Abstract
In protoplasts isolated from nonacclimated rye leaves (Secale cereale L. cultivar Puma), cooling to -- 10 degrees C at a rate of 1 degrees C/min results in extensive freeze-induced dehydration (osmotic contraction), and injury is manifested as the loss of osmotic responsiveness during warming. Under these conditions, several changes were observed in the freeze-fracture morphology of the plasma membrane. These included (i) lateral phase separations in the plasma membrane, (ii) aparticulate lamellae lying next to the plasma membrane, and (iii) regions of the plasma membrane and associated lamellae in various stages of lamellar-to-hexagonalII transition. These morphological changes also were observed after equilibration in 5.37 osmolal sorbitol at 0 degrees C, which produced a similar extent of dehydration as did freezing to -- 10 degrees C. In contrast, only small areas of lateral phase separation in the plasma membrane, with no observable aparticulate lamellae or hexagonalII configurations, were observed in protoplasts supercooled to -- 10 degrees C. Therefore, freeze-induced lamellar-to-hexagonalII phase transitions in the plasma membrane are a consequence of dehydration rather than subzero temperature per se. When suspensions of protoplasts isolated from cold-acclimated leaves were frozen to -- 10 degrees C, no injury was incurred, and hexagonalII phase transitions were not observed. No hexagonalII phase was observed even at -- 35 degrees C, though acclimated protoplasts are injured at this temperature.
Full text
PDF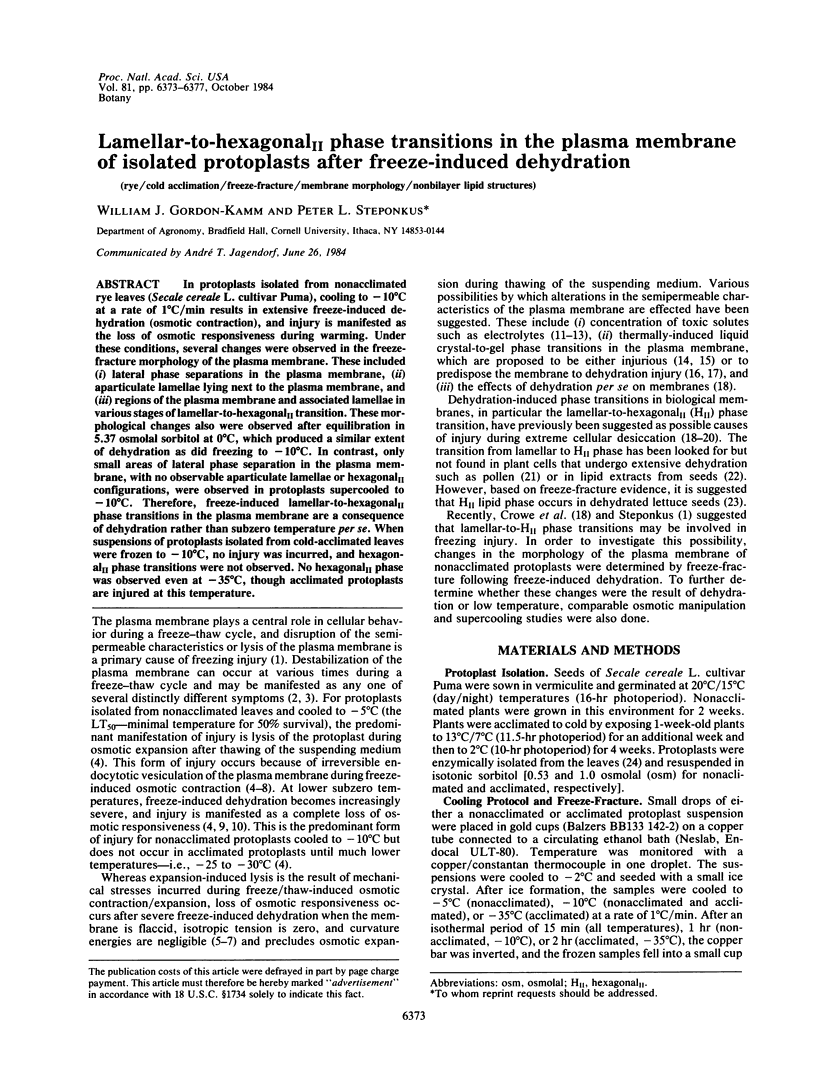
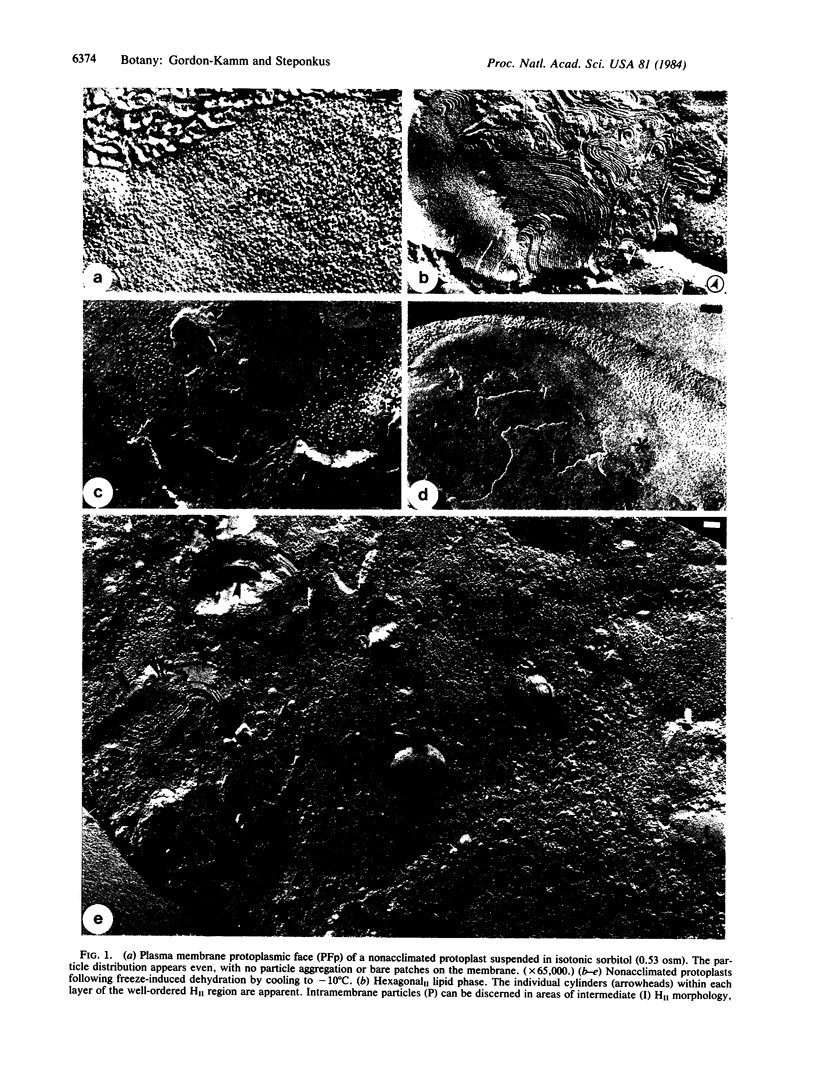
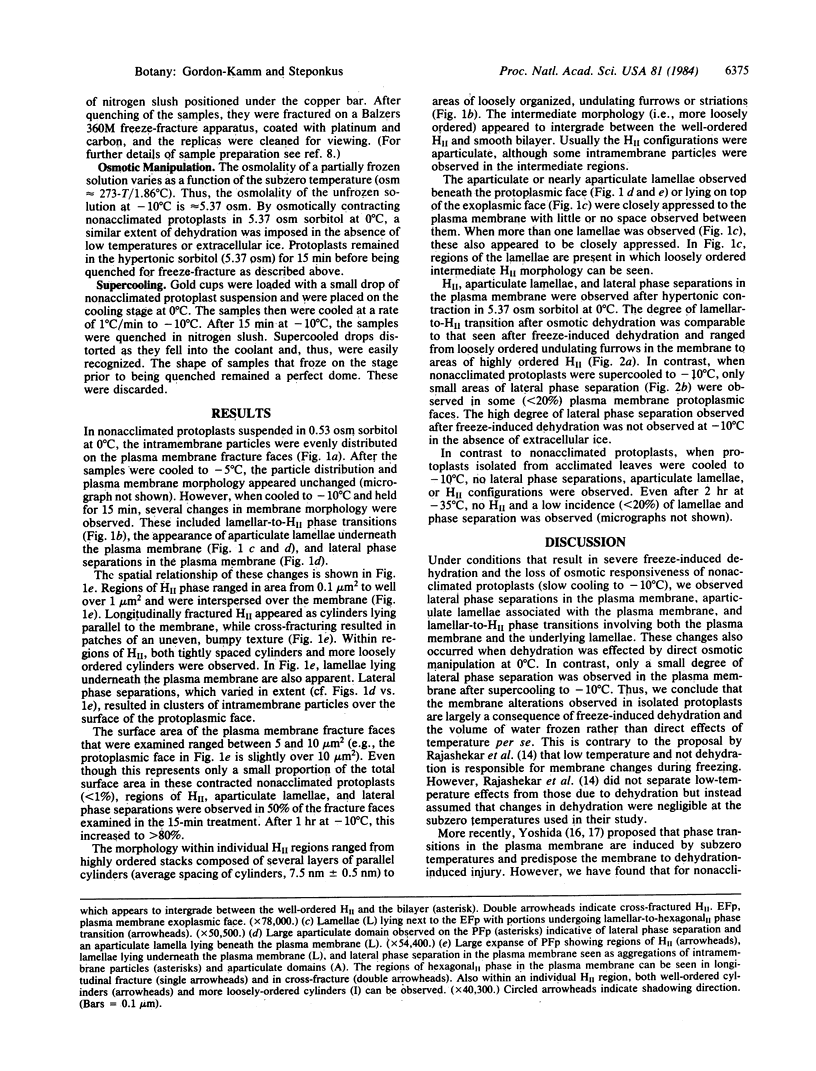
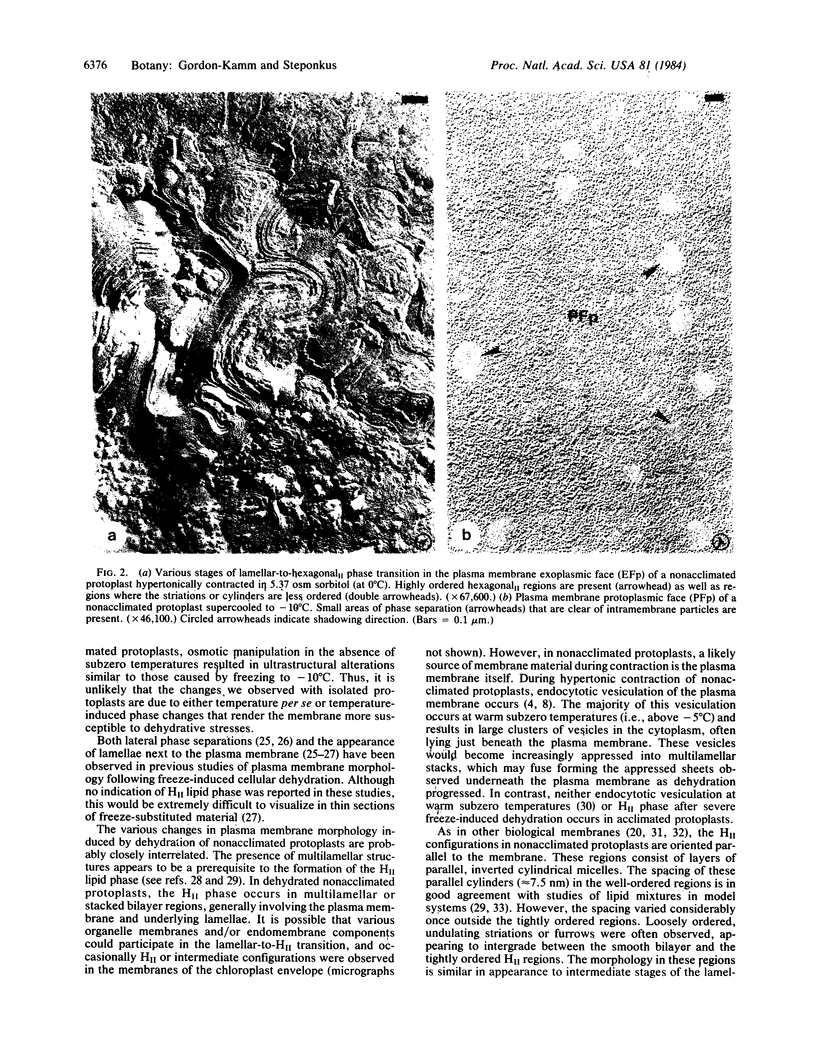
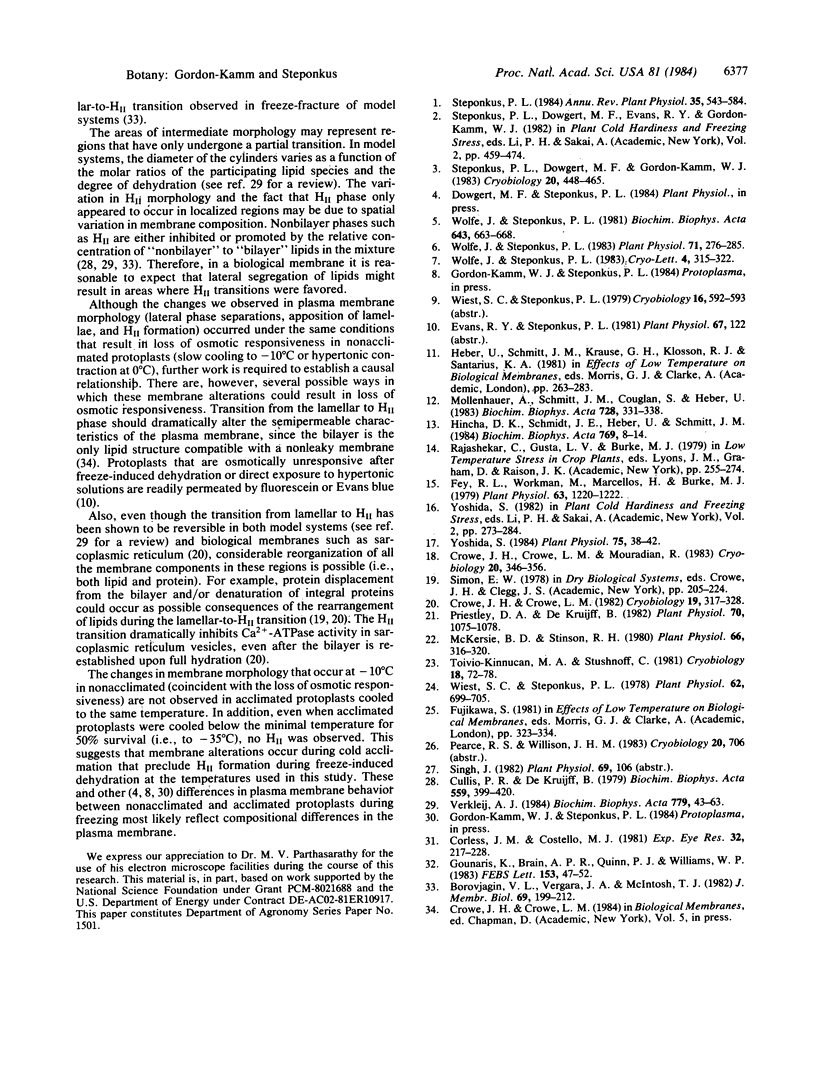
Images in this article
Selected References
These references are in PubMed. This may not be the complete list of references from this article.
- Borovjagin V. L., Vergara J. A., McIntosh T. J. Morphology of the intermediate stages in the lamellar to hexagonal lipid phase transition. J Membr Biol. 1982;69(3):199–212. doi: 10.1007/BF01870399. [DOI] [PubMed] [Google Scholar]
- Corless J. M., Costello M. J. Paracrystalline inclusions associated with the disk membranes of frog retinal rod outer segments. Exp Eye Res. 1981 Feb;32(2):217–228. doi: 10.1016/0014-4835(81)90010-5. [DOI] [PubMed] [Google Scholar]
- Crowe J. H., Crowe L. M. Induction of anhydrobiosis: membrane changes during drying. Cryobiology. 1982 Jun;19(3):317–328. doi: 10.1016/0011-2240(82)90160-2. [DOI] [PubMed] [Google Scholar]
- Crowe J. H., Crowe L. M., Mouradian R. Stabilization of biological membranes at low water activities. Cryobiology. 1983 Jun;20(3):346–356. doi: 10.1016/0011-2240(83)90023-8. [DOI] [PubMed] [Google Scholar]
- Cullis P. R., de Kruijff B. Lipid polymorphism and the functional roles of lipids in biological membranes. Biochim Biophys Acta. 1979 Dec 20;559(4):399–420. doi: 10.1016/0304-4157(79)90012-1. [DOI] [PubMed] [Google Scholar]
- Fey R. L., Workman M., Marcellos H., Burke M. J. Electron Spin Resonance of 2,2,6,6-Tetramethylpiperidine-1-Oxyl (TEMPO)-labeled Plant Leaves. Plant Physiol. 1979 Jun;63(6):1220–1222. doi: 10.1104/pp.63.6.1220. [DOI] [PMC free article] [PubMed] [Google Scholar]
- McKersie B. D., Stinson R. H. Effect of Dehydration on Leakage and Membrane Structure in Lotus corniculatus L. Seeds. Plant Physiol. 1980 Aug;66(2):316–320. doi: 10.1104/pp.66.2.316. [DOI] [PMC free article] [PubMed] [Google Scholar]
- Priestley D. A., de Kruijff B. Phospholipid Motional Characteristics in a Dry Biological System : A P-Nuclear Magnetic Resonance Study of Hydrating Typha latifolia Pollen. Plant Physiol. 1982 Oct;70(4):1075–1078. doi: 10.1104/pp.70.4.1075. [DOI] [PMC free article] [PubMed] [Google Scholar]
- Steponkus P. L., Dowgert M. F., Gordon-Kamm W. J. Destabilization of the plasma membrane of isolated plant protoplasts during a freeze-thaw cycle: the influence of cold acclimation. Cryobiology. 1983 Aug;20(4):448–465. doi: 10.1016/0011-2240(83)90035-4. [DOI] [PubMed] [Google Scholar]
- Toivio-Kinnucan M. A., Stushnoff C. Lipid participation in intracellular freezing avoidance mechanisms of lettuce seed. Cryobiology. 1981 Feb;18(1):72–78. doi: 10.1016/0011-2240(81)90007-9. [DOI] [PubMed] [Google Scholar]
- Verkleij A. J. Lipidic intramembranous particles. Biochim Biophys Acta. 1984 Jan 27;779(1):43–63. doi: 10.1016/0304-4157(84)90003-0. [DOI] [PubMed] [Google Scholar]
- Wiest S. C., Steponkus P. L. Freeze-thaw injury to isolated spinach protoplasts and its simulation at above freezing temperatures. Plant Physiol. 1978 Nov;62(5):699–705. doi: 10.1104/pp.62.5.699. [DOI] [PMC free article] [PubMed] [Google Scholar]
- Wolfe J., Steponkus P. L. Mechanical properties of the plasma membrane of isolated plant protoplasts : mechanism of hyperosmotic and extracellular freezing injury. Plant Physiol. 1983 Feb;71(2):276–285. doi: 10.1104/pp.71.2.276. [DOI] [PMC free article] [PubMed] [Google Scholar]
- Wolfe J., Steponkus P. L. The stress-strain relation of the plasma membrane of isolated plant protoplasts. Biochim Biophys Acta. 1981 May 20;643(3):663–668. doi: 10.1016/0005-2736(81)90363-1. [DOI] [PubMed] [Google Scholar]
- Yoshida S. Studies on Freezing Injury of Plant Cells: I. Relation between Thermotropic Properties of Isolated Plasma Membrane Vesicles and Freezing Injury. Plant Physiol. 1984 May;75(1):38–42. doi: 10.1104/pp.75.1.38. [DOI] [PMC free article] [PubMed] [Google Scholar]