Abstract
The first three steps in mammalian de novo pyrimidine biosynthesis are catalyzed by the multifunctional protein designated CAD. Regions of the single 240-kDa poly-peptide chain are folded into separate structural domains that have discrete functions. Previous studies suggested that CAD forms predominantly trimers. The trimers are found to be in slow equilibrium with hexamers and higher oligomers composed of multiples of three copies of the CAD polypeptide chain. However, quantitative chemical crosslinking studies of CAD with dimethyl suberimidate were used here to show a progressive conversion of monomer to crosslinked hexamer. High levels of the hexamer accumulate in the reaction mixture, suggesting that the major oligomeric form is hexameric, although residual amounts of smaller oligomers remain present. Larger oligomers may form by association of hexamers and are seen after longer crosslinking times. Sucrose gradient centrifugation shows a 20.8S species to be the slowest sedimenting peak, while the larger species sediments at 27.9S. Electron microscopic studies of rotary-shadowed preparations of CAD have confirmed that, while small amounts of other oligomeric forms are present, the CAD monomer is primarily associated into cyclic hexamers with an open planar appearance.
Full text
PDF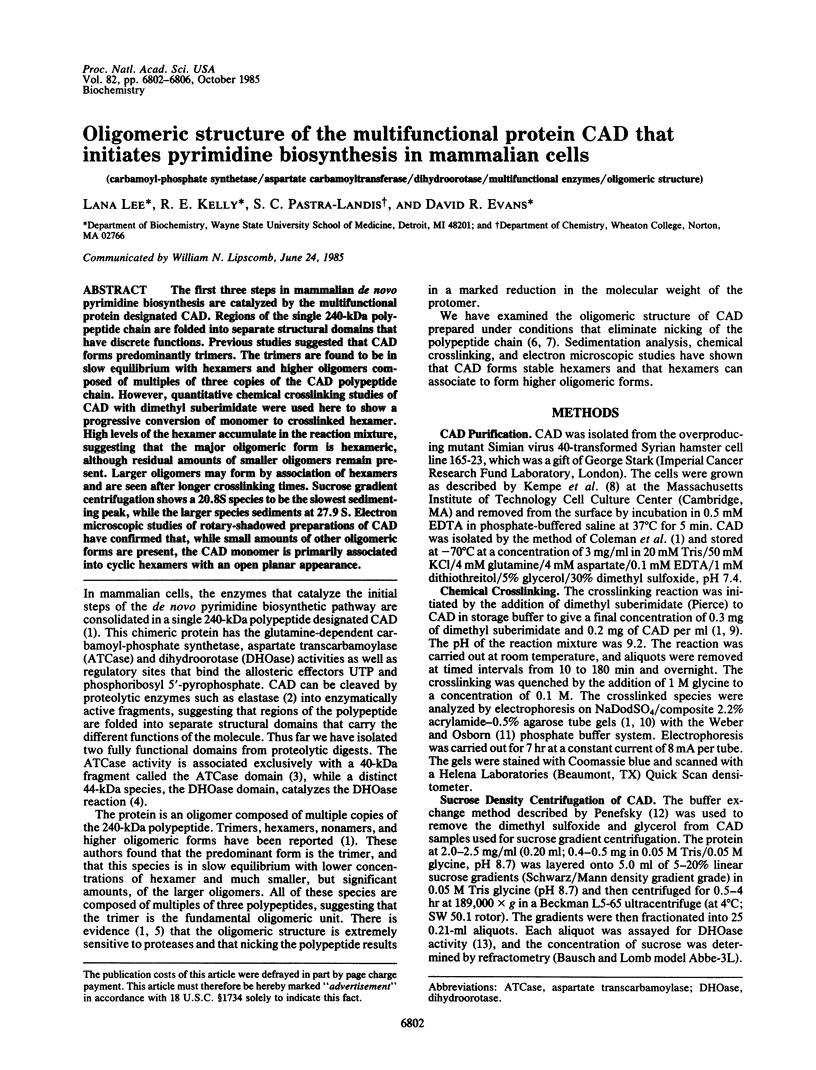
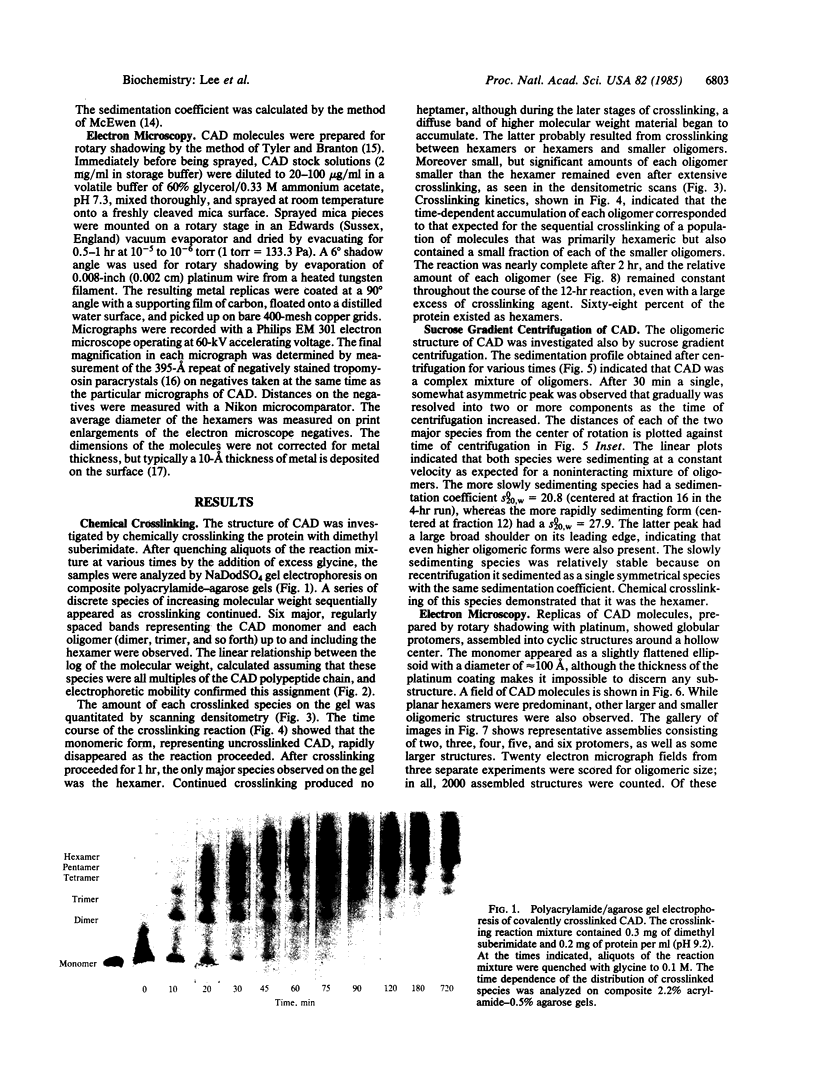
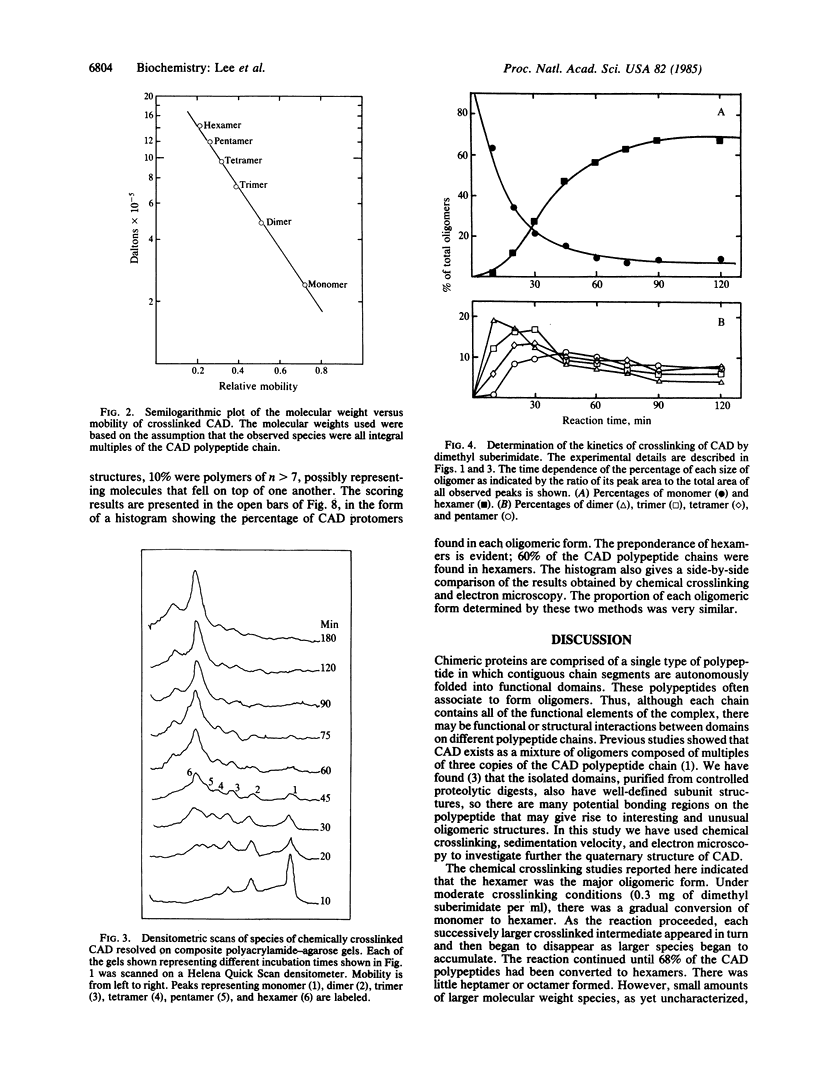
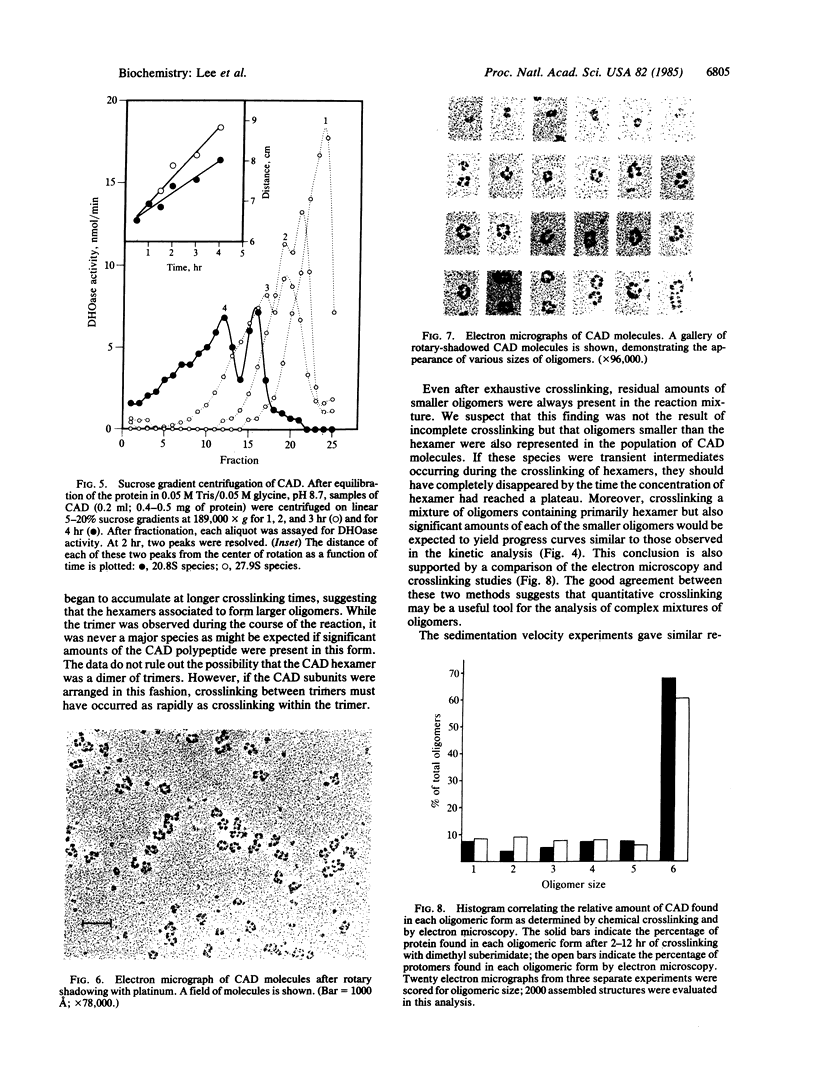
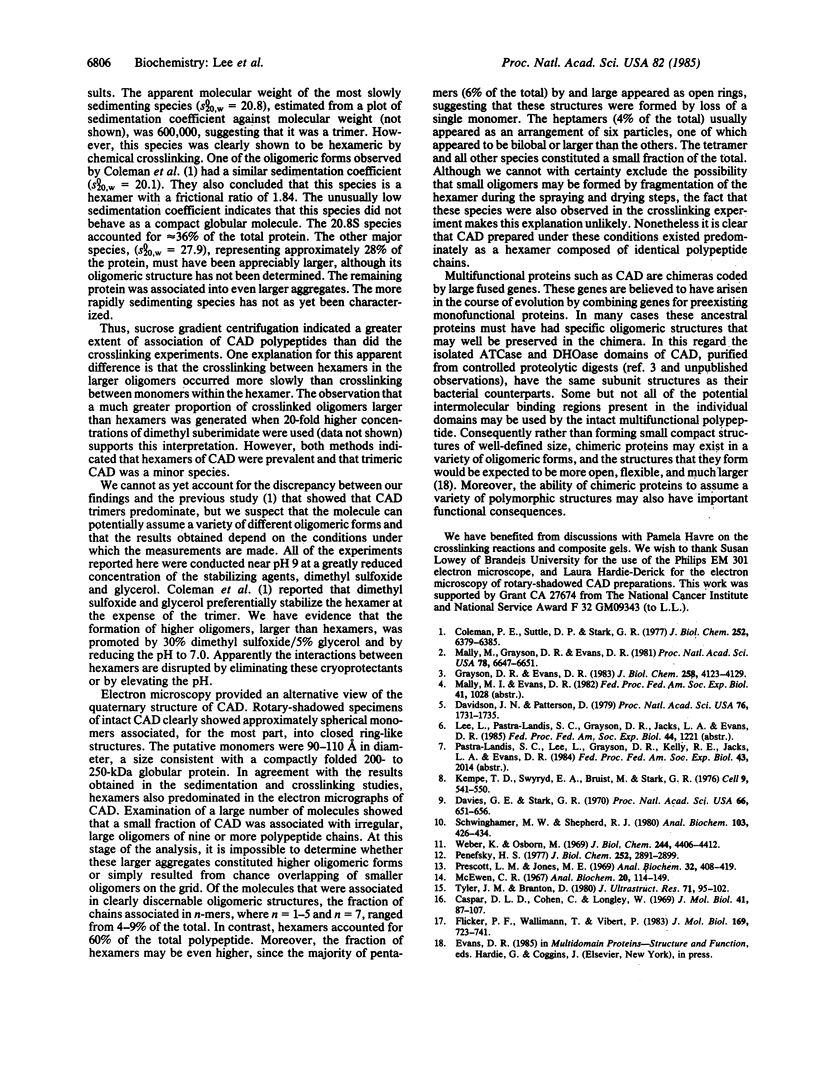
Images in this article
Selected References
These references are in PubMed. This may not be the complete list of references from this article.
- Caspar D. L., Cohen C., Longley W. Tropomyosin: crystal structure, polymorphism and molecular interactions. J Mol Biol. 1969 Apr 14;41(1):87–107. doi: 10.1016/0022-2836(69)90128-4. [DOI] [PubMed] [Google Scholar]
- Coleman P. F., Suttle D. P., Stark G. R. Purification from hamster cells of the multifunctional protein that initiates de novo synthesis of pyrimidine nucleotides. J Biol Chem. 1977 Sep 25;252(18):6379–6385. [PubMed] [Google Scholar]
- Davidson J. N., Patterson D. Alteration in structure of multifunctional protein from Chinese hamster ovary cells defective in pyrimidine biosynthesis. Proc Natl Acad Sci U S A. 1979 Apr;76(4):1731–1735. doi: 10.1073/pnas.76.4.1731. [DOI] [PMC free article] [PubMed] [Google Scholar]
- Davies G. E., Stark G. R. Use of dimethyl suberimidate, a cross-linking reagent, in studying the subunit structure of oligomeric proteins. Proc Natl Acad Sci U S A. 1970 Jul;66(3):651–656. doi: 10.1073/pnas.66.3.651. [DOI] [PMC free article] [PubMed] [Google Scholar]
- Flicker P. F., Wallimann T., Vibert P. Electron microscopy of scallop myosin. Location of regulatory light chains. J Mol Biol. 1983 Sep 25;169(3):723–741. doi: 10.1016/s0022-2836(83)80167-3. [DOI] [PubMed] [Google Scholar]
- Grayson D. R., Evans D. R. The isolation and characterization of the aspartate transcarbamylase domain of the multifunctional protein, CAD. J Biol Chem. 1983 Apr 10;258(7):4123–4129. [PubMed] [Google Scholar]
- Kempe T. D., Swyryd E. A., Bruist M., Stark G. R. Stable mutants of mammalian cells that overproduce the first three enzymes of pyrimidine nucleotide biosynthesis. Cell. 1976 Dec;9(4 Pt 1):541–550. doi: 10.1016/0092-8674(76)90036-2. [DOI] [PubMed] [Google Scholar]
- Mally M. I., Grayson D. R., Evans D. R. Controlled proteolysis of the multifunctional protein that initiates pyrimidine biosynthesis in mammalian cells: evidence for discrete structural domains. Proc Natl Acad Sci U S A. 1981 Nov;78(11):6647–6651. doi: 10.1073/pnas.78.11.6647. [DOI] [PMC free article] [PubMed] [Google Scholar]
- McEwen C. R. Tables for estimating sedimentation through linear concentration gradients of sucrose solution. Anal Biochem. 1967 Jul;20(1):114–149. doi: 10.1016/0003-2697(67)90271-0. [DOI] [PubMed] [Google Scholar]
- Penefsky H. S. Reversible binding of Pi by beef heart mitochondrial adenosine triphosphatase. J Biol Chem. 1977 May 10;252(9):2891–2899. [PubMed] [Google Scholar]
- Prescott L. M., Jones M. E. Modified methods for the determination of carbamyl aspartate. Anal Biochem. 1969 Dec;32(3):408–419. doi: 10.1016/s0003-2697(69)80008-4. [DOI] [PubMed] [Google Scholar]
- Schwinghamer M. W., Shepherd R. J. Formaldehyde-containing slab gels for analysis of denatured, tritium-labeled RNA. Anal Biochem. 1980 Apr;103(2):426–434. doi: 10.1016/0003-2697(80)90634-x. [DOI] [PubMed] [Google Scholar]
- Tyler J. M., Branton D. Rotary shadowing of extended molecules dried from glycerol. J Ultrastruct Res. 1980 May;71(2):95–102. doi: 10.1016/s0022-5320(80)90098-2. [DOI] [PubMed] [Google Scholar]
- Weber K., Osborn M. The reliability of molecular weight determinations by dodecyl sulfate-polyacrylamide gel electrophoresis. J Biol Chem. 1969 Aug 25;244(16):4406–4412. [PubMed] [Google Scholar]