Abstract
The movement of labeled concanavalin A (Con A) receptors in reconstituted human erythrocyte membranes was observed directly in an electron microscope, using an environmental stage that kept the sample fully hydrated at all experimental temperatures. Human erythrocyte membrane ghosts were spread on the air/water interface in a Langmuir trough. The surface monolayer film contained most native proteins and lipids of the erythrocyte membrane. The Con A receptors in the film were labeled with Con A-conjugated, 25-nm-diameter gold microspheres. Unsupported bilayer membranes were reconstituted by dipping a 1000-mesh grid through the labeled surface film. The reconstituted membrane samples were observed under low beam current and photographed by timed exposures with sensitive x-ray films. The total radiation per exposure was kept below the damage threshold of 5 X 10(-4) coulomb/cm2. The Con A-gold labels were observed to move in unison within local areas (domains) of the reconstituted membrane. The size of the domains and the velocity of the labels were measured as functions of temperature. The typical domain size was 10 micron2 and the typical velocity of the labels was 7 nm/sec. The minimum domain size and velocity were found at 17 degrees-28 degrees C. Reduction of the amount of cholesterol in the precursor erythrocyte membrane caused the domain velocity at 7 degrees C to decrease and the domain size to increase; the opposite effect was observed with cholesterol enrichment. The results indicate that the components of the erythrocyte membrane tended to form moving domains and that the motion was related to lipid phase separation in the bilayer.
Full text
PDF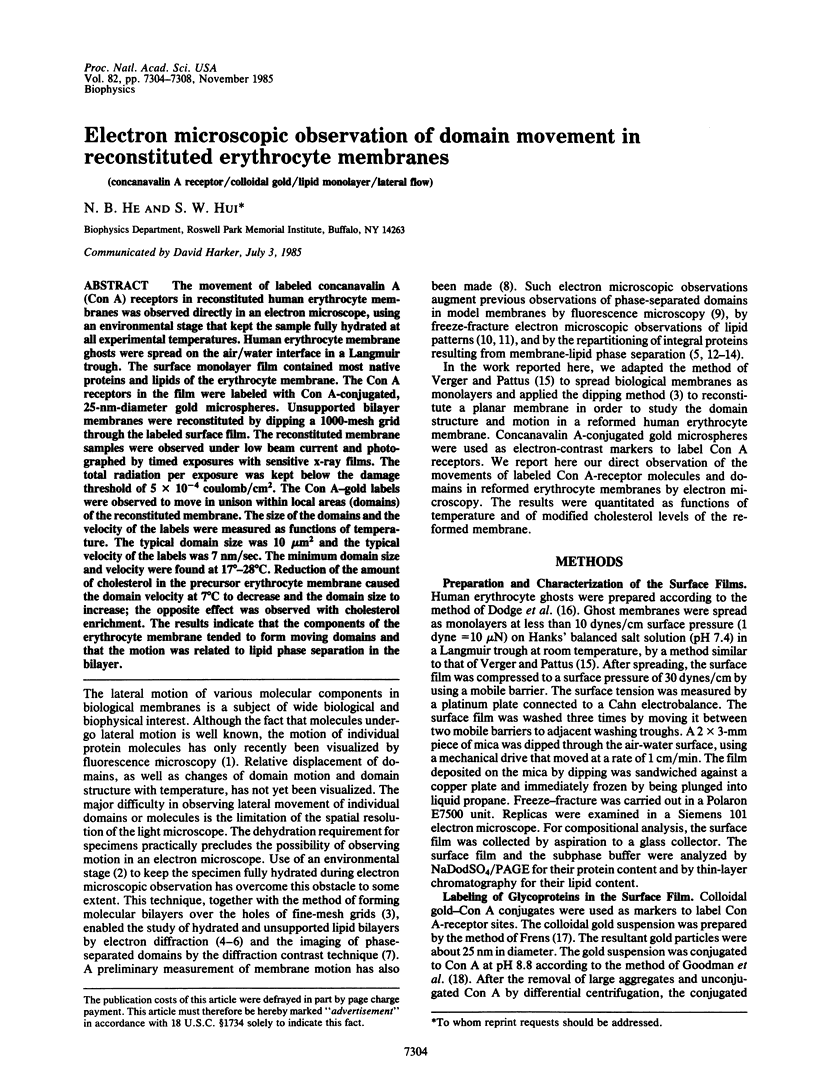
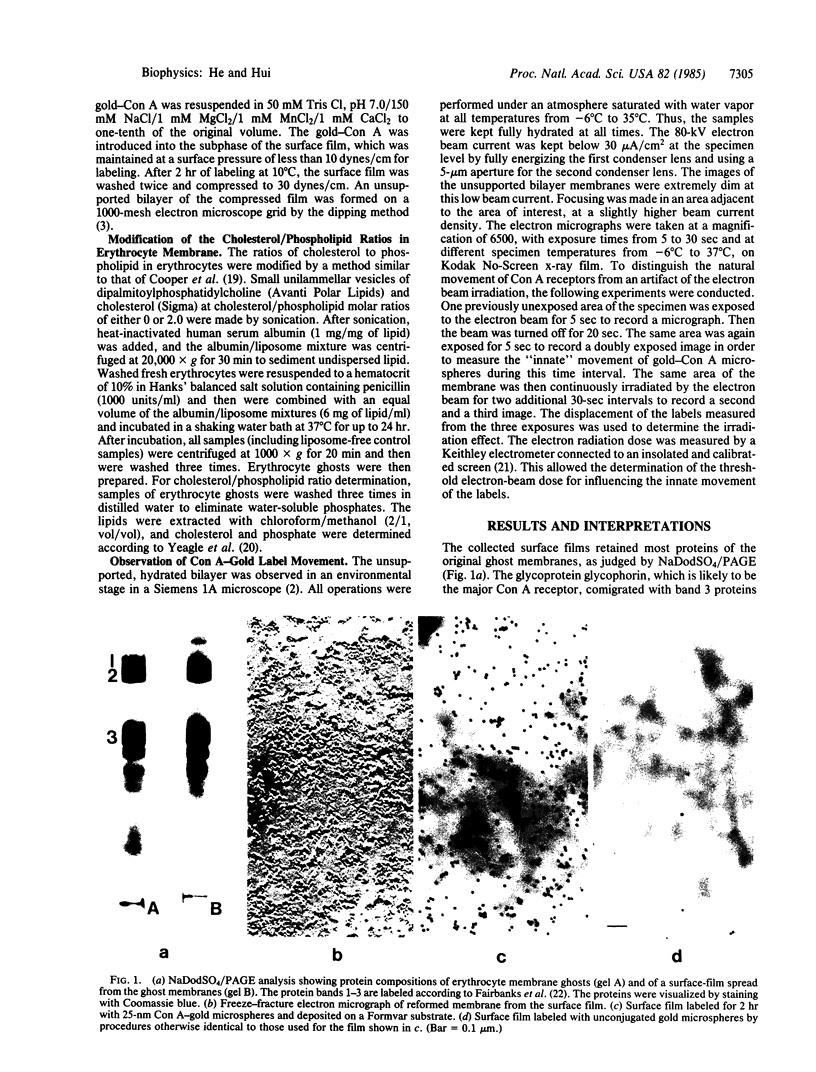
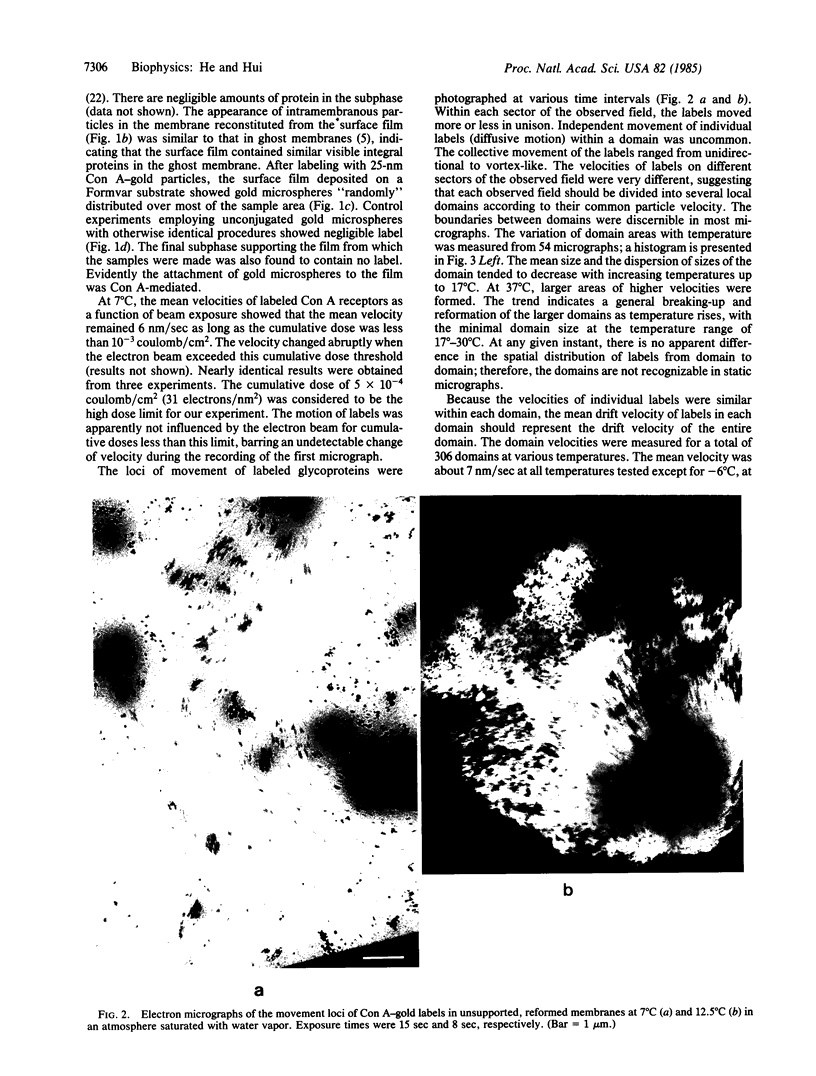
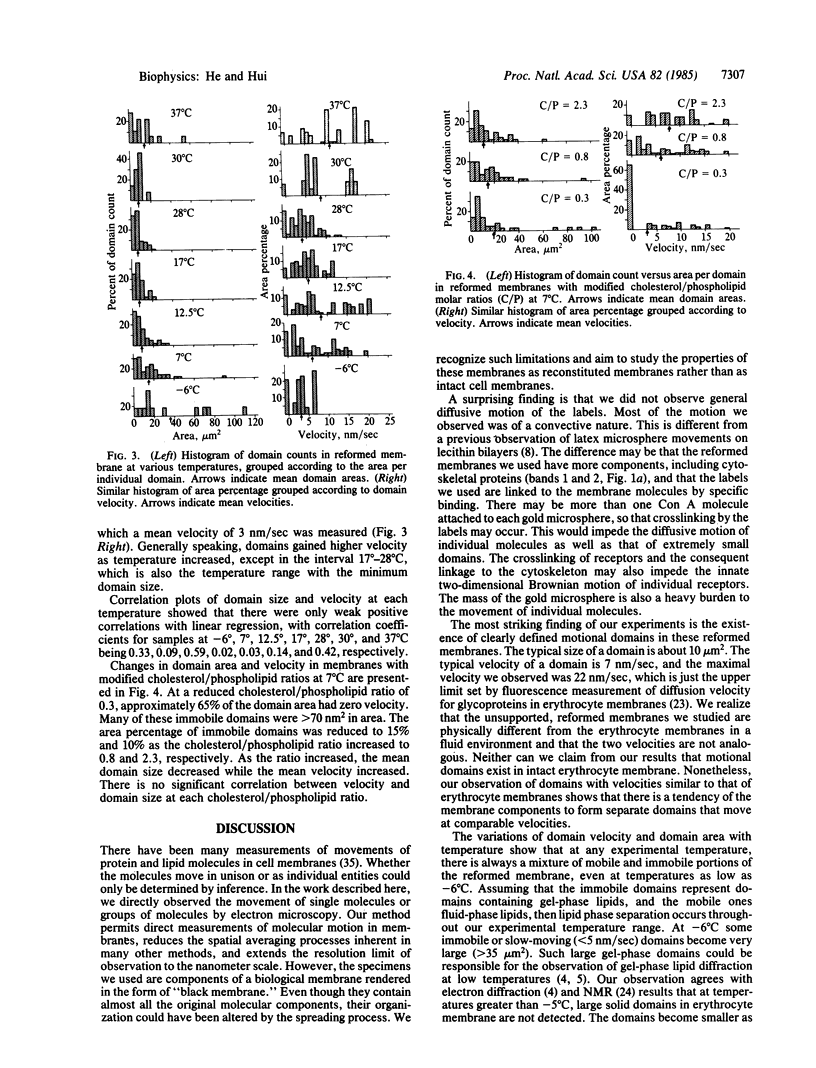
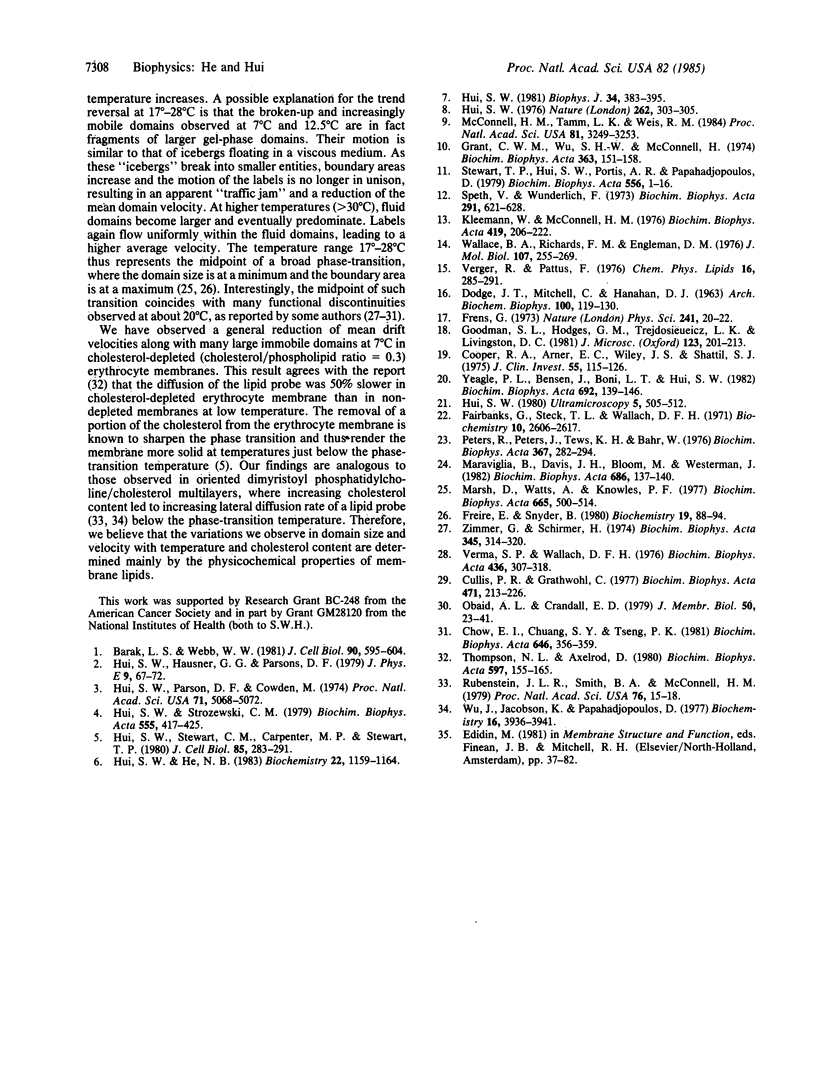
Images in this article
Selected References
These references are in PubMed. This may not be the complete list of references from this article.
- Barak L. S., Webb W. W. Fluorescent low density lipoprotein for observation of dynamics of individual receptor complexes on cultured human fibroblasts. J Cell Biol. 1981 Sep;90(3):595–604. doi: 10.1083/jcb.90.3.595. [DOI] [PMC free article] [PubMed] [Google Scholar]
- Chow E. I., Chuang S. Y., Tseng P. K. Detection of a phase transition in red cell membranes using positronium as a probe. Biochim Biophys Acta. 1981 Aug 20;646(2):356–359. doi: 10.1016/0005-2736(81)90344-8. [DOI] [PubMed] [Google Scholar]
- Cooper R. A., Arner E. C., Wiley J. S., Shattil S. J. Modification of red cell membrane structure by cholesterol-rich lipid dispersions. A model for the primary spur cell defect. J Clin Invest. 1975 Jan;55(1):115–126. doi: 10.1172/JCI107901. [DOI] [PMC free article] [PubMed] [Google Scholar]
- Cullis P. R., Grathwohl C. Hydrocarbon phase transitions and lipid-protein interactions in the erythrocyte membrane. A 31P NMR and fluorescence study. Biochim Biophys Acta. 1977 Dec 1;471(2):213–226. doi: 10.1016/0005-2736(77)90251-6. [DOI] [PubMed] [Google Scholar]
- DODGE J. T., MITCHELL C., HANAHAN D. J. The preparation and chemical characteristics of hemoglobin-free ghosts of human erythrocytes. Arch Biochem Biophys. 1963 Jan;100:119–130. doi: 10.1016/0003-9861(63)90042-0. [DOI] [PubMed] [Google Scholar]
- Fairbanks G., Steck T. L., Wallach D. F. Electrophoretic analysis of the major polypeptides of the human erythrocyte membrane. Biochemistry. 1971 Jun 22;10(13):2606–2617. doi: 10.1021/bi00789a030. [DOI] [PubMed] [Google Scholar]
- Freire E., Snyder B. Estimation of the lateral distribution of molecules in two-component lipid bilayers. Biochemistry. 1980 Jan 8;19(1):88–94. doi: 10.1021/bi00542a014. [DOI] [PubMed] [Google Scholar]
- Goodman S. L., Hodges G. M., Trejdosiewicz L. K., Livingston D. C. Colloidal gold markers and probes for routine application in microscopy. J Microsc. 1981 Aug;123(Pt 2):201–213. doi: 10.1111/j.1365-2818.1981.tb01295.x. [DOI] [PubMed] [Google Scholar]
- Grant C. W., Wu S. H., McConnell H. M. Lateral phase separations in binary lipid mixtures: correlation between spin label and freeze-fracture electron microscopic studies. Biochim Biophys Acta. 1974 Sep 6;363(2):151–158. doi: 10.1016/0005-2736(74)90055-8. [DOI] [PubMed] [Google Scholar]
- Hui S. W. Direct measurement of membrane motion and fluidity by electron microscopy. Nature. 1976 Jul 22;262(5566):303–305. doi: 10.1038/262303a0. [DOI] [PubMed] [Google Scholar]
- Hui S. W. Geometry of phase-separated domains in phospholipid bilayers by diffraction-contrast electron microscopy. Biophys J. 1981 Jun;34(3):383–395. doi: 10.1016/S0006-3495(81)84857-6. [DOI] [PMC free article] [PubMed] [Google Scholar]
- Hui S. W., He N. B. Molecular organization in cholesterol-lecithin bilayers by X-ray and electron diffraction measurements. Biochemistry. 1983 Mar 1;22(5):1159–1164. doi: 10.1021/bi00274a026. [DOI] [PubMed] [Google Scholar]
- Hui S. W., Parsons D. F., Cowden M. Electron diffraction of wet phospholipid bilayers. Proc Natl Acad Sci U S A. 1974 Dec;71(12):5068–5072. doi: 10.1073/pnas.71.12.5068. [DOI] [PMC free article] [PubMed] [Google Scholar]
- Hui S. W. Radiation damage of phosphatidylcholine bilayers effects of temperature and hydration. Ultramicroscopy. 1980;5(4):505–512. doi: 10.1016/s0304-3991(80)80007-6. [DOI] [PubMed] [Google Scholar]
- Hui S. W., Stewart C. M., Carpenter M. P., Stewart T. P. Effects of cholesterol on lipid organization in human erythrocyte membrane. J Cell Biol. 1980 May;85(2):283–291. doi: 10.1083/jcb.85.2.283. [DOI] [PMC free article] [PubMed] [Google Scholar]
- Hui S. W., Strozewski C. M. Electron diffraction studies of human erythrocyte membrane and its lipid extracts. Effects of hydration, temperature and hydrolysis. Biochim Biophys Acta. 1979 Aug 23;555(3):417–425. doi: 10.1016/0005-2736(79)90395-x. [DOI] [PubMed] [Google Scholar]
- Kleemann W., McConnell H. M. Interactions of proteins and cholesterol with lipids in bilayer membranes. Biochim Biophys Acta. 1976 Jan 21;419(2):206–222. doi: 10.1016/0005-2736(76)90347-3. [DOI] [PubMed] [Google Scholar]
- Maraviglia B., Davis J. H., Bloom M., Westerman J., Wirtz K. W. Human erythrocyte membranes are fluid down to -5 degrees C. Biochim Biophys Acta. 1982 Mar 23;686(1):137–140. doi: 10.1016/0005-2736(82)90160-2. [DOI] [PubMed] [Google Scholar]
- Marsh D., Watts A., Knowles P. F. Cooperativity of the phase transition in single- and multibilayer lipid vesicles. Biochim Biophys Acta. 1977 Mar 17;465(3):500–514. doi: 10.1016/0005-2736(77)90268-1. [DOI] [PubMed] [Google Scholar]
- McConnell H. M., Tamm L. K., Weis R. M. Periodic structures in lipid monolayer phase transitions. Proc Natl Acad Sci U S A. 1984 May;81(10):3249–3253. doi: 10.1073/pnas.81.10.3249. [DOI] [PMC free article] [PubMed] [Google Scholar]
- Obaid A. L., Crandall E. D. HCO3-/Cl- exchange across the human erythrocyte membrane: effects of pH and temperature. J Membr Biol. 1979 Oct 5;50(1):23–41. doi: 10.1007/BF01868786. [DOI] [PubMed] [Google Scholar]
- Peters R., Peters J., Tews K. H., Bähr W. A microfluorimetric study of translational diffusion in erythrocyte membranes. Biochim Biophys Acta. 1974 Nov 15;367(3):282–294. doi: 10.1016/0005-2736(74)90085-6. [DOI] [PubMed] [Google Scholar]
- Rubenstein J. L., Smith B. A., McConnell H. M. Lateral diffusion in binary mixtures of cholesterol and phosphatidylcholines. Proc Natl Acad Sci U S A. 1979 Jan;76(1):15–18. doi: 10.1073/pnas.76.1.15. [DOI] [PMC free article] [PubMed] [Google Scholar]
- Speth V., Wunderlich F. Membranes of Tetrahymena. II. Direct visualization of reversible transitions in biomembrane structure induced by temperature. Biochim Biophys Acta. 1973 Feb 16;291(3):621–628. doi: 10.1016/0005-2736(73)90467-7. [DOI] [PubMed] [Google Scholar]
- Stewart T. P., Hui S. W., Portis A. R., Jr, Papahadjopoulos D. Complex phase mixing of phosphatidylcholine and phosphatidylserine in multilamellar membrane vesicles. Biochim Biophys Acta. 1979 Sep 4;556(1):1–16. doi: 10.1016/0005-2736(79)90415-2. [DOI] [PubMed] [Google Scholar]
- Thompson N. L., Axelrod D. Reduced lateral mobility of a fluorescent lipid probe in cholesterol-depleted erythrocyte membrane. Biochim Biophys Acta. 1980 Mar 27;597(1):155–165. doi: 10.1016/0005-2736(80)90159-5. [DOI] [PubMed] [Google Scholar]
- Verger R., Pattus F. Spreading of membranes at the air/water interface. Chem Phys Lipids. 1976 Jul;16(4):285–291. doi: 10.1016/0009-3084(76)90023-2. [DOI] [PubMed] [Google Scholar]
- Verma S. P., Wallach D. F. Multiple thermotropic state transitions in erythrocyte membranes. A laser-Raman study of the CH-stretching and acoustical regions. Biochim Biophys Acta. 1976 Jun 17;436(2):307–318. doi: 10.1016/0005-2736(76)90196-6. [DOI] [PubMed] [Google Scholar]
- Wallace B. A., Richards F. M., Engelman D. M. The influence of lipid state on the planar distribution of membrane proteins in Acholeplasma laidlawii. J Mol Biol. 1976 Nov 5;107(3):255–269. doi: 10.1016/s0022-2836(76)80004-6. [DOI] [PubMed] [Google Scholar]