Abstract
The unicellular green alga Chlamydomonas reinhardtii synthesizes carbonic anhydrase in response to low levels of CO2 (i.e., air levels of CO2). This enzyme, localized predominantly in the periplasmic space of the alga (or associated with the cell wall), is an important component of the machinery required for the active accumulation of inorganic carbon by C. reinhardtii and the saturation of ribulose-1,5-bisphosphate carboxylase at low extracellular carbon concentrations. We have begun to examine the synthesis and compartmentalization of carbonic anhydrase in C. reinhardtii. The monomeric species associated with carbonic anhydrase activity is synthesized as a precursor on 80S cytoplasmic ribosomes. This precursor can be detected immunologically in the profiles of translation products when a reticulocyte lysate, cell-free system is primed with poly(A)-RNA from either air-grown C. reinhardtii or cells shifted from growth on 5% CO2 to air for 12 hr. It is not synthesized when the in vitro system is primed with poly(A)-RNA from CO2-grown algae. Since translatable RNA for the polypeptide responsible for carbonic anhydrase activity was only present in cells that experienced low levels of CO2, the adaptation process either involves the regulation of transcription of the carbonic anhydrase gene (and perhaps other genes involved in adaptation) or the post-transcriptional processing of the messenger RNA. Furthermore, the appearance of the mature polypeptide associated with carbonic anhydrase activity in the periplasmic space of C. reinhardtii is inhibited by tunicamycin, an antibiotic that prevents core glycosylation of polypeptides on the endoplasmic reticulum. Together, these results suggest that the biosynthesis of this extracellular algal enzyme involves the translation of mRNA for the carbonic anhydrase monomer on ribosomes bound to the endoplasmic reticulum, the cleavage of a signal sequence during transport of the nascent polypeptide into the lumen of the endoplasmic reticulum, and subsequent glycosylation events prior to export across the plasmalemma.
Keywords: glycosylation, tunicamycin, precursor, regulation
Full text
PDF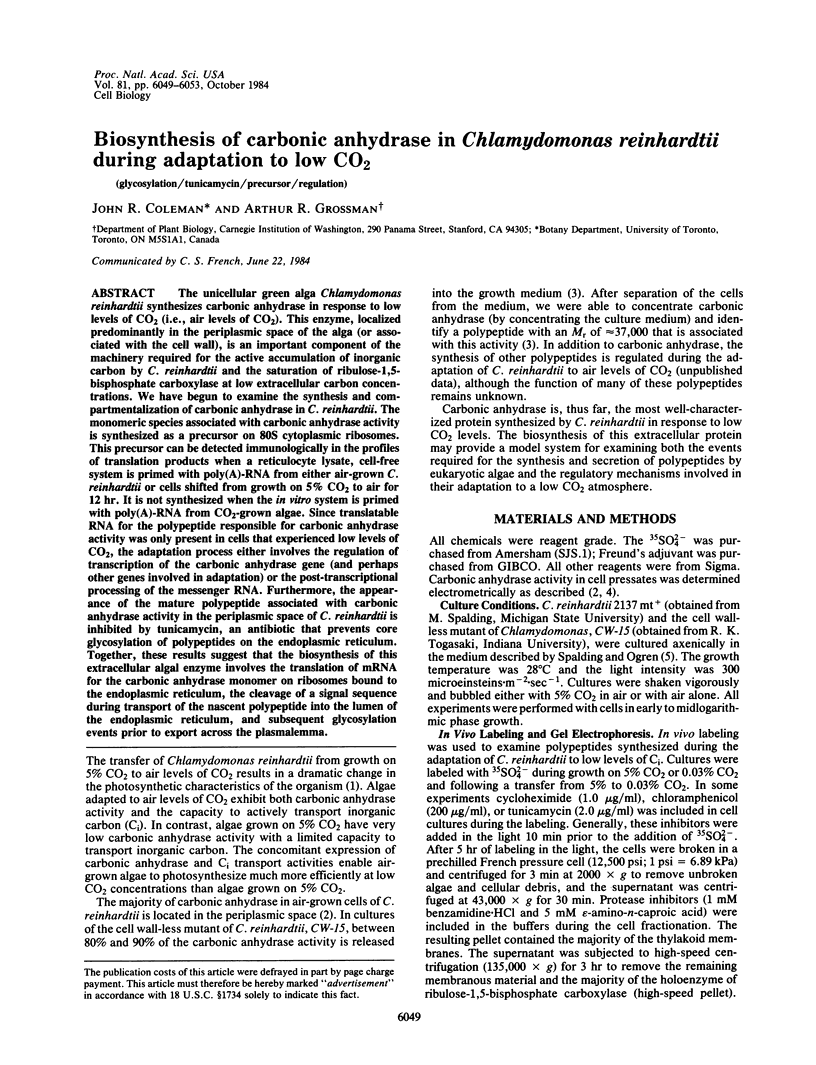
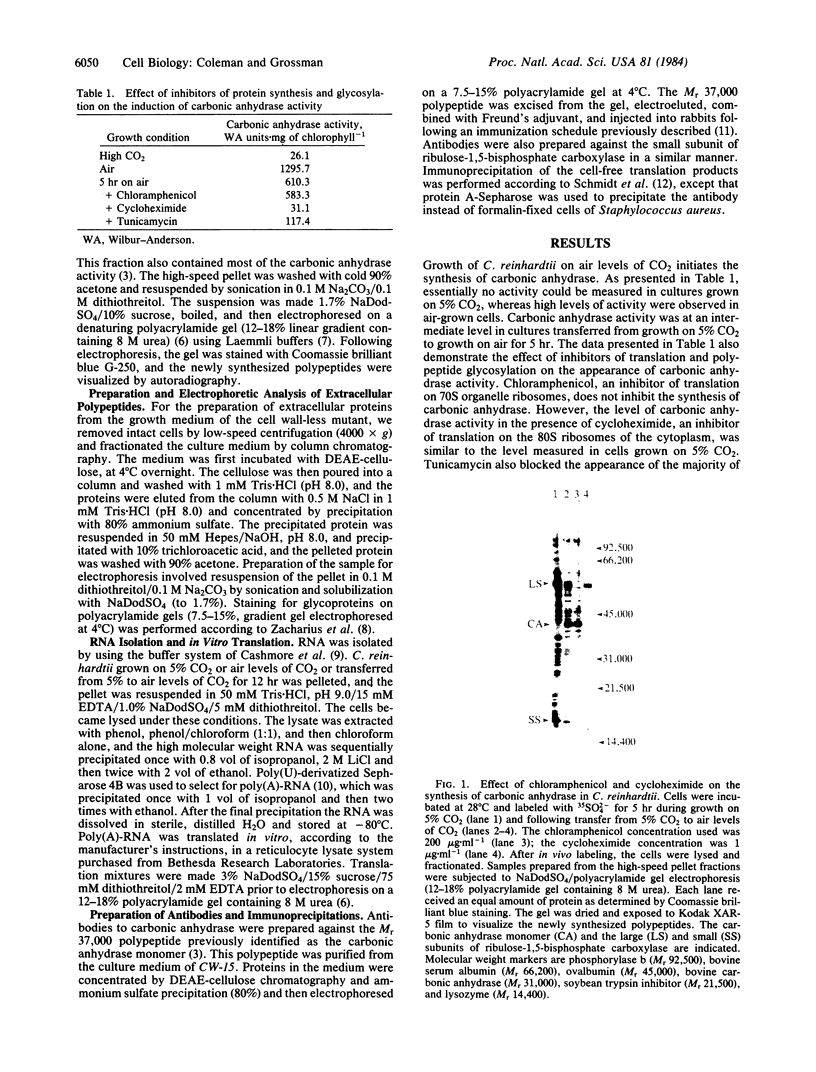
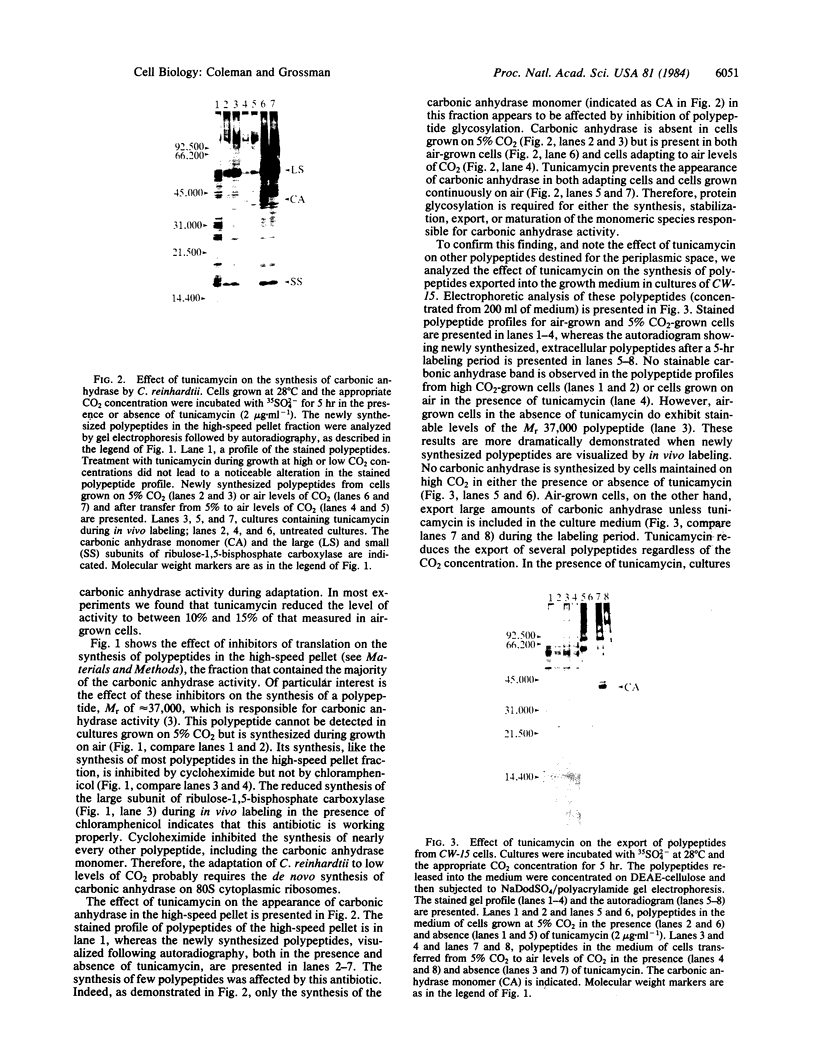
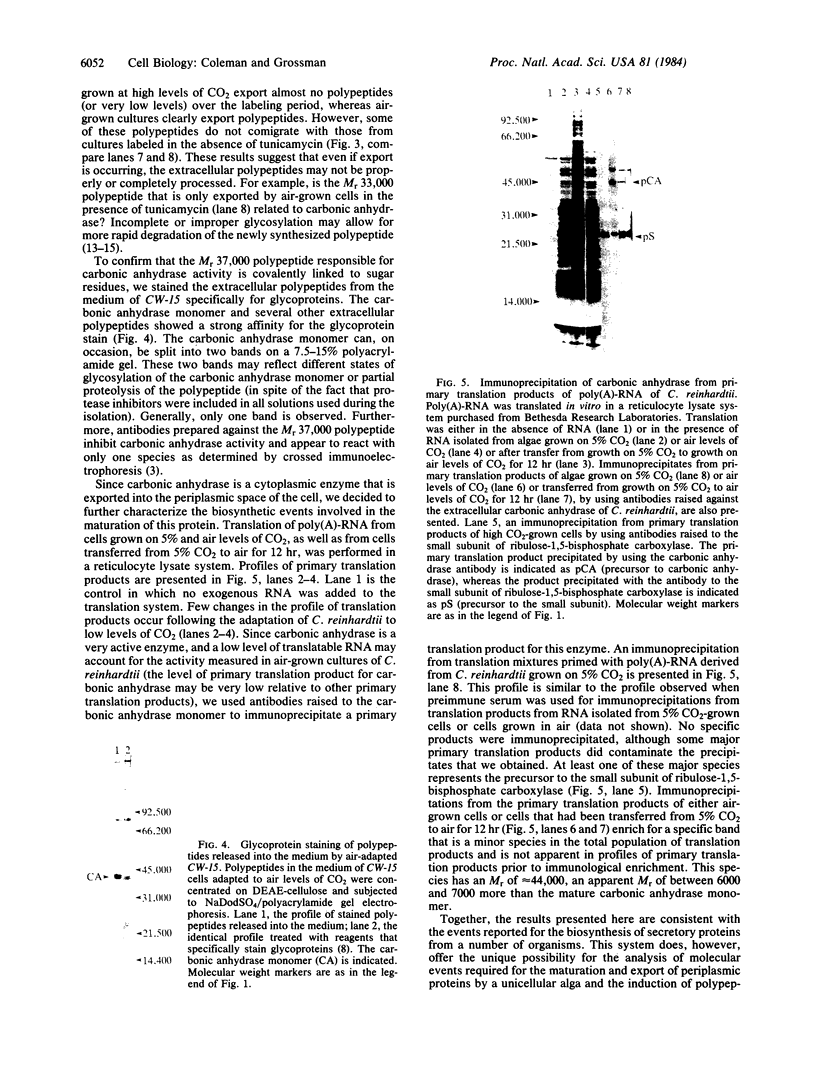
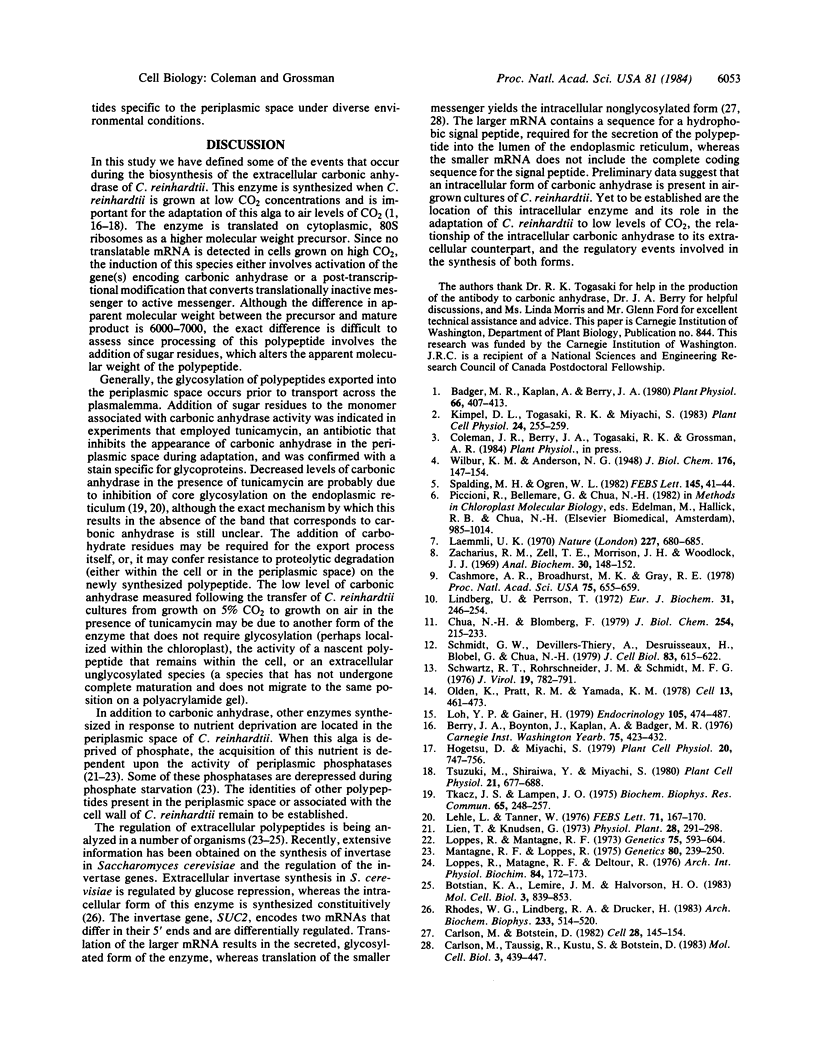
Images in this article
Selected References
These references are in PubMed. This may not be the complete list of references from this article.
- Badger M. R., Kaplan A., Berry J. A. Internal Inorganic Carbon Pool of Chlamydomonas reinhardtii: EVIDENCE FOR A CARBON DIOXIDE-CONCENTRATING MECHANISM. Plant Physiol. 1980 Sep;66(3):407–413. doi: 10.1104/pp.66.3.407. [DOI] [PMC free article] [PubMed] [Google Scholar]
- Bostian K. A., Lemire J. M., Halvorson H. O. Physiological control of repressible acid phosphatase gene transcripts in Saccharomyces cerevisiae. Mol Cell Biol. 1983 May;3(5):839–853. doi: 10.1128/mcb.3.5.839. [DOI] [PMC free article] [PubMed] [Google Scholar]
- Carlson M., Botstein D. Two differentially regulated mRNAs with different 5' ends encode secreted with intracellular forms of yeast invertase. Cell. 1982 Jan;28(1):145–154. doi: 10.1016/0092-8674(82)90384-1. [DOI] [PubMed] [Google Scholar]
- Carlson M., Taussig R., Kustu S., Botstein D. The secreted form of invertase in Saccharomyces cerevisiae is synthesized from mRNA encoding a signal sequence. Mol Cell Biol. 1983 Mar;3(3):439–447. doi: 10.1128/mcb.3.3.439. [DOI] [PMC free article] [PubMed] [Google Scholar]
- Cashmore A. R., Broadhurst M. K., Gray R. E. Cell-free synthesis of leaf protein: Identification of an apparent precursor of the small subunit of ribulose-1,5-bisphosphate carboxylase. Proc Natl Acad Sci U S A. 1978 Feb;75(2):655–659. doi: 10.1073/pnas.75.2.655. [DOI] [PMC free article] [PubMed] [Google Scholar]
- Chua N. H., Blomberg F. Immunochemical studies of thylakoid membrane polypeptides from spinach and Chlamydomonas reinhardtii. A modified procedure for crossed immunoelectrophoresis of dodecyl sulfate.protein complexes. J Biol Chem. 1979 Jan 10;254(1):215–223. [PubMed] [Google Scholar]
- Laemmli U. K. Cleavage of structural proteins during the assembly of the head of bacteriophage T4. Nature. 1970 Aug 15;227(5259):680–685. doi: 10.1038/227680a0. [DOI] [PubMed] [Google Scholar]
- Lehle L., Tanner W. The specific site of tunicamycin inhibition in the formation of dolichol-bound N-acetylglucosamine derivatives. FEBS Lett. 1976 Nov 15;72(1):167–170. doi: 10.1016/0014-5793(76)80922-2. [DOI] [PubMed] [Google Scholar]
- Lindberg U., Persson T. Isolation of mRNA from KB-cells by affinity chromatography on polyuridylic acid covalently linked to Sepharose. Eur J Biochem. 1972 Dec 4;31(2):246–254. doi: 10.1111/j.1432-1033.1972.tb02527.x. [DOI] [PubMed] [Google Scholar]
- Loh Y. P., Gainer H. The role of the carbohydrate in the stabilization, processing, and packaging of the glycosylated adrenocorticotropin-endorphin common precursor in toad pituitaries. Endocrinology. 1979 Aug;105(2):474–487. doi: 10.1210/endo-105-2-474. [DOI] [PubMed] [Google Scholar]
- Loppes R., Matagne R. F. Acid phosphatase mutants in Chlamydomonas: isolation and characterization by biochemical, electrophoretic and genetic analysis. Genetics. 1973 Dec;75(4):593–604. doi: 10.1093/genetics/75.4.593. [DOI] [PMC free article] [PubMed] [Google Scholar]
- Loppes R., Matagne R. F., Deltour R. Proceedings: Biochemical and cytochemical study of the two constitutive acid phosphatases in Chlamydomonas reinhardi. Arch Int Physiol Biochim. 1976 Feb;84(1):172–173. [PubMed] [Google Scholar]
- Matagne R. F., Loppes R. Isolation and study of mutants lacking a derepressible phosphatase in Chlamydomonas reinhardi. Genetics. 1975 Jun;80(2):239–250. doi: 10.1093/genetics/80.2.239. [DOI] [PMC free article] [PubMed] [Google Scholar]
- Olden K., Pratt R. M., Yamada K. M. Role of carbohydrates in protein secretion and turnover: effects of tunicamycin on the major cell surface glycoprotein of chick embryo fibroblasts. Cell. 1978 Mar;13(3):461–473. doi: 10.1016/0092-8674(78)90320-3. [DOI] [PubMed] [Google Scholar]
- Rhodes W. G., Lindberg R. A., Drucker H. Purification and characterization of an extracellular acid protease from Neurospora crassa. Arch Biochem Biophys. 1983 Jun;223(2):514–520. doi: 10.1016/0003-9861(83)90616-1. [DOI] [PubMed] [Google Scholar]
- Schmidt G. W., Devillers-Thiery A., Desruisseaux H., Blobel G., Chua N. H. NH2-terminal amino acid sequences of precursor and mature forms of the ribulose-1,5-bisphosphate carboxylase small subunit from Chlamydomonas reinhardtii. J Cell Biol. 1979 Dec;83(3):615–622. doi: 10.1083/jcb.83.3.615. [DOI] [PMC free article] [PubMed] [Google Scholar]
- Schwarz R. T., Rohrschneider J. M., Schmidt M. F. Suppression of glycoprotein formation of Semliki Forest, influenza, and avian sarcoma virus by tunicamycin. J Virol. 1976 Sep;19(3):782–791. doi: 10.1128/jvi.19.3.782-791.1976. [DOI] [PMC free article] [PubMed] [Google Scholar]
- Tkacz J. S., Lampen O. Tunicamycin inhibition of polyisoprenyl N-acetylglucosaminyl pyrophosphate formation in calf-liver microsomes. Biochem Biophys Res Commun. 1975 Jul 8;65(1):248–257. doi: 10.1016/s0006-291x(75)80086-6. [DOI] [PubMed] [Google Scholar]
- Zacharius R. M., Zell T. E., Morrison J. H., Woodlock J. J. Glycoprotein staining following electrophoresis on acrylamide gels. Anal Biochem. 1969 Jul;30(1):148–152. doi: 10.1016/0003-2697(69)90383-2. [DOI] [PubMed] [Google Scholar]