Abstract
A detailed mechanism that fully accounts for the Mg2+-induced polymerization of actin in the presence or absence of Ca2+ at 20 degrees C and pH 8 is presented. In the absence of Ca2+, the mechanism of the Mg2+-induced polymerization is as follows: Mg2+ binds to a metal-binding site on G-actin and induces a conformational change, which is required for eventual polymerization. The overall dissociation constant for this binding is about 30 microM. This actin species then binds a second molecule of Mg2+ (Kd = 5 mM), which yields a species capable of polymerization. Dimer formation from this monomeric species is quite unfavorable, but trimer formation from dimer and monomer is much more favorable. The trimer may then elongate to give filaments. Ca2+, when present, binds at the same site as the tightly bound Mg2+ and must be displaced by Mg2+ before the conformational change can occur. The rate and dissociation constants for tight binding of Ca2+ and Mg2+ and for the conformational change are consistent with those observed previously by using a fluorescently labeled G-actin. With the mechanism proposed, it is possible to fit the full time course of polymerization over a wide range of actin concentrations, Mg2+ concentrations, and Ca2+ concentrations.
Full text
PDF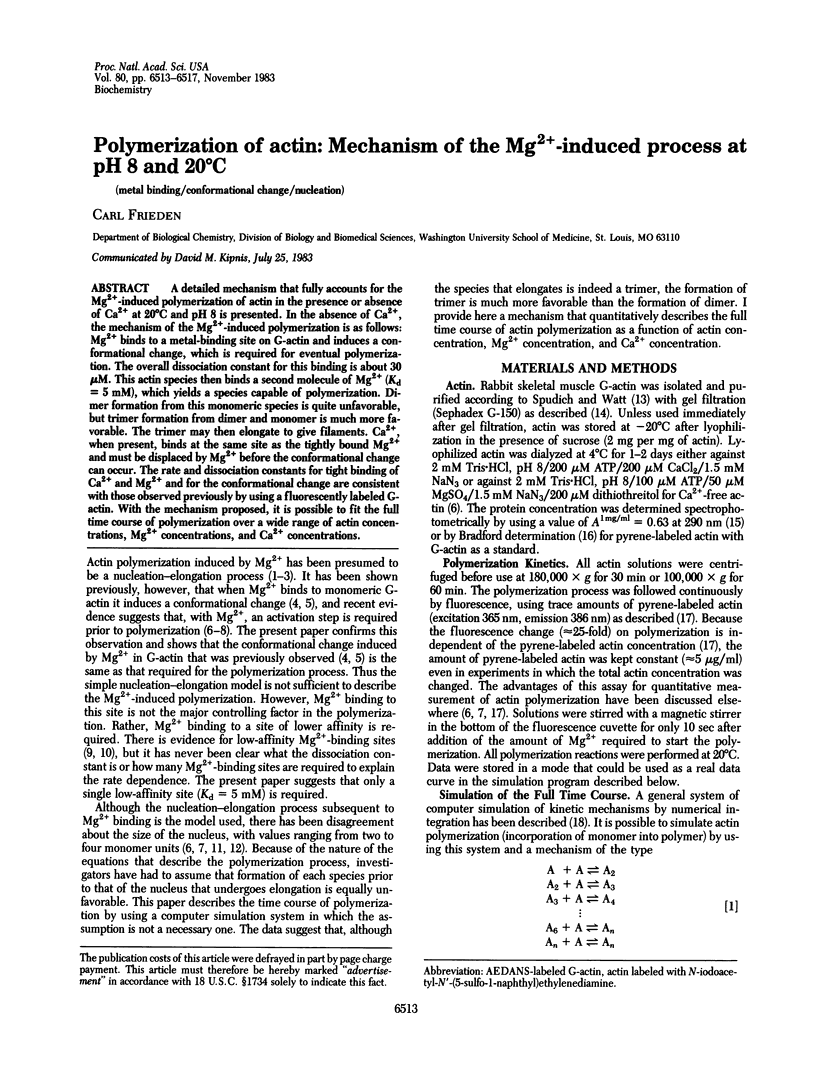
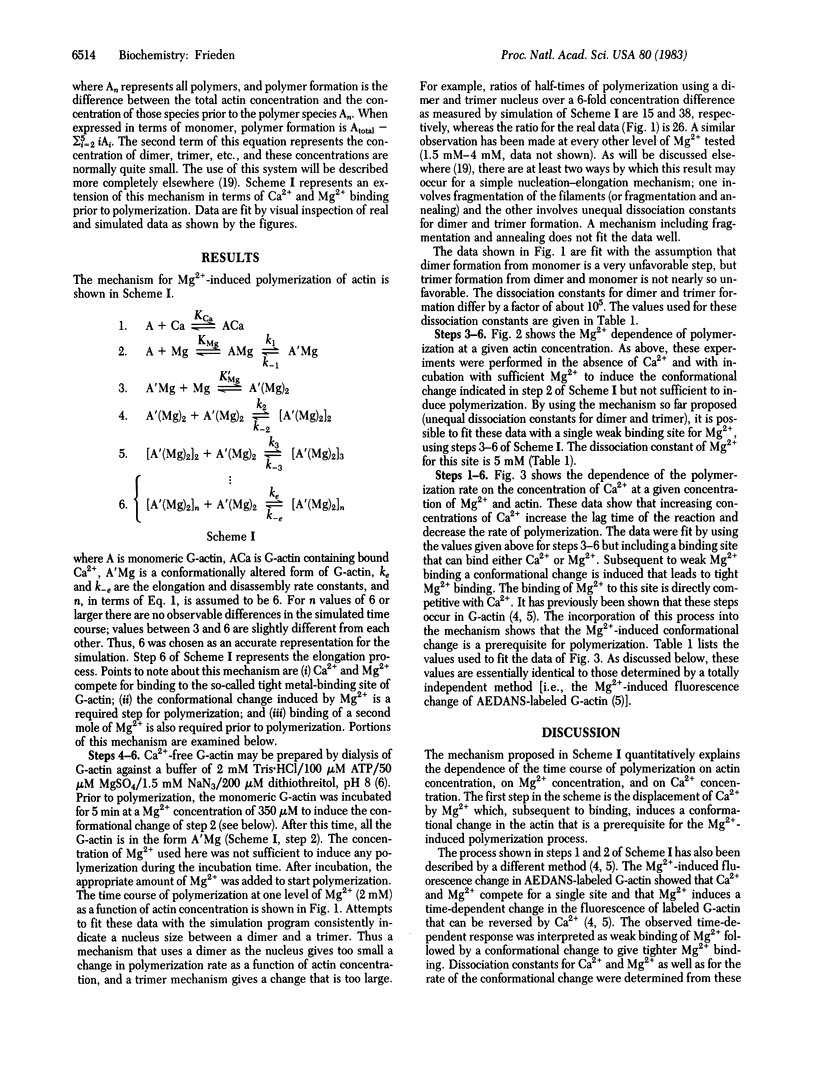
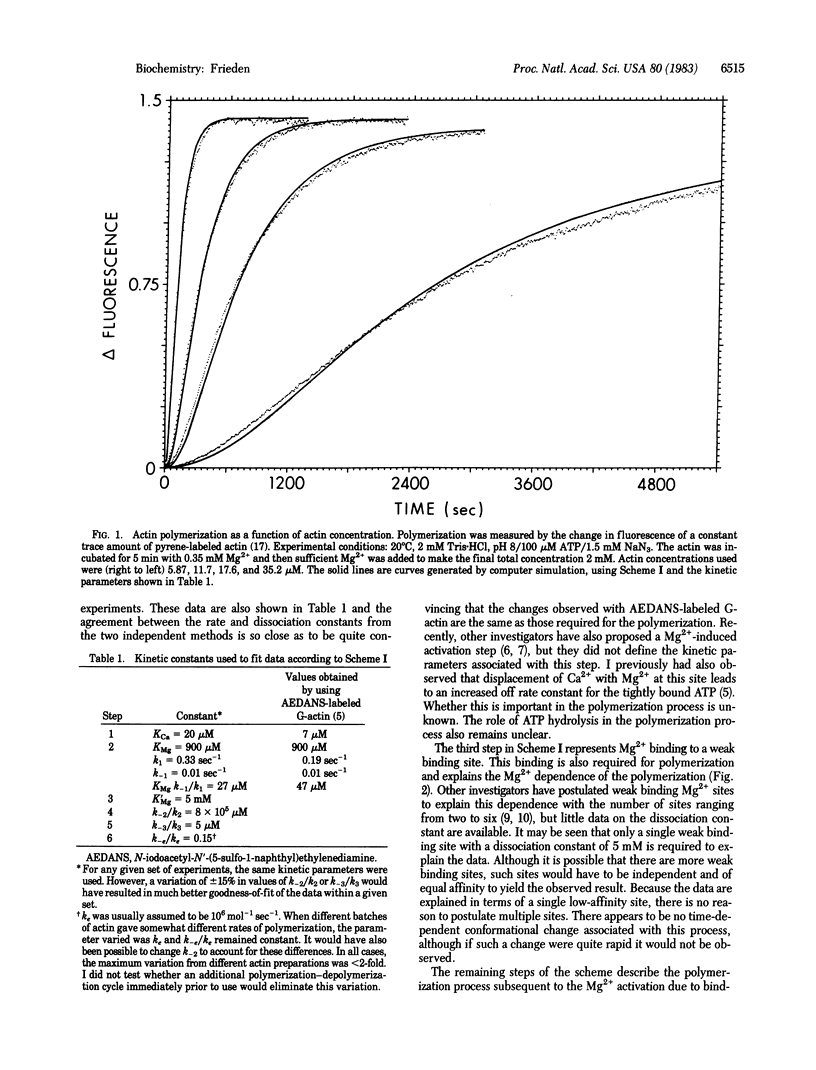
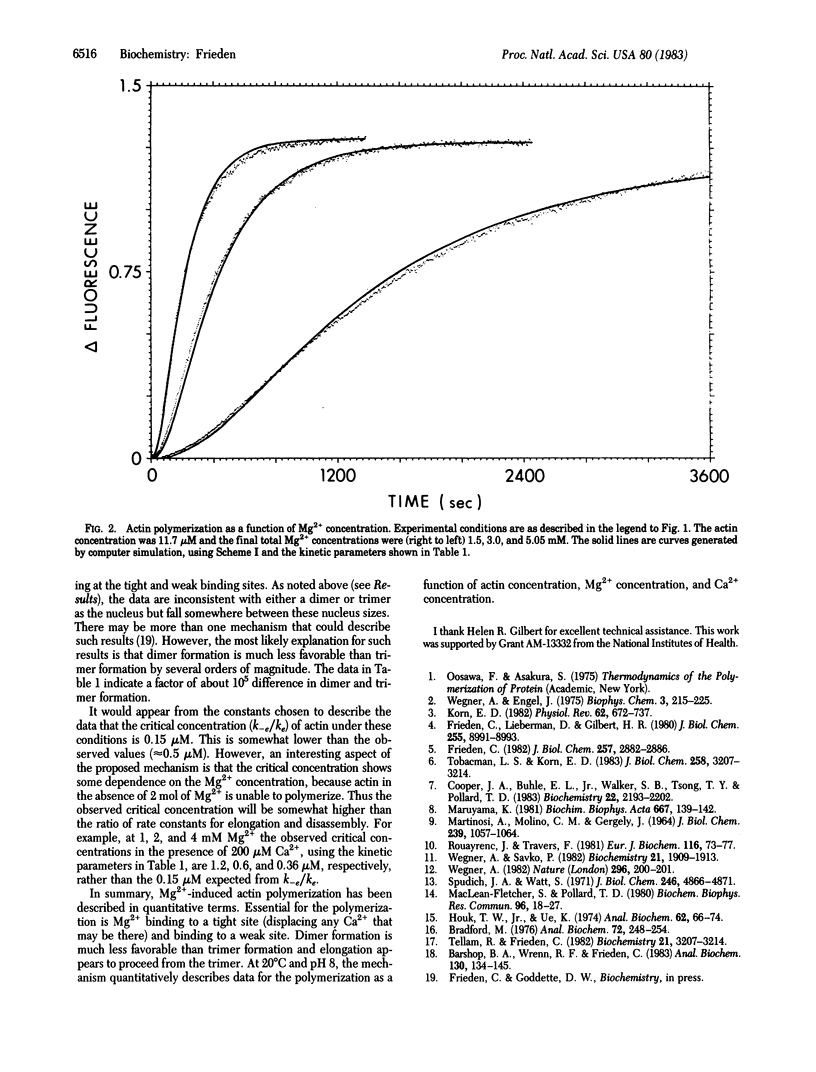
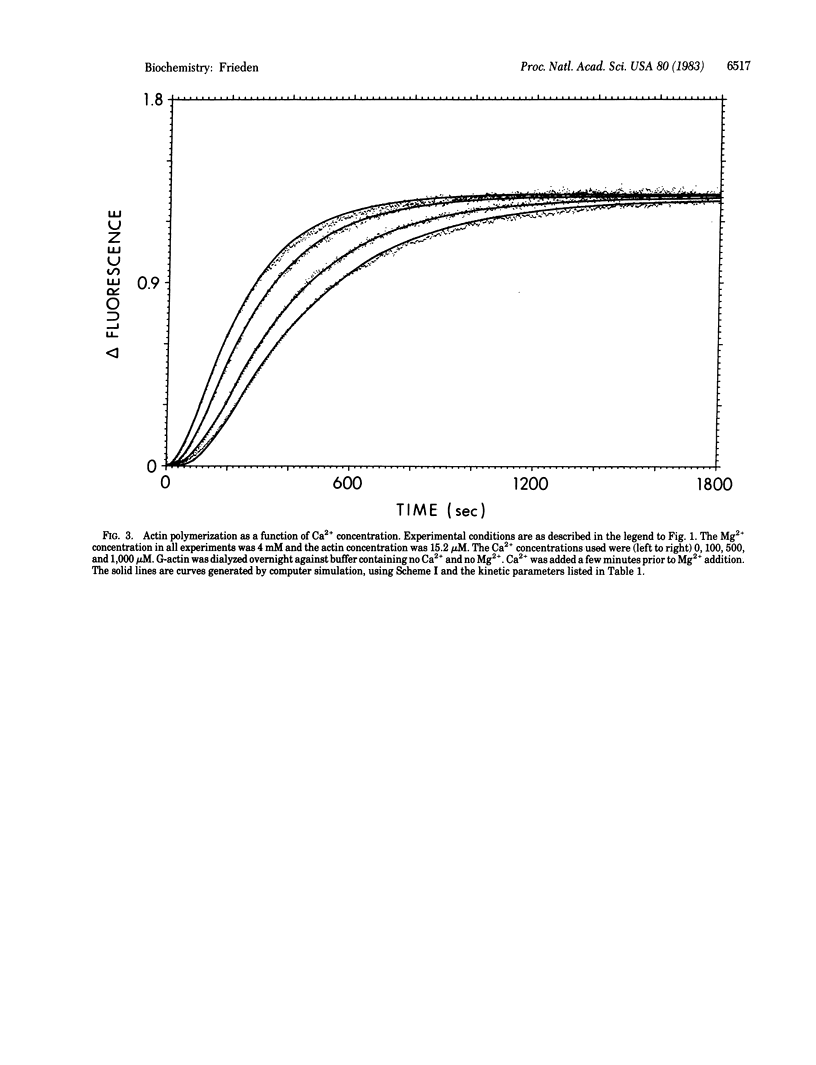
Selected References
These references are in PubMed. This may not be the complete list of references from this article.
- Barshop B. A., Wrenn R. F., Frieden C. Analysis of numerical methods for computer simulation of kinetic processes: development of KINSIM--a flexible, portable system. Anal Biochem. 1983 Apr 1;130(1):134–145. doi: 10.1016/0003-2697(83)90660-7. [DOI] [PubMed] [Google Scholar]
- Bradford M. M. A rapid and sensitive method for the quantitation of microgram quantities of protein utilizing the principle of protein-dye binding. Anal Biochem. 1976 May 7;72:248–254. doi: 10.1016/0003-2697(76)90527-3. [DOI] [PubMed] [Google Scholar]
- Cooper J. A., Buhle E. L., Jr, Walker S. B., Tsong T. Y., Pollard T. D. Kinetic evidence for a monomer activation step in actin polymerization. Biochemistry. 1983 Apr 26;22(9):2193–2202. doi: 10.1021/bi00278a021. [DOI] [PubMed] [Google Scholar]
- Frieden C., Lieberman D., Gilbert H. R. A fluorescent probe for conformational changes in skeletal muscle G-actin. J Biol Chem. 1980 Oct 10;255(19):8991–8993. [PubMed] [Google Scholar]
- Frieden C. The Mg2+-induced conformational change in rabbit skeletal muscle G-actin. J Biol Chem. 1982 Mar 25;257(6):2882–2886. [PubMed] [Google Scholar]
- Houk T. W., Jr, Ue K. The measurement of actin concentration in solution: a comparison of methods. Anal Biochem. 1974 Nov;62(1):66–74. doi: 10.1016/0003-2697(74)90367-4. [DOI] [PubMed] [Google Scholar]
- Korn E. D. Actin polymerization and its regulation by proteins from nonmuscle cells. Physiol Rev. 1982 Apr;62(2):672–737. doi: 10.1152/physrev.1982.62.2.672. [DOI] [PubMed] [Google Scholar]
- Lane D., Koprowski H. Molecular recognition and the future of monoclonal antibodies. Nature. 1982 Mar 18;296(5854):200–202. doi: 10.1038/296200a0. [DOI] [PubMed] [Google Scholar]
- MARTONOSII A., MOLINO C. M., GERGELY J. THE BINDING OF DIVALENT CATIONS TO ACTIN. J Biol Chem. 1964 Apr;239:1057–1064. [PubMed] [Google Scholar]
- MacLean-Fletcher S., Pollard T. D. Identification of a factor in conventional muscle actin preparations which inhibits actin filament self-association. Biochem Biophys Res Commun. 1980 Sep 16;96(1):18–27. doi: 10.1016/0006-291x(80)91175-4. [DOI] [PubMed] [Google Scholar]
- Maruyama K. Effects of trace amounts of Ca2+ and Mg2+ on the polymerization of actin. Biochim Biophys Acta. 1981 Jan 30;667(1):139–142. doi: 10.1016/0005-2795(81)90074-x. [DOI] [PubMed] [Google Scholar]
- Rouayrenc J. F., Travers F. The first step in the polymerisation of actin. Eur J Biochem. 1981 May;116(1):73–77. doi: 10.1111/j.1432-1033.1981.tb05302.x. [DOI] [PubMed] [Google Scholar]
- Spudich J. A., Watt S. The regulation of rabbit skeletal muscle contraction. I. Biochemical studies of the interaction of the tropomyosin-troponin complex with actin and the proteolytic fragments of myosin. J Biol Chem. 1971 Aug 10;246(15):4866–4871. [PubMed] [Google Scholar]
- Tellam R., Frieden C. Cytochalasin D and platelet gelsolin accelerate actin polymer formation. A model for regulation of the extent of actin polymer formation in vivo. Biochemistry. 1982 Jun 22;21(13):3207–3214. doi: 10.1021/bi00256a027. [DOI] [PubMed] [Google Scholar]
- Tobacman L. S., Korn E. D. The kinetics of actin nucleation and polymerization. J Biol Chem. 1983 Mar 10;258(5):3207–3214. [PubMed] [Google Scholar]
- Wegner A., Engel J. Kinetics of the cooperative association of actin to actin filaments. Biophys Chem. 1975 Jul;3(3):215–225. doi: 10.1016/0301-4622(75)80013-5. [DOI] [PubMed] [Google Scholar]
- Wegner A., Savko P. Fragmentation of actin filaments. Biochemistry. 1982 Apr 13;21(8):1909–1913. doi: 10.1021/bi00537a032. [DOI] [PubMed] [Google Scholar]