Abstract
The principal catalytic factor in the activation of tyrosine by the tyrosyl-tRNA synthetase is found to be improved binding of ATP in the transition state. The activation reaction involves the attack of the tyrosyl carboxylate on the alpha-phosphate group of ATP to generate a pentacoordinate transition state. Model building of this complex located a binding site for the gamma-phosphate group of ATP, consisting of hydrogen bonds with the side chains of Thr-40 and His-45. Removal of these groups by protein engineering shows that they contribute no binding energy with unreacted ATP but put all of their binding energy into stabilizing the [tyrosine-ATP] transition state [the mutant tyrosyl-tRNA synthetase (Thr-40----Ala-40; His-45----Gly-45) has the rate of formation of tyrosyl adenylate lowered by 3.2 X 10(5) but KS for ATP is lowered by only a factor of 5]. The side chains of these residues also provide a binding site for pyrophosphate in the reverse reaction. Thus, catalysis is accomplished by stabilization of the transition state by improved binding of a group on the substrate that is distant from the seat of reaction.
Full text
PDF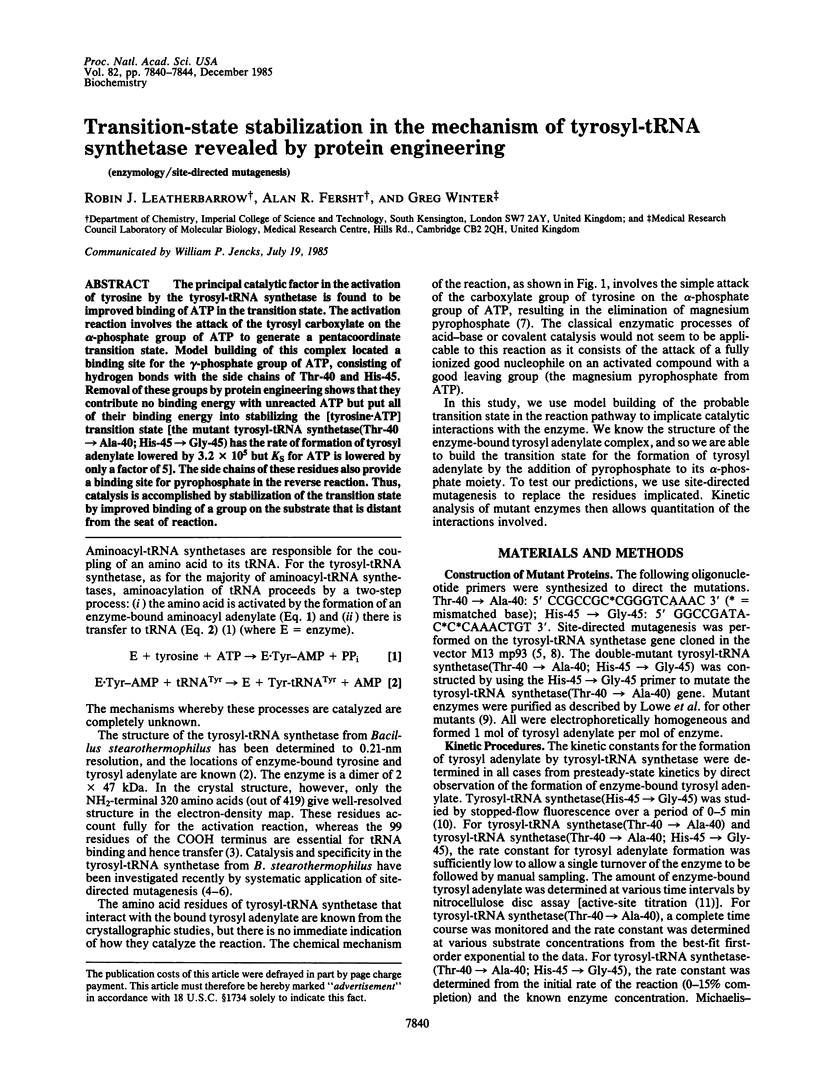
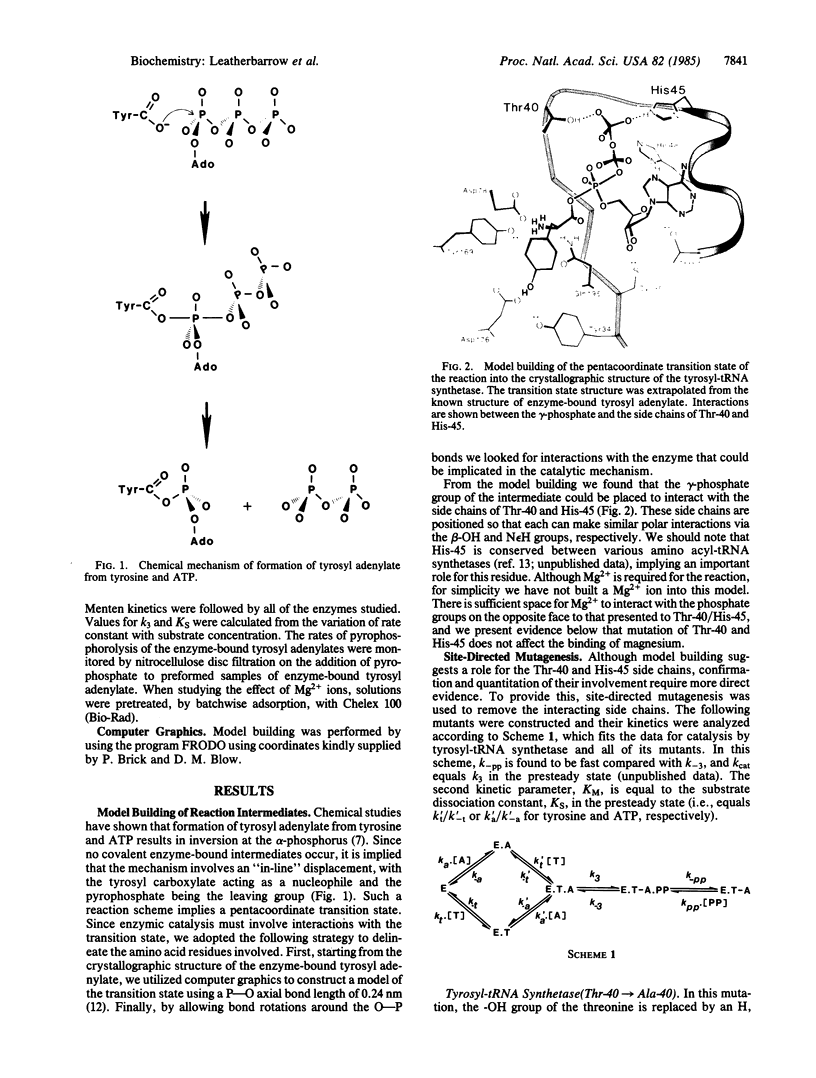
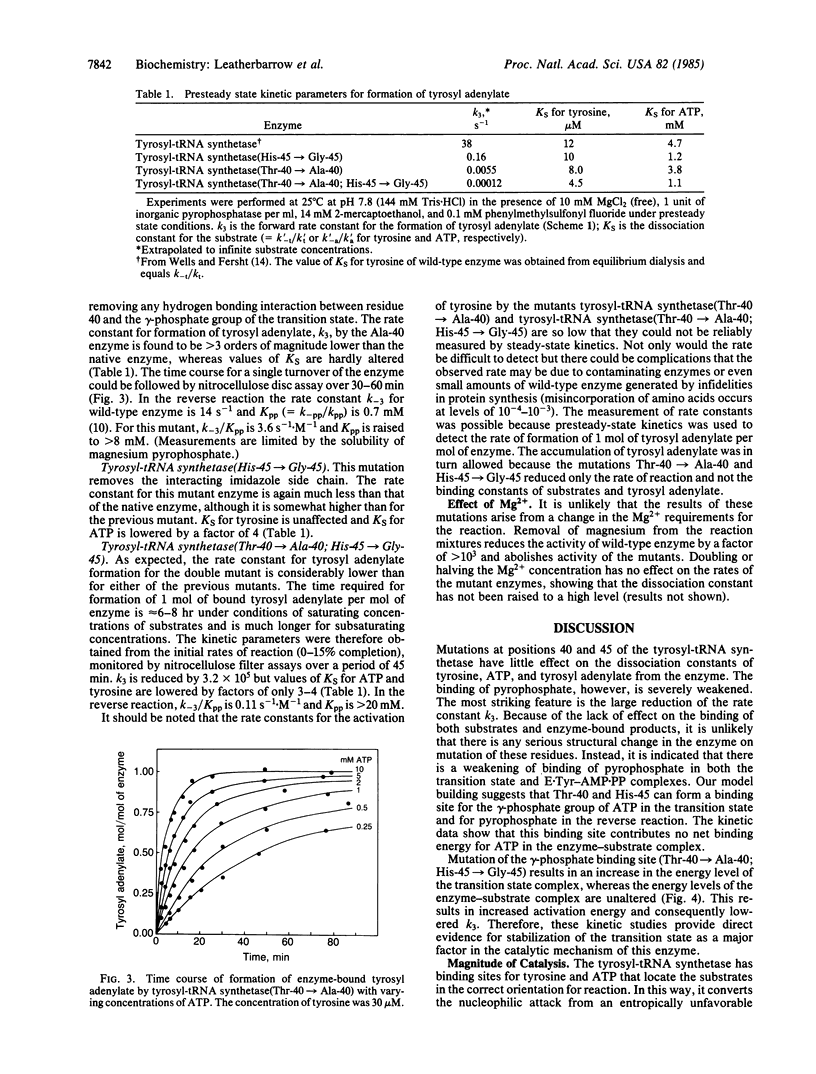
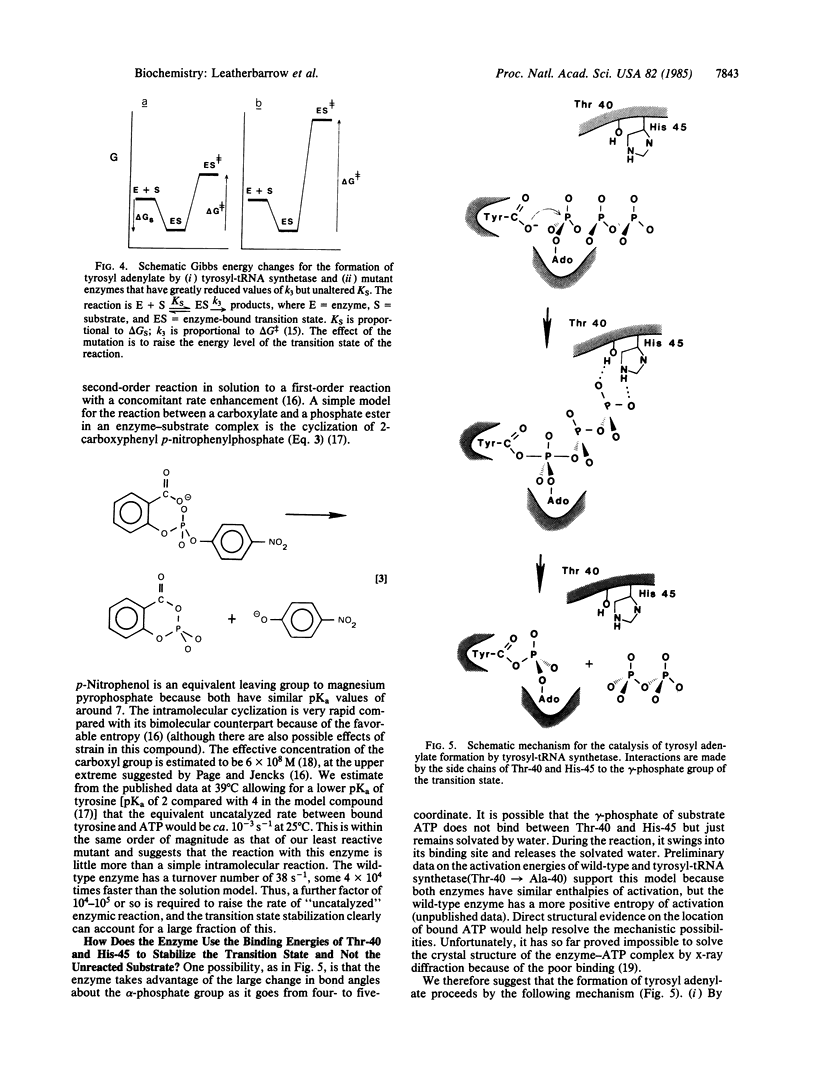
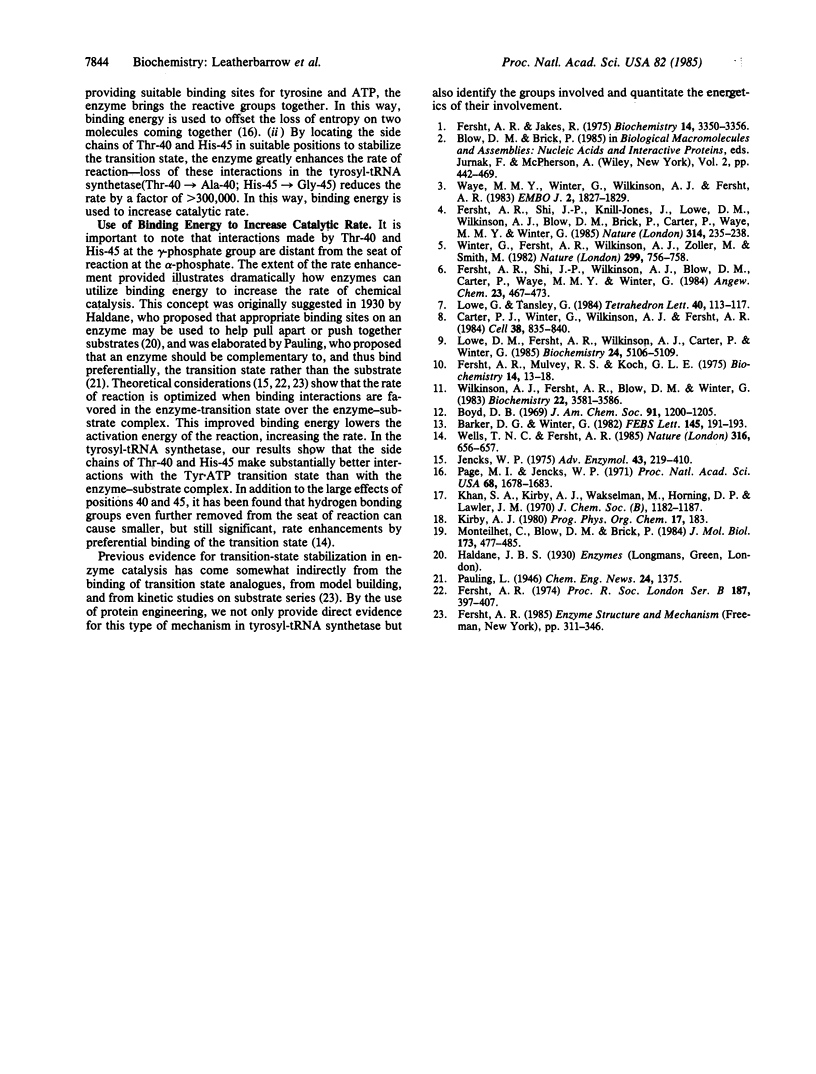
Images in this article
Selected References
These references are in PubMed. This may not be the complete list of references from this article.
- Barker D. G., Winter G. Conserved cysteine and histidine residues in the structures of the tyrosyl and methionyl-tRNA synthetases. FEBS Lett. 1982 Aug 23;145(2):191–193. doi: 10.1016/0014-5793(82)80165-8. [DOI] [PubMed] [Google Scholar]
- Carter P. J., Winter G., Wilkinson A. J., Fersht A. R. The use of double mutants to detect structural changes in the active site of the tyrosyl-tRNA synthetase (Bacillus stearothermophilus). Cell. 1984 Oct;38(3):835–840. doi: 10.1016/0092-8674(84)90278-2. [DOI] [PubMed] [Google Scholar]
- Fersht A. R. Catalysis, binding and enzyme-substrate complementarity. Proc R Soc Lond B Biol Sci. 1974 Nov 19;187(1089):397–407. doi: 10.1098/rspb.1974.0084. [DOI] [PubMed] [Google Scholar]
- Fersht A. R., Jakes R. Demonstration of two reaction pathways for the aminoacylation of tRNA. Application of the pulsed quenched flow technique. Biochemistry. 1975 Jul 29;14(15):3350–3356. doi: 10.1021/bi00686a010. [DOI] [PubMed] [Google Scholar]
- Fersht A. R., Mulvey R. S., Koch G. L. Ligand binding and enzymic catalysis coupled through subunits in tyrosyl-tRNA synthetase. Biochemistry. 1975 Jan 14;14(1):13–18. doi: 10.1021/bi00672a003. [DOI] [PubMed] [Google Scholar]
- Fersht A. R., Shi J. P., Knill-Jones J., Lowe D. M., Wilkinson A. J., Blow D. M., Brick P., Carter P., Waye M. M., Winter G. Hydrogen bonding and biological specificity analysed by protein engineering. Nature. 1985 Mar 21;314(6008):235–238. doi: 10.1038/314235a0. [DOI] [PubMed] [Google Scholar]
- Jencks W. P. Binding energy, specificity, and enzymic catalysis: the circe effect. Adv Enzymol Relat Areas Mol Biol. 1975;43:219–410. doi: 10.1002/9780470122884.ch4. [DOI] [PubMed] [Google Scholar]
- Lowe D. M., Fersht A. R., Wilkinson A. J., Carter P., Winter G. Probing histidine-substrate interactions in tyrosyl-tRNA synthetase using asparagine and glutamine replacements. Biochemistry. 1985 Sep 10;24(19):5106–5109. doi: 10.1021/bi00340a022. [DOI] [PubMed] [Google Scholar]
- Monteilhet C., Blow D. M., Brick P. Interaction of crystalline tyrosyl-tRNA synthetase with adenosine, adenosine monophosphate, adenosine triphosphate and pyrophosphate in the presence of tyrosinol. J Mol Biol. 1984 Mar 15;173(4):477–485. doi: 10.1016/0022-2836(84)90392-9. [DOI] [PubMed] [Google Scholar]
- Page M. I., Jencks W. P. Entropic contributions to rate accelerations in enzymic and intramolecular reactions and the chelate effect. Proc Natl Acad Sci U S A. 1971 Aug;68(8):1678–1683. doi: 10.1073/pnas.68.8.1678. [DOI] [PMC free article] [PubMed] [Google Scholar]
- Waye M. M., Winter G., Wilkinson A. J., Fersht A. R. Deletion mutagenesis using an 'M13 splint': the N-terminal structural domain of tyrosyl-tRNA synthetase (B. stearothermophilus) catalyses the formation of tyrosyl adenylate. EMBO J. 1983;2(10):1827–1829. doi: 10.1002/j.1460-2075.1983.tb01665.x. [DOI] [PMC free article] [PubMed] [Google Scholar]
- Wilkinson A. J., Fersht A. R., Blow D. M., Winter G. Site-directed mutagenesis as a probe of enzyme structure and catalysis: tyrosyl-tRNA synthetase cysteine-35 to glycine-35 mutation. Biochemistry. 1983 Jul 19;22(15):3581–3586. doi: 10.1021/bi00284a007. [DOI] [PubMed] [Google Scholar]
- Winter G., Fersht A. R., Wilkinson A. J., Zoller M., Smith M. Redesigning enzyme structure by site-directed mutagenesis: tyrosyl tRNA synthetase and ATP binding. Nature. 1982 Oct 21;299(5885):756–758. doi: 10.1038/299756a0. [DOI] [PubMed] [Google Scholar]