Abstract
Anaerobic acetogenic bacteria utilize a pathway of autotrophic growth that differs from any previously described. One part of the pathway involves the reduction of CO2 to formate and its subsequent conversion to the methyl moiety of methyltetrahydrofolate. The second part involves the formation of a one-carbon intermediate from CO, CO2 and H2, or the carboxyl of pyruvate and combination of the intermediate with CoA and methyltetrahydrofolate mediated by a corrinoid enzyme to yield acetyl-CoA. Our studies have been concerned with this latter portion of the pathway and we have proposed that a one-carbon intermediate is formed via carbon monoxide dehydrogenase. It remained possible, however, that the function of the CO dehydrogenase is to reduce the cobalt of the corrinoid enzyme to Co+, which is required for it to act as a methyl acceptor, and that the dehydrogenase is not involved directly in the formation of a C1 intermediate. All the enzymes required for the synthesis of acetyl-CoA from CO and methyltetrahydrofolate or from methyltetrahydrofolate and the carboxyl of pyruvate have now been purified. With these purified enzymes, it has been possible to show that CO dehydrogenase is essential for acetyl-CoA synthesis with CO as the substrate under conditions in which the cobalt of the corrinoid is reduced by other means. In addition, using pyruvate ferredoxin oxidoreductase, it has been shown that a 14C1-CO dehydrogenase complex is formed from [1-14C]pyruvate. Furthermore, [1-14C]acetyl-CoA was synthesized using the 14C1-CO dehydrogenase complex. Thus the evidence appears conclusive that CO dehydrogenase has a direct role in the formation of the carboxyl of acetyl-CoA.
Full text
PDF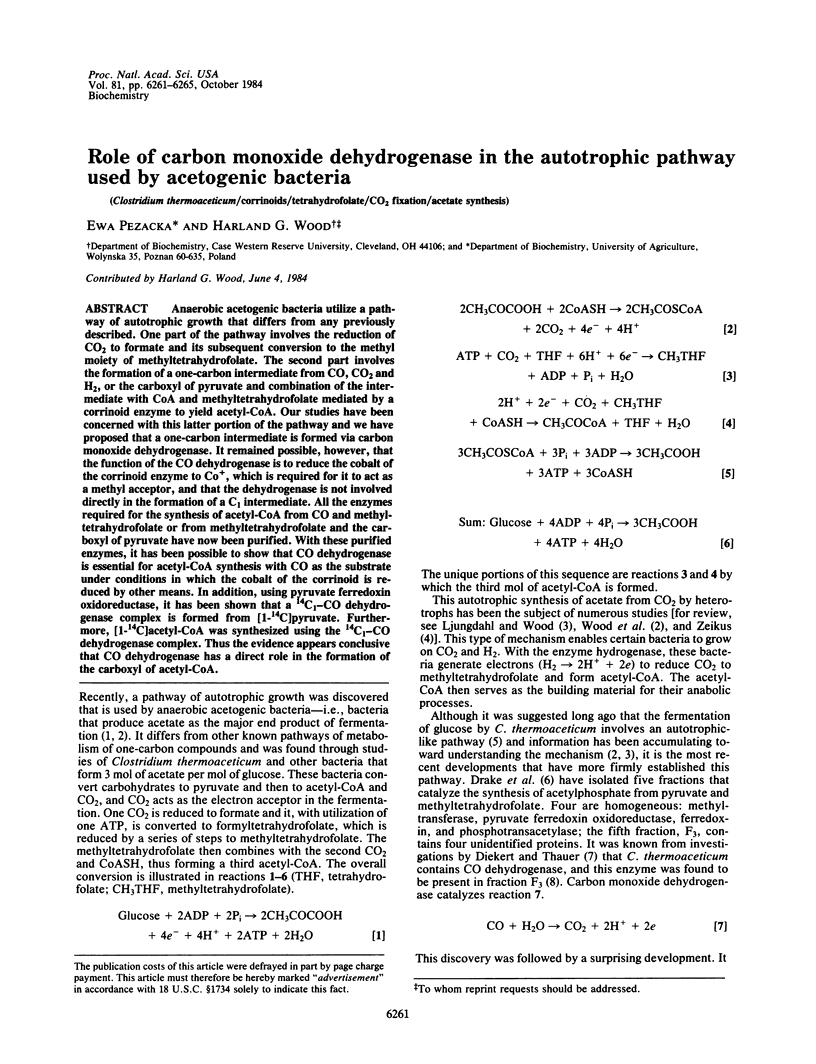
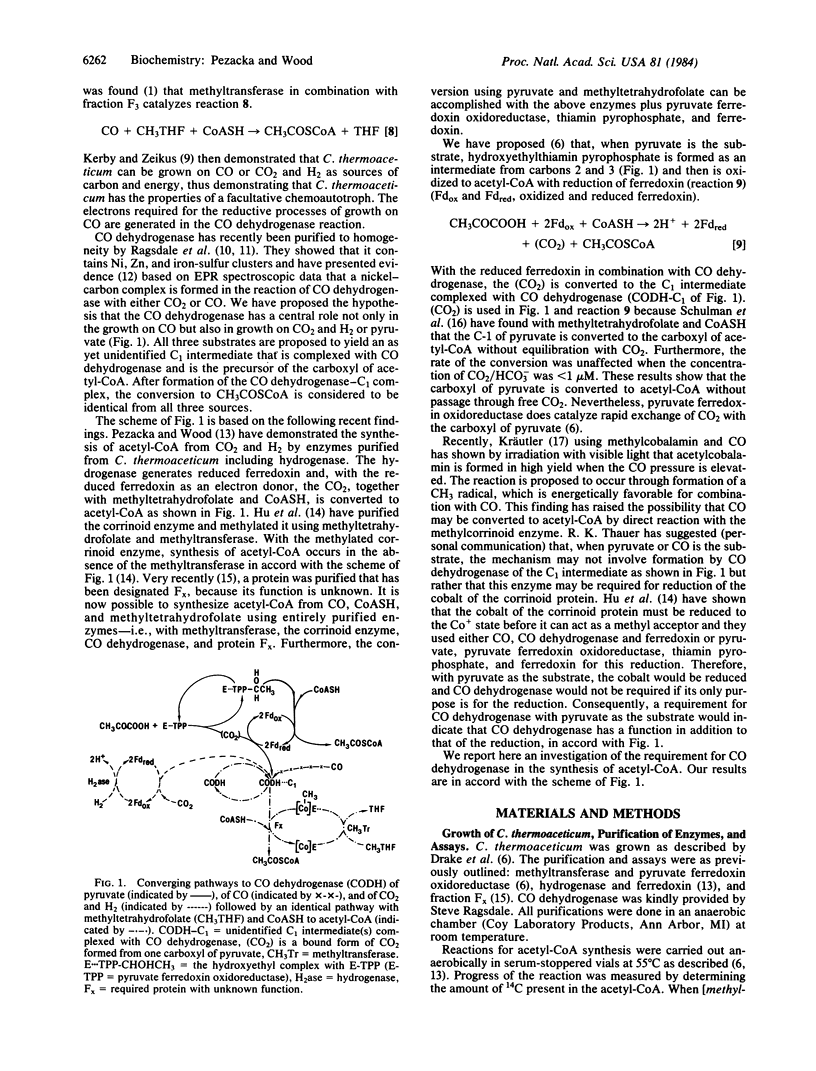
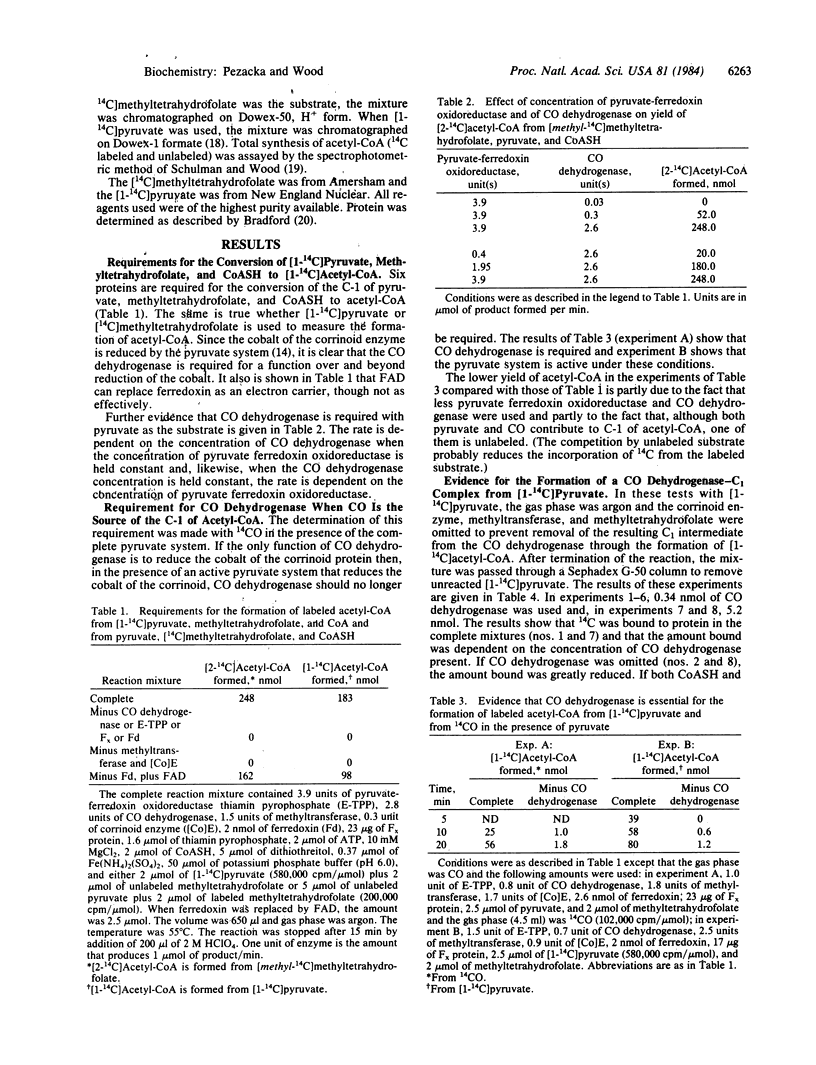
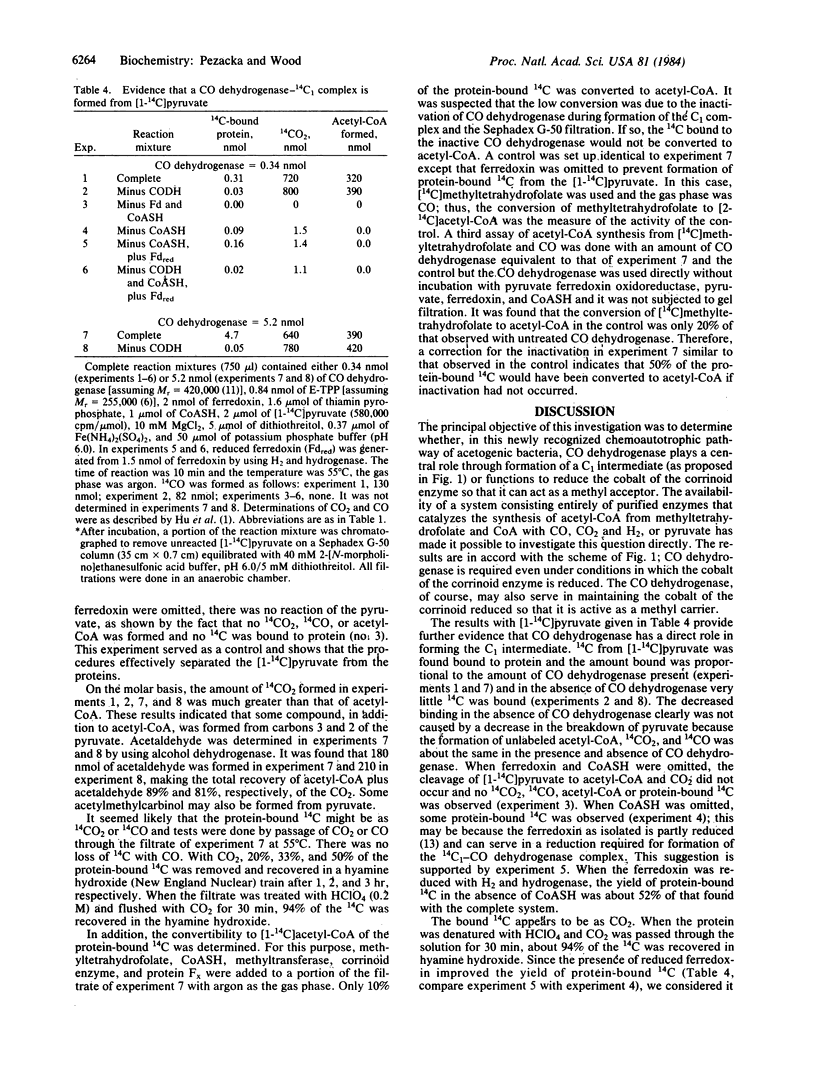
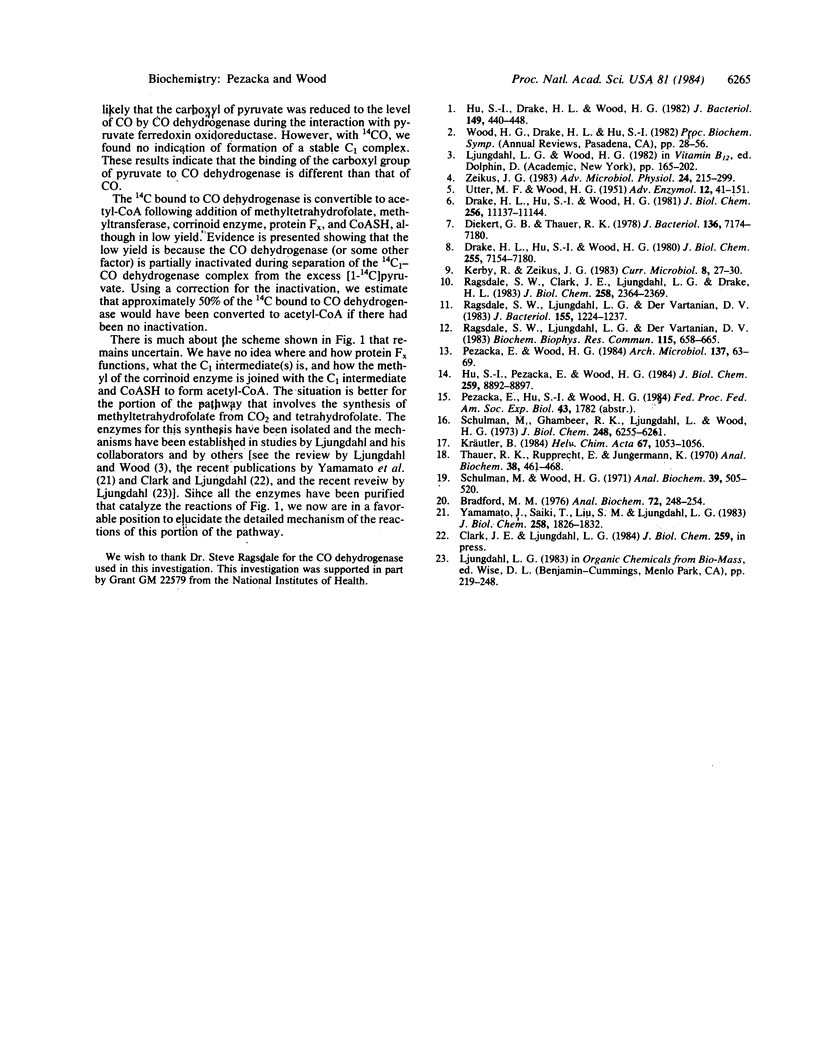
Selected References
These references are in PubMed. This may not be the complete list of references from this article.
- Bradford M. M. A rapid and sensitive method for the quantitation of microgram quantities of protein utilizing the principle of protein-dye binding. Anal Biochem. 1976 May 7;72:248–254. doi: 10.1016/0003-2697(76)90527-3. [DOI] [PubMed] [Google Scholar]
- Drake H. L., Hu S. I., Wood H. G. Purification of carbon monoxide dehydrogenase, a nickel enzyme from Clostridium thermocaceticum. J Biol Chem. 1980 Aug 10;255(15):7174–7180. [PubMed] [Google Scholar]
- Drake H. L., Hu S. I., Wood H. G. Purification of five components from Clostridium thermoaceticum which catalyze synthesis of acetate from pyruvate and methyltetrahydrofolate. Properties of phosphotransacetylase. J Biol Chem. 1981 Nov 10;256(21):11137–11144. [PubMed] [Google Scholar]
- Hu S. I., Drake H. L., Wood H. G. Synthesis of acetyl coenzyme A from carbon monoxide, methyltetrahydrofolate, and coenzyme A by enzymes from Clostridium thermoaceticum. J Bacteriol. 1982 Feb;149(2):440–448. doi: 10.1128/jb.149.2.440-448.1982. [DOI] [PMC free article] [PubMed] [Google Scholar]
- Hu S. I., Pezacka E., Wood H. G. Acetate synthesis from carbon monoxide by Clostridium thermoaceticum. Purification of the corrinoid protein. J Biol Chem. 1984 Jul 25;259(14):8892–8897. [PubMed] [Google Scholar]
- Pezacka E., Wood H. G. The synthesis of acetyl-CoA by Clostridium thermoaceticum from carbon dioxide, hydrogen, coenzyme A and methyltetrahydrofolate. Arch Microbiol. 1984 Jan;137(1):63–69. doi: 10.1007/BF00425809. [DOI] [PubMed] [Google Scholar]
- Ragsdale S. W., Clark J. E., Ljungdahl L. G., Lundie L. L., Drake H. L. Properties of purified carbon monoxide dehydrogenase from Clostridium thermoaceticum, a nickel, iron-sulfur protein. J Biol Chem. 1983 Feb 25;258(4):2364–2369. [PubMed] [Google Scholar]
- Ragsdale S. W., Ljungdahl L. G., DerVartanian D. V. 13C and 61Ni isotope substitutions confirm the presence of a nickel (III)-carbon species in acetogenic CO dehydrogenases. Biochem Biophys Res Commun. 1983 Sep 15;115(2):658–665. doi: 10.1016/s0006-291x(83)80195-8. [DOI] [PubMed] [Google Scholar]
- Ragsdale S. W., Ljungdahl L. G., DerVartanian D. V. Isolation of carbon monoxide dehydrogenase from Acetobacterium woodii and comparison of its properties with those of the Clostridium thermoaceticum enzyme. J Bacteriol. 1983 Sep;155(3):1224–1237. doi: 10.1128/jb.155.3.1224-1237.1983. [DOI] [PMC free article] [PubMed] [Google Scholar]
- Schulman M., Ghambeer R. K., Ljungdahl L. G., Wood H. G. Total synthesis of acetate from CO2. VII. Evidence with Clostridium thermoaceticum that the carboxyl of acetate is derived from the carboxyl of pyruvate by transcarboxylation and not by fixation of CO2. J Biol Chem. 1973 Sep 25;248(18):6255–6261. [PubMed] [Google Scholar]
- Schulman M., Wood H. G. Determination and degradation of microquantities of acetate. Anal Biochem. 1971 Feb;39(2):505–520. doi: 10.1016/0003-2697(71)90441-6. [DOI] [PubMed] [Google Scholar]
- Thauer R. K., Rupprecht E., Jungermann K. Separation of 14C-formate from CO2 fixation metabolites by isoionic-exchange chromatography. Anal Biochem. 1970 Dec;38(2):461–468. doi: 10.1016/0003-2697(70)90471-9. [DOI] [PubMed] [Google Scholar]
- UTTER M. F., WOOD H. G. Mechanisms of fixation of carbon dioxide by heterotrophes and autotrophs. Adv Enzymol Relat Subj Biochem. 1951;12:41–151. doi: 10.1002/9780470122570.ch2. [DOI] [PubMed] [Google Scholar]
- Yamamoto I., Saiki T., Liu S. M., Ljungdahl L. G. Purification and properties of NADP-dependent formate dehydrogenase from Clostridium thermoaceticum, a tungsten-selenium-iron protein. J Biol Chem. 1983 Feb 10;258(3):1826–1832. [PubMed] [Google Scholar]
- Zeikus J. G. Metabolism of one-carbon compounds by chemotrophic anaerobes. Adv Microb Physiol. 1983;24:215–299. doi: 10.1016/s0065-2911(08)60387-2. [DOI] [PubMed] [Google Scholar]