Abstract
The Ca2+-dependent cytolytic activity of isolated T-lymphocyte granules was purified to apparent homogeneity by high-salt extraction, gel filtration, and ion-exchange chromatography. The lytic activity resided in a 72- to 75-kDa protein of cytolytic granules. Incubation of the isolated protein with erythrocytes in the presence of Ca2+ ions resulted in hemolysis and the formation of membrane lesions of 160 A in diameter, corresponding in size and morphology to membrane lesions formed on target cells by cloned, intact natural killer (NK) and cytolytic T lymphocytes. Hence, the 75-kDa granule protein is identified as monomeric perforin 1 (P1), postulated previously from the analysis of membrane lesions formed during NK and T-cell-mediated cytolysis. P1-mediated hemolysis is Ca2+-dependent and is inhibited by Zn2+ ions. Lysis is accompanied by the polymerization of P1 to membrane-associated tubular complexes (poly-P1) that form large transmembrane pores. P1 causes a rapid membrane depolarization of J774 cells in the presence of Ca2+. Purified P1 also induces transmembrane monovalent and divalent ion flow across lipid vesicles only in the presence of Ca2+. Whole-cell patch-clamp recordings of S49 lymphoma cells show a P1-dependent inward membrane current flow in the presence but not in the absence of Ca2+. The current increase can be dissected as a summation of discrete current events, indicative of formation of functional channels by polymerization of P1.
Full text
PDF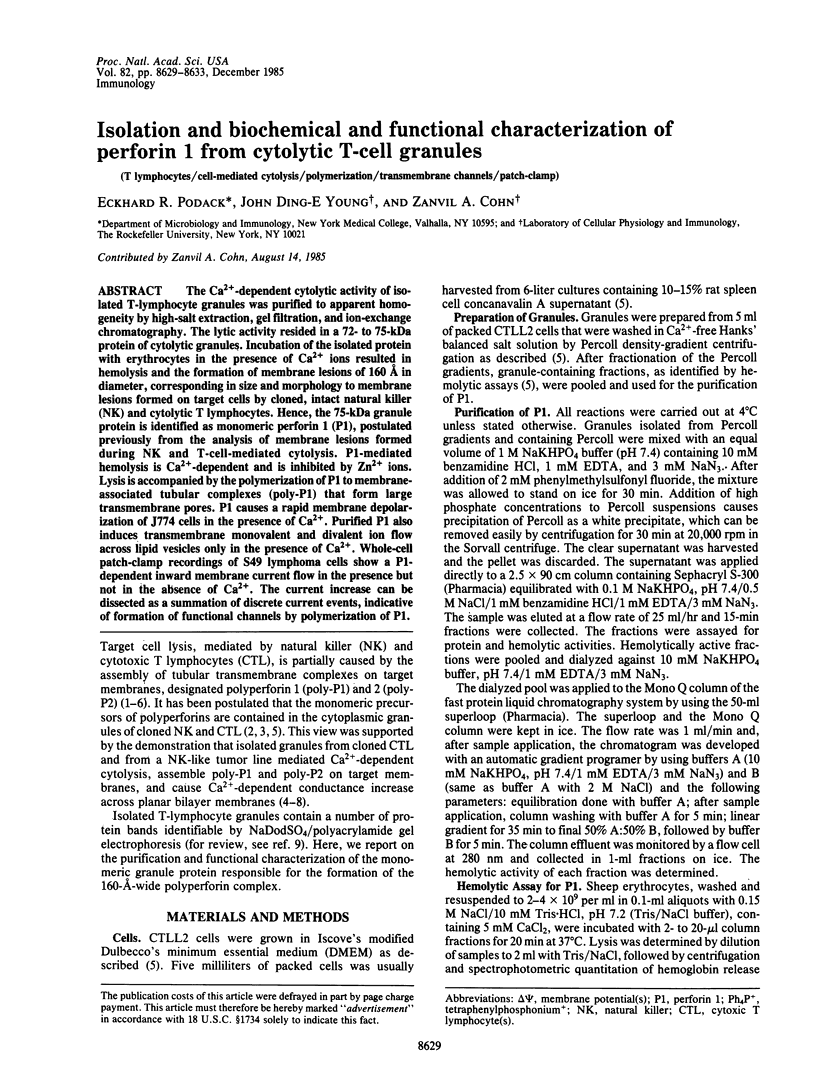
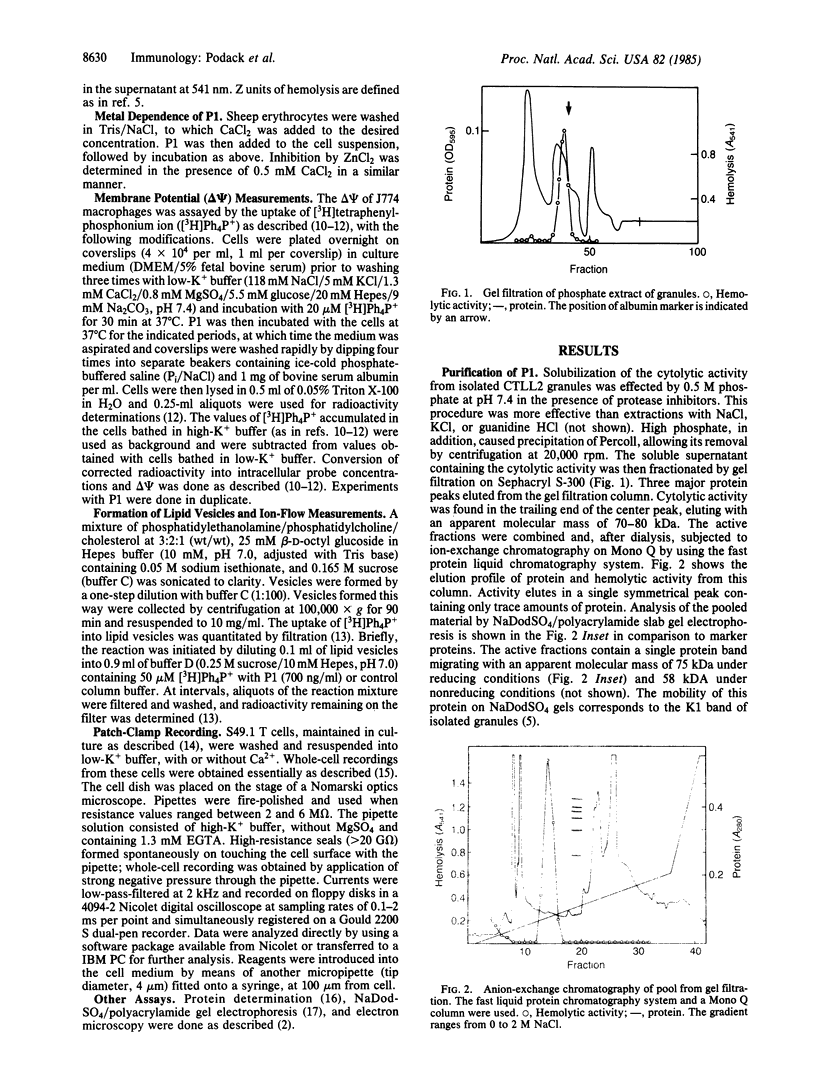
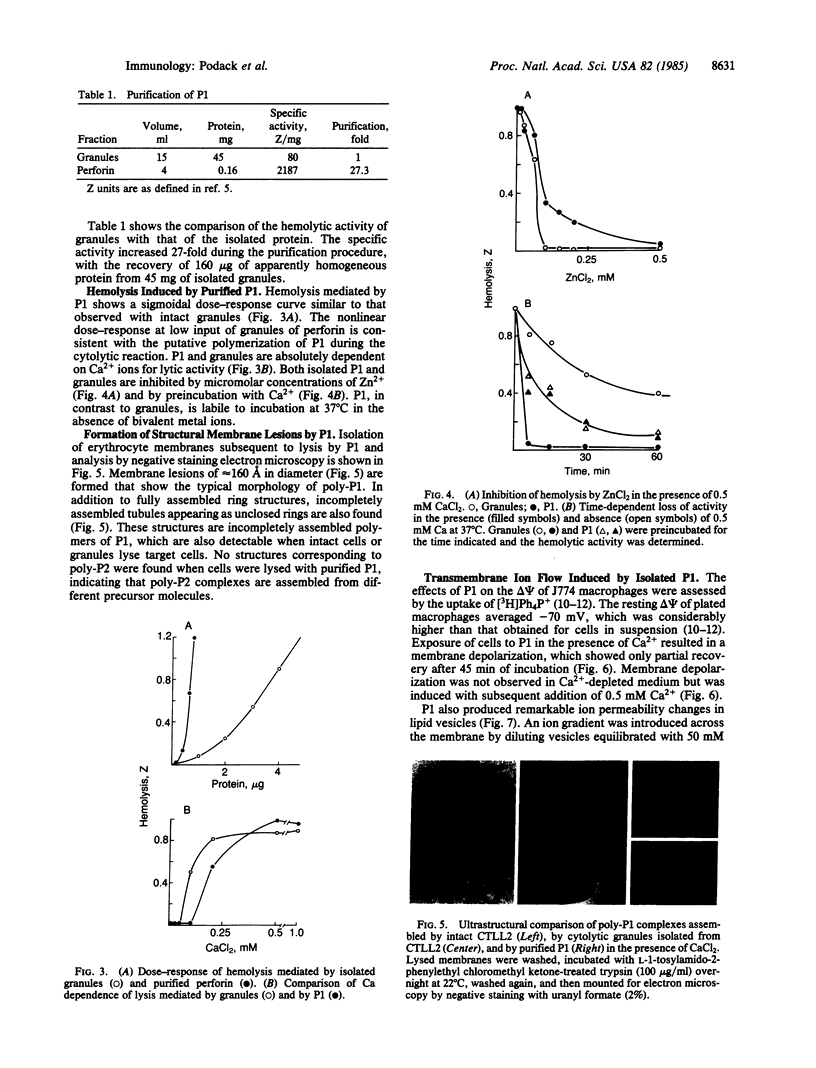
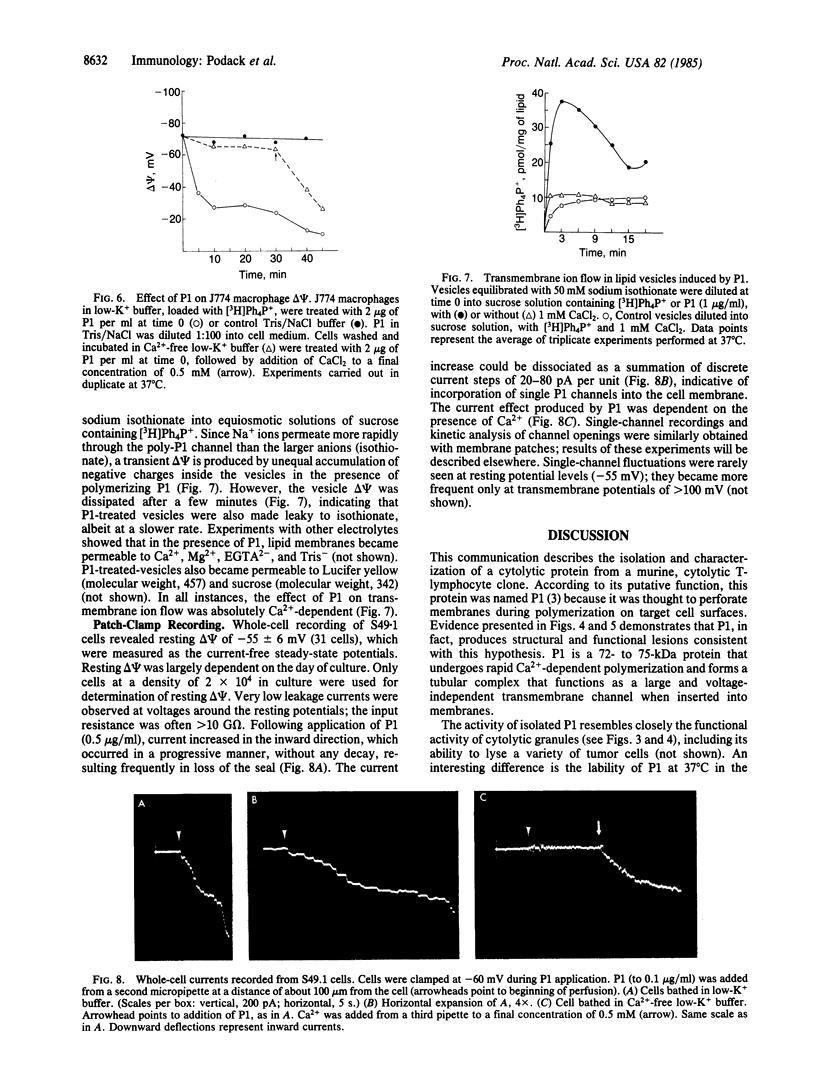
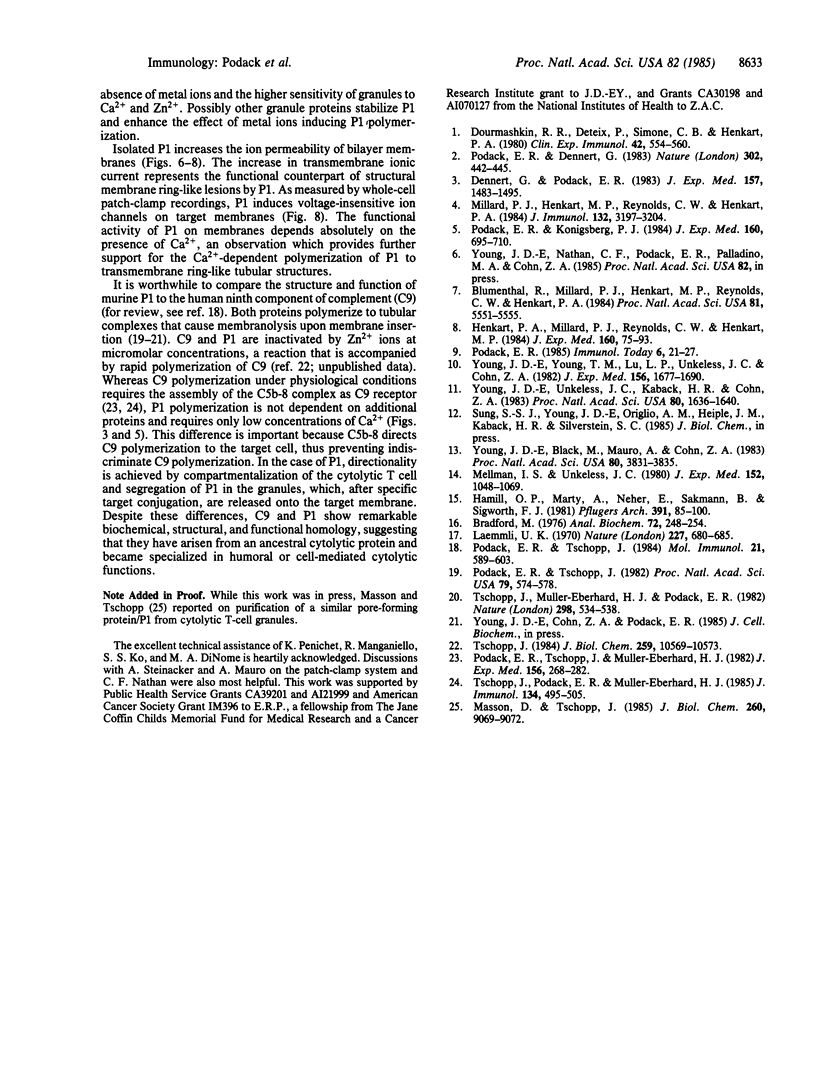
Images in this article
Selected References
These references are in PubMed. This may not be the complete list of references from this article.
- Blumenthal R., Millard P. J., Henkart M. P., Reynolds C. W., Henkart P. A. Liposomes as targets for granule cytolysin from cytotoxic large granular lymphocyte tumors. Proc Natl Acad Sci U S A. 1984 Sep;81(17):5551–5555. doi: 10.1073/pnas.81.17.5551. [DOI] [PMC free article] [PubMed] [Google Scholar]
- Bradford M. M. A rapid and sensitive method for the quantitation of microgram quantities of protein utilizing the principle of protein-dye binding. Anal Biochem. 1976 May 7;72:248–254. doi: 10.1016/0003-2697(76)90527-3. [DOI] [PubMed] [Google Scholar]
- Dennert G., Podack E. R. Cytolysis by H-2-specific T killer cells. Assembly of tubular complexes on target membranes. J Exp Med. 1983 May 1;157(5):1483–1495. doi: 10.1084/jem.157.5.1483. [DOI] [PMC free article] [PubMed] [Google Scholar]
- Dourmashkin R. R., Deteix P., Simone C. B., Henkart P. Electron microscopic demonstration of lesions in target cell membranes associated with antibody-dependent cellular cytotoxicity. Clin Exp Immunol. 1980 Dec;42(3):554–560. [PMC free article] [PubMed] [Google Scholar]
- Hamill O. P., Marty A., Neher E., Sakmann B., Sigworth F. J. Improved patch-clamp techniques for high-resolution current recording from cells and cell-free membrane patches. Pflugers Arch. 1981 Aug;391(2):85–100. doi: 10.1007/BF00656997. [DOI] [PubMed] [Google Scholar]
- Henkart P. A., Millard P. J., Reynolds C. W., Henkart M. P. Cytolytic activity of purified cytoplasmic granules from cytotoxic rat large granular lymphocyte tumors. J Exp Med. 1984 Jul 1;160(1):75–93. doi: 10.1084/jem.160.1.75. [DOI] [PMC free article] [PubMed] [Google Scholar]
- Laemmli U. K. Cleavage of structural proteins during the assembly of the head of bacteriophage T4. Nature. 1970 Aug 15;227(5259):680–685. doi: 10.1038/227680a0. [DOI] [PubMed] [Google Scholar]
- Masson D., Tschopp J. Isolation of a lytic, pore-forming protein (perforin) from cytolytic T-lymphocytes. J Biol Chem. 1985 Aug 5;260(16):9069–9072. [PubMed] [Google Scholar]
- Mellman I. S., Unkeless J. C. Purificaton of a functional mouse Fc receptor through the use of a monoclonal antibody. J Exp Med. 1980 Oct 1;152(4):1048–1069. doi: 10.1084/jem.152.4.1048. [DOI] [PMC free article] [PubMed] [Google Scholar]
- Millard P. J., Henkart M. P., Reynolds C. W., Henkart P. A. Purification and properties of cytoplasmic granules from cytotoxic rat LGL tumors. J Immunol. 1984 Jun;132(6):3197–3204. [PubMed] [Google Scholar]
- Podack E. R., Dennert G. Assembly of two types of tubules with putative cytolytic function by cloned natural killer cells. 1983 Mar 31-Apr 6Nature. 302(5907):442–445. doi: 10.1038/302442a0. [DOI] [PubMed] [Google Scholar]
- Podack E. R., Konigsberg P. J. Cytolytic T cell granules. Isolation, structural, biochemical, and functional characterization. J Exp Med. 1984 Sep 1;160(3):695–710. doi: 10.1084/jem.160.3.695. [DOI] [PMC free article] [PubMed] [Google Scholar]
- Podack E. R., Tschoop J., Müller-Eberhard H. J. Molecular organization of C9 within the membrane attack complex of complement. Induction of circular C9 polymerization by the C5b-8 assembly. J Exp Med. 1982 Jul 1;156(1):268–282. doi: 10.1084/jem.156.1.268. [DOI] [PMC free article] [PubMed] [Google Scholar]
- Podack E. R., Tschopp J. Membrane attack by complement. Mol Immunol. 1984 Jul;21(7):589–603. doi: 10.1016/0161-5890(84)90044-0. [DOI] [PubMed] [Google Scholar]
- Podack E. R., Tschopp J. Polymerization of the ninth component of complement (C9): formation of poly(C9) with a tubular ultrastructure resembling the membrane attack complex of complement. Proc Natl Acad Sci U S A. 1982 Jan;79(2):574–578. doi: 10.1073/pnas.79.2.574. [DOI] [PMC free article] [PubMed] [Google Scholar]
- Tschopp J. Circular polymerization of the membranolytic ninth component of complement. Dependence on metal ions. J Biol Chem. 1984 Aug 25;259(16):10569–10573. [PubMed] [Google Scholar]
- Tschopp J., Müller-Eberhard H. J., Podack E. R. Formation of transmembrane tubules by spontaneous polymerization of the hydrophilic complement protein C9. Nature. 1982 Aug 5;298(5874):534–538. doi: 10.1038/298534a0. [DOI] [PubMed] [Google Scholar]
- Tschopp J., Podack E. R., Müller-Eberhard H. J. The membrane attack complex of complement: C5b-8 complex as accelerator of C9 polymerization. J Immunol. 1985 Jan;134(1):495–499. [PubMed] [Google Scholar]
- Young J. D., Blake M., Mauro A., Cohn Z. A. Properties of the major outer membrane protein from Neisseria gonorrhoeae incorporated into model lipid membranes. Proc Natl Acad Sci U S A. 1983 Jun;80(12):3831–3835. doi: 10.1073/pnas.80.12.3831. [DOI] [PMC free article] [PubMed] [Google Scholar]
- Young J. D., Unkeless J. C., Kaback H. R., Cohn Z. A. Mouse macrophage Fc receptor for IgG gamma 2b/gamma 1 in artificial and plasma membrane vesicles functions as a ligand-dependent ionophore. Proc Natl Acad Sci U S A. 1983 Mar;80(6):1636–1640. doi: 10.1073/pnas.80.6.1636. [DOI] [PMC free article] [PubMed] [Google Scholar]
- Young J. D., Young T. M., Lu L. P., Unkeless J. C., Cohn Z. A. Characterization of a membrane pore-forming protein from Entamoeba histolytica. J Exp Med. 1982 Dec 1;156(6):1677–1690. doi: 10.1084/jem.156.6.1677. [DOI] [PMC free article] [PubMed] [Google Scholar]