in quail caused by nonsense mutation in neurofilament-L gene. J Cell Biol. 1993 Apr;121(2):387–395. doi: 10.1083/jcb.121.2.387. [DOI] [PMC free article] [PubMed] [Google Scholar]
- Pekny M., Levéen P., Pekna M., Eliasson C., Berthold C. H., Westermark B., Betsholtz C. Mice lacking glial fibrillary acidic protein display astrocytes devoid of intermediate filaments but develop and reproduce normally. EMBO J. 1995 Apr 18;14(8):1590–1598. doi: 10.1002/j.1460-2075.1995.tb07147.x. [DOI] [PMC free article] [PubMed] [Google Scholar]
- Pixley S. K., de Vellis J. Transition between immature radial glia and mature astrocytes studied with a monoclonal antibody to vimentin. Brain Res. 1984 Aug;317(2):201–209. doi: 10.1016/0165-3806(84)90097-x. [DOI] [PubMed] [Google Scholar]
- Pleasure S. J., Lee V. M., Nelson D. L. Site-specific phosphorylation of the middle molecular weight human neurofilament protein in transfected non-neuronal cells. J Neurosci. 1990 Jul;10(7):2428–2437. doi: 10.1523/JNEUROSCI.10-07-02428.1990. [DOI] [PMC free article] [PubMed] [Google Scholar]
- Ramírez-Solis R., Davis A. C., Bradley A. Gene targeting in embryonic stem cells. Methods Enzymol. 1993;225:855–878. doi: 10.1016/0076-6879(93)25054-6. [DOI] [PubMed] [Google Scholar]
- Rudge John S., Alderson Ralph F., Pasnikowski Elizabeth, McClain Joyce, Ip Nancy Y., Lindsay Ronald M. Expression of Ciliary Neurotrophic Factor and the Neurotrophins-Nerve Growth Factor, Brain-Derived Neurotrophic Factor and Neurotrophin 3-in Cultured Rat Hippocampal Astrocytes. Eur J Neurosci. 1992;4(6):459–471. doi: 10.1111/j.1460-9568.1992.tb00896.x. [DOI] [PubMed] [Google Scholar]
- Rutka J. T., Smith S. L. Transfection of human astrocytoma cells with glial fibrillary acidic protein complementary DNA: analysis of expression, proliferation, and tumorigenicity. Cancer Res. 1993 Aug 1;53(15):3624–3631. [PubMed] [Google Scholar]
- Sakimura K., Kutsuwada T., Ito I., Manabe T., Takayama C., Kushiya E., Yagi T., Aizawa S., Inoue Y., Sugiyama H. Reduced hippocampal LTP and spatial learning in mice lacking NMDA receptor epsilon 1 subunit. Nature. 1995 Jan 12;373(6510):151–155. doi: 10.1038/373151a0. [DOI] [PubMed] [Google Scholar]
- Silva A. J., Stevens C. F., Tonegawa S., Wang Y. Deficient hippocampal long-term potentiation in alpha-calcium-calmodulin kinase II mutant mice. Science. 1992 Jul 10;257(5067):201–206. doi: 10.1126/science.1378648. [DOI] [PubMed] [Google Scholar]
- Smith S. J. Do astrocytes process neural information? Prog Brain Res. 1992;94:119–136. doi: 10.1016/s0079-6123(08)61744-6. [DOI] [PubMed] [Google Scholar]
- Soriano P., Montgomery C., Geske R., Bradley A. Targeted disruption of the c-src proto-oncogene leads to osteopetrosis in mice. Cell. 1991 Feb 22;64(4):693–702. doi: 10.1016/0092-8674(91)90499-o. [DOI] [PubMed] [Google Scholar]
- Steward O., Torre E. R., Tomasulo R., Lothman E. Neuronal activity up-regulates astroglial gene expression. Proc Natl Acad Sci U S A. 1991 Aug 1;88(15):6819–6823. doi: 10.1073/pnas.88.15.6819. [DOI] [PMC free article] [PubMed] [Google Scholar]
- Weinstein D. E., Shelanski M. L., Liem R. K. Suppression by antisense mRNA demonstrates a requirement for the glial fibrillary acidic protein in the formation of stable astrocytic processes in response to neurons. J Cell Biol. 1991 Mar;112(6):1205–1213. doi: 10.1083/jcb.112.6.1205. [DOI] [PMC free article] [PubMed] [Google Scholar]
- Wenzel J., Lammert G., Meyer U., Krug M. The influence of long-term potentiation on the spatial relationship between astrocyte processes and potentiated synapses in the dentate gyrus neuropil of rat brain. Brain Res. 1991 Sep 27;560(1-2):122–131. doi: 10.1016/0006-8993(91)91222-m. [DOI] [PubMed] [Google Scholar]
- Zerlin M., Levison S. W., Goldman J. E. Early patterns of migration, morphogenesis, and intermediate filament expression of subventricular zone cells in the postnatal rat forebrain. J Neurosci. 1995 Nov;15(11):7238–7249. doi: 10.1523/JNEUROSCI.15-11-07238.1995. [DOI] [PMC free article] [PubMed] [Google Scholar]