Abstract
The permeability of Ca channels to various foreign cations has been investigated in the absence of external Ca2+. All physiological metal cations are clearly permeant, including Mg2+. The large organic cation n-butylamine+ is sparingly permeant or impermeant, but its larger derivative 1,4-diaminobutane2+ is highly permeant. Among the cations of the methylated ammonium series, permeability diminishes in a graded fashion as ion size increases. Tetramethylammonium, the largest cation found to be permeant, has a diameter of about 6 A; hence, the aqueous pore of the Ca channel at its narrowest point can be no smaller. That the pore is so large strengthens our view that, under physiologic conditions, the high selectivity of Ca channels is due to selective binding of Ca2+ rather than to rejection of other cations by, for example, a sieving mechanism.
Full text
PDF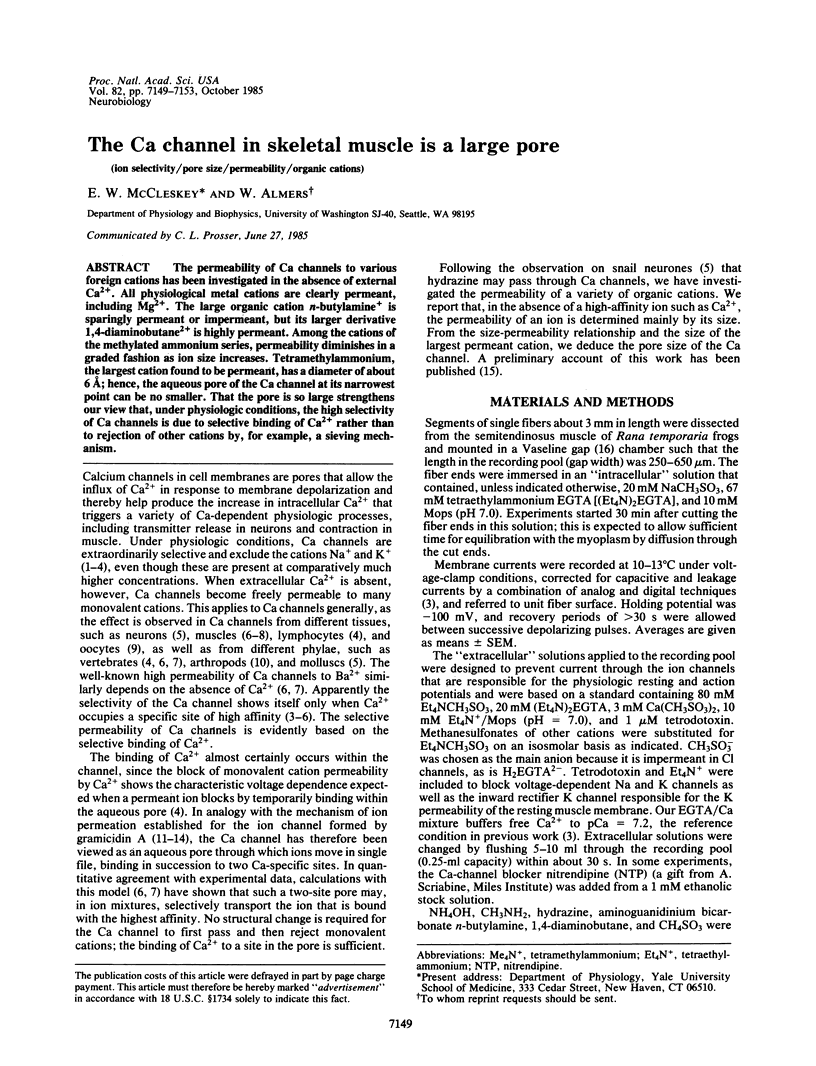
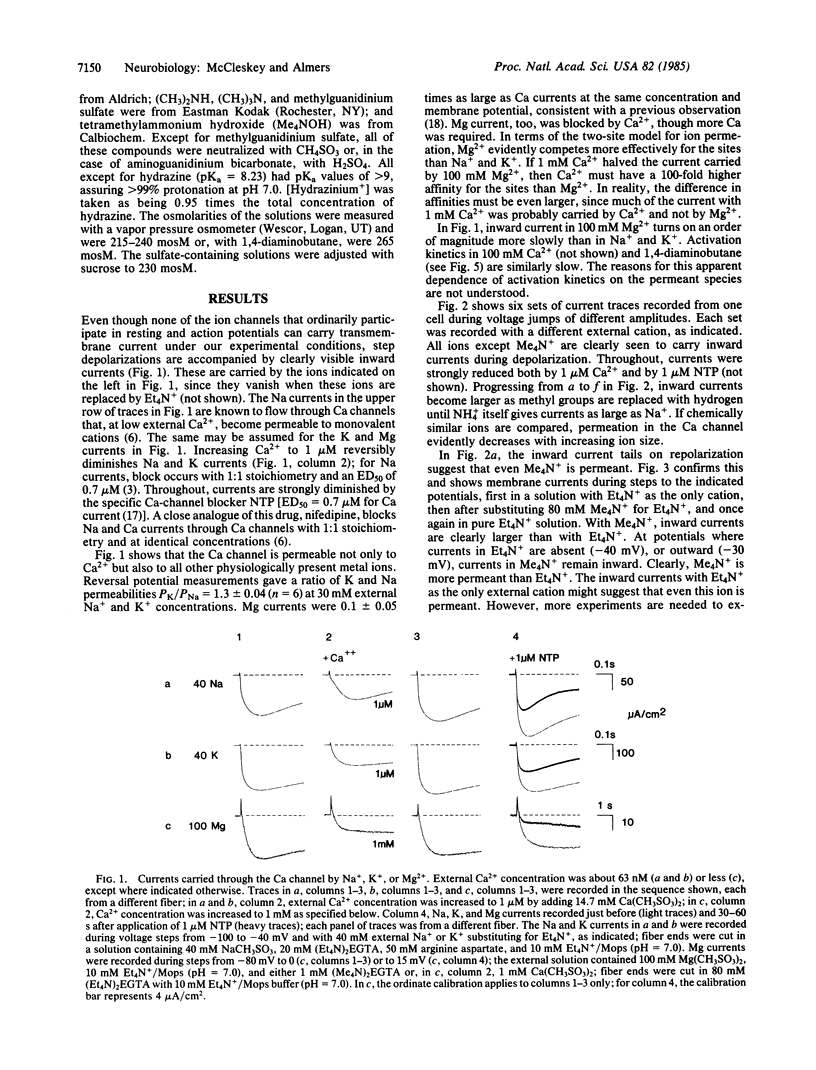
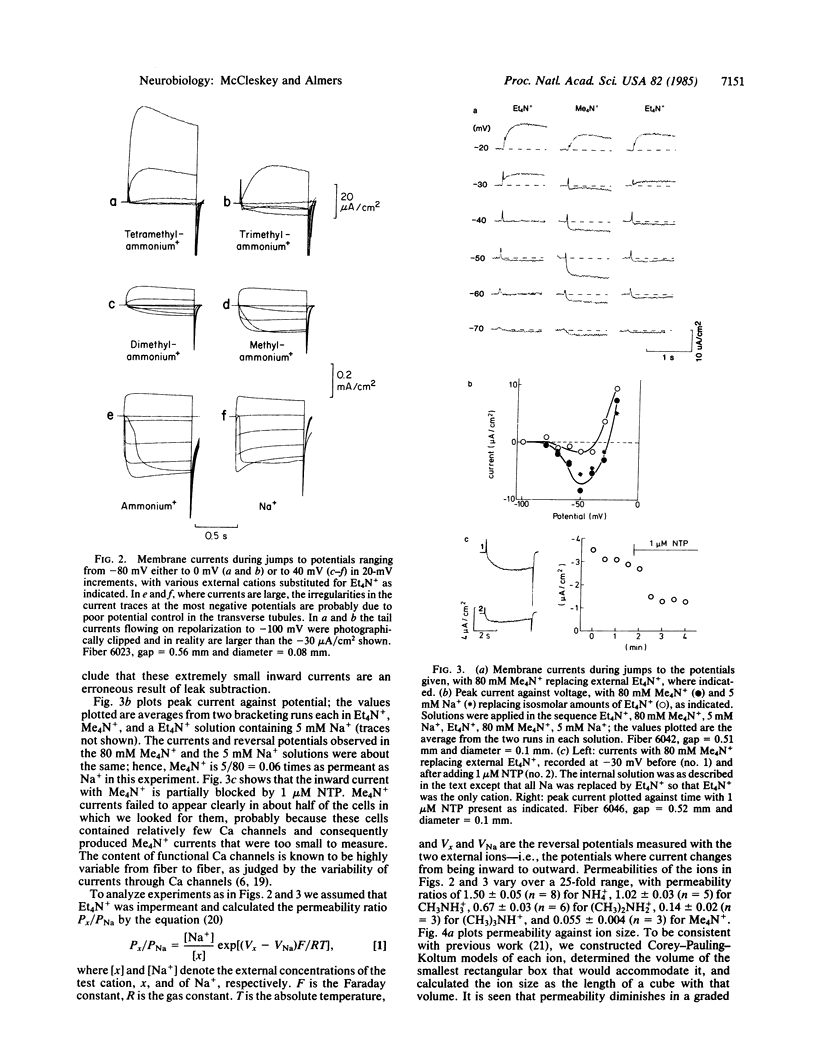
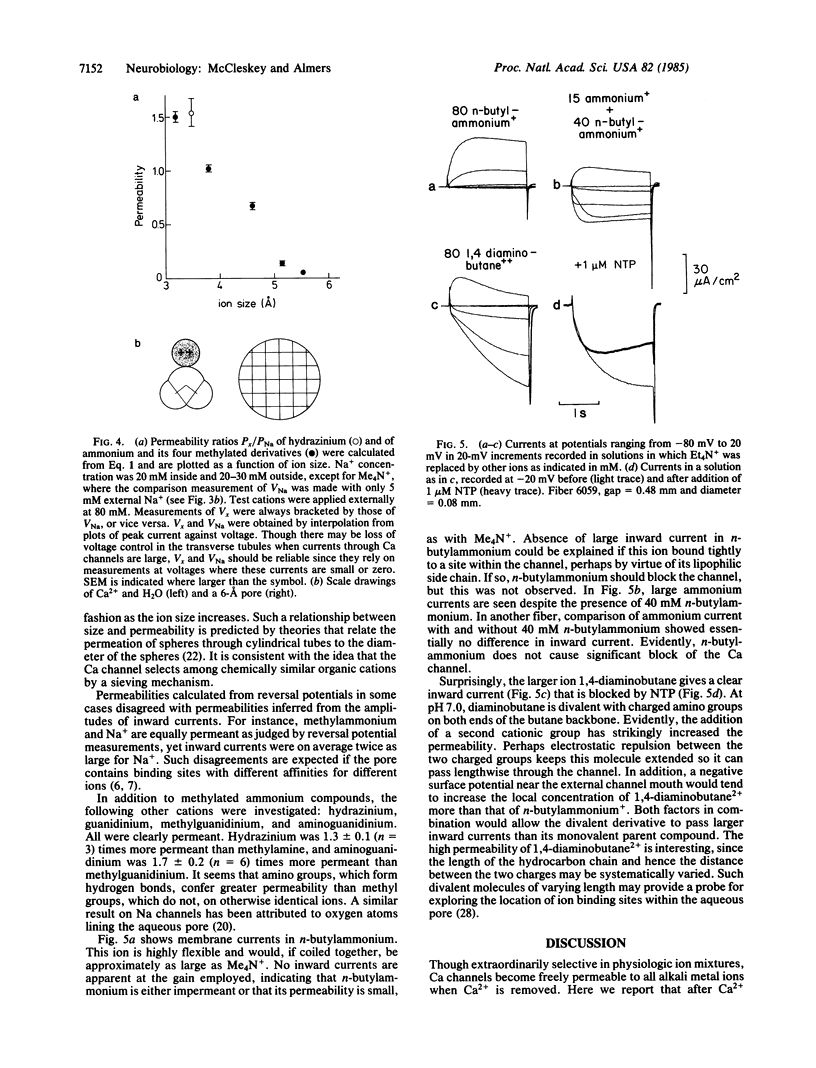
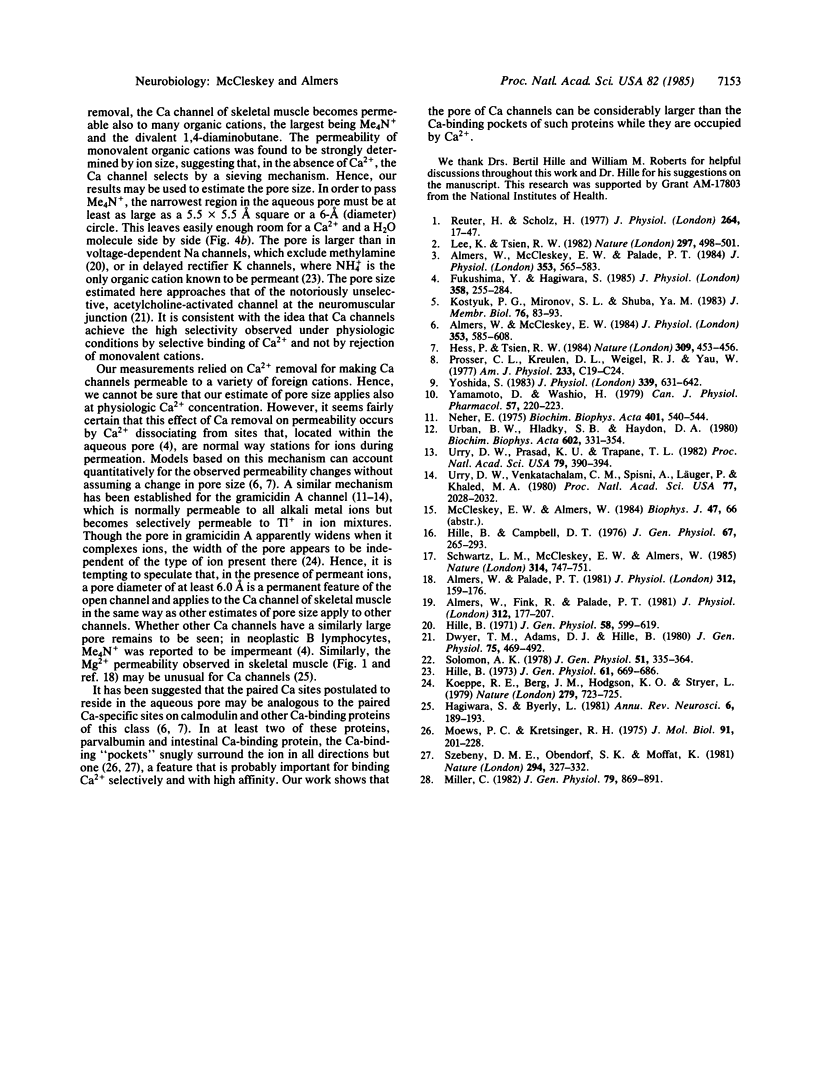
Selected References
These references are in PubMed. This may not be the complete list of references from this article.
- Almers W., Fink R., Palade P. T. Calcium depletion in frog muscle tubules: the decline of calcium current under maintained depolarization. J Physiol. 1981 Mar;312:177–207. doi: 10.1113/jphysiol.1981.sp013623. [DOI] [PMC free article] [PubMed] [Google Scholar]
- Almers W., McCleskey E. W. Non-selective conductance in calcium channels of frog muscle: calcium selectivity in a single-file pore. J Physiol. 1984 Aug;353:585–608. doi: 10.1113/jphysiol.1984.sp015352. [DOI] [PMC free article] [PubMed] [Google Scholar]
- Almers W., McCleskey E. W., Palade P. T. A non-selective cation conductance in frog muscle membrane blocked by micromolar external calcium ions. J Physiol. 1984 Aug;353:565–583. doi: 10.1113/jphysiol.1984.sp015351. [DOI] [PMC free article] [PubMed] [Google Scholar]
- Almers W., Palade P. T. Slow calcium and potassium currents across frog muscle membrane: measurements with a vaseline-gap technique. J Physiol. 1981 Mar;312:159–176. doi: 10.1113/jphysiol.1981.sp013622. [DOI] [PMC free article] [PubMed] [Google Scholar]
- Dwyer T. M., Adams D. J., Hille B. The permeability of the endplate channel to organic cations in frog muscle. J Gen Physiol. 1980 May;75(5):469–492. doi: 10.1085/jgp.75.5.469. [DOI] [PMC free article] [PubMed] [Google Scholar]
- Fukushima Y., Hagiwara S. Currents carried by monovalent cations through calcium channels in mouse neoplastic B lymphocytes. J Physiol. 1985 Jan;358:255–284. doi: 10.1113/jphysiol.1985.sp015550. [DOI] [PMC free article] [PubMed] [Google Scholar]
- Hess P., Tsien R. W. Mechanism of ion permeation through calcium channels. 1984 May 31-Jun 6Nature. 309(5967):453–456. doi: 10.1038/309453a0. [DOI] [PubMed] [Google Scholar]
- Hille B., Campbell D. T. An improved vaseline gap voltage clamp for skeletal muscle fibers. J Gen Physiol. 1976 Mar;67(3):265–293. doi: 10.1085/jgp.67.3.265. [DOI] [PMC free article] [PubMed] [Google Scholar]
- Hille B. Potassium channels in myelinated nerve. Selective permeability to small cations. J Gen Physiol. 1973 Jun;61(6):669–686. doi: 10.1085/jgp.61.6.669. [DOI] [PMC free article] [PubMed] [Google Scholar]
- Hille B. The permeability of the sodium channel to organic cations in myelinated nerve. J Gen Physiol. 1971 Dec;58(6):599–619. doi: 10.1085/jgp.58.6.599. [DOI] [PMC free article] [PubMed] [Google Scholar]
- Koeppe R. E., 2nd, Berg J. M., Hodgson K. O., Stryer L. Gramicidin A crystals contain two cation binding sites per channel. Nature. 1979 Jun 21;279(5715):723–725. doi: 10.1038/279723a0. [DOI] [PubMed] [Google Scholar]
- Lee K. S., Tsien R. W. Reversal of current through calcium channels in dialysed single heart cells. Nature. 1982 Jun 10;297(5866):498–501. doi: 10.1038/297498a0. [DOI] [PubMed] [Google Scholar]
- Miller C. Bis-quaternary ammonium blockers as structural probes of the sarcoplasmic reticulum K+ channel. J Gen Physiol. 1982 May;79(5):869–891. doi: 10.1085/jgp.79.5.869. [DOI] [PMC free article] [PubMed] [Google Scholar]
- Moews P. C., Kretsinger R. H. Refinement of the structure of carp muscle calcium-binding parvalbumin by model building and difference Fourier analysis. J Mol Biol. 1975 Jan 15;91(2):201–225. doi: 10.1016/0022-2836(75)90160-6. [DOI] [PubMed] [Google Scholar]
- Prosser C. L., Kreulen D. L., Weigel R. J., Yau W. Prolonged potentials in gastrointestinal muscles induced by calcium chelation. Am J Physiol. 1977 Jul;233(1):C19–C24. doi: 10.1152/ajpcell.1977.233.1.C19. [DOI] [PubMed] [Google Scholar]
- Reuter H., Scholz H. A study of the ion selectivity and the kinetic properties of the calcium dependent slow inward current in mammalian cardiac muscle. J Physiol. 1977 Jan;264(1):17–47. doi: 10.1113/jphysiol.1977.sp011656. [DOI] [PMC free article] [PubMed] [Google Scholar]
- Schwartz L. M., McCleskey E. W., Almers W. Dihydropyridine receptors in muscle are voltage-dependent but most are not functional calcium channels. 1985 Apr 25-May 1Nature. 314(6013):747–751. doi: 10.1038/314747a0. [DOI] [PubMed] [Google Scholar]
- Solomon A. K. Characterization of biological membranes by equivalent pores. J Gen Physiol. 1968 May 1;51(5):335–364. [PMC free article] [PubMed] [Google Scholar]
- Szebenyi D. M., Obendorf S. K., Moffat K. Structure of vitamin D-dependent calcium-binding protein from bovine intestine. Nature. 1981 Nov 26;294(5839):327–332. doi: 10.1038/294327a0. [DOI] [PubMed] [Google Scholar]
- Urban B. W., Hladky S. B., Haydon D. A. Ion movements in gramicidin pores. An example of single-file transport. Biochim Biophys Acta. 1980 Nov 4;602(2):331–354. doi: 10.1016/0005-2736(80)90316-8. [DOI] [PubMed] [Google Scholar]
- Urry D. W., Prasad K. U., Trapane T. L. Location of monovalent cation binding sites in the gramicidin channel. Proc Natl Acad Sci U S A. 1982 Jan;79(2):390–394. doi: 10.1073/pnas.79.2.390. [DOI] [PMC free article] [PubMed] [Google Scholar]
- Urry D. W., Venkatachalam C. M., Spisni A., Läuger P., Khaled M. A. Rate theory calculation of gramicidin single-channel currents using NMR-derived rate constants. Proc Natl Acad Sci U S A. 1980 Apr;77(4):2028–2032. doi: 10.1073/pnas.77.4.2028. [DOI] [PMC free article] [PubMed] [Google Scholar]
- Yamamoto D., Washio H. Permeation of sodium through calcium channels of an insect muscle membrane. Can J Physiol Pharmacol. 1979 Feb;57(2):220–222. doi: 10.1139/y79-033. [DOI] [PubMed] [Google Scholar]
- Yoshida S. Permeation of divalent and monovalent cations through the ovarian oocyte membrane of the mouse. J Physiol. 1983 Jun;339:631–642. doi: 10.1113/jphysiol.1983.sp014739. [DOI] [PMC free article] [PubMed] [Google Scholar]