Abstract
Closed circular DNA molecules in aqueous solution take the form of interwound superhelices over a wide range of superhelix densities. We describe a very simple model of such a superhelix in which twisting and bending forces are in balance, subject both to topological constraints and to a limitation on the distance of closet approach of the interwound duplexes of the superhelix. The model is consistent with some of the observed physical properties of closed circular DNA, and suggests that there may be severe limits to the range of allowable geometries for the superhelix structure of minimum energy.
Full text
PDF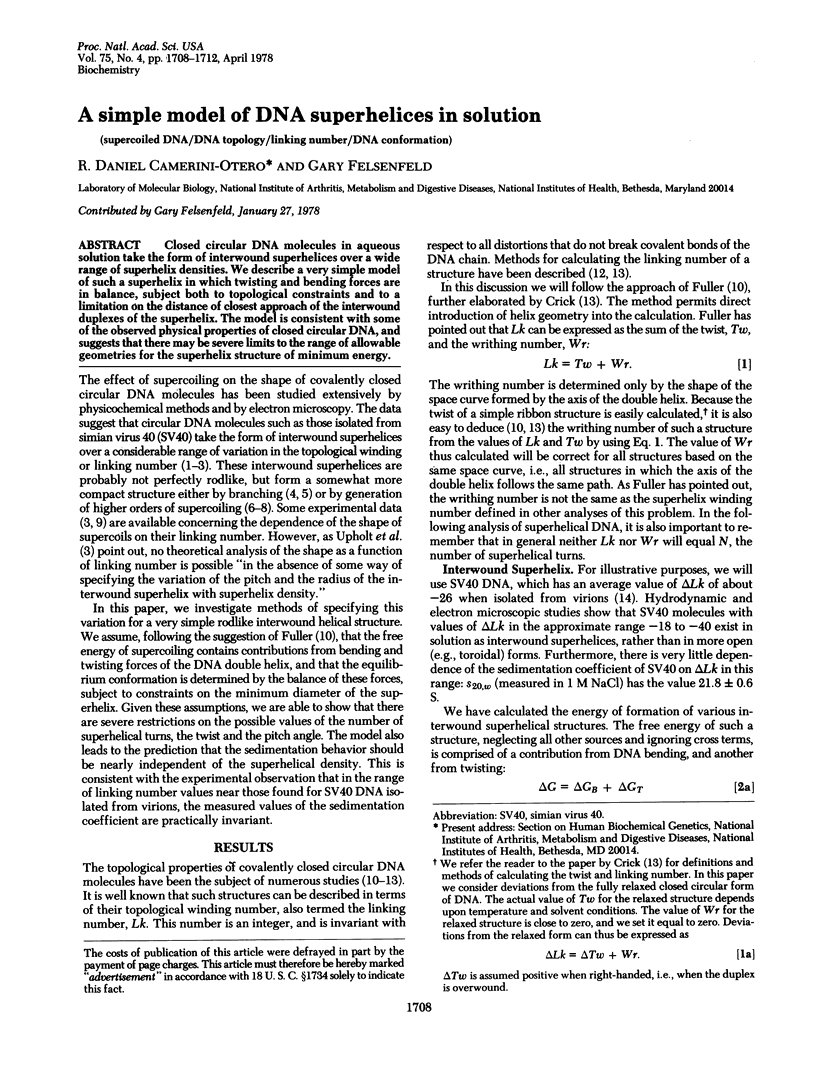
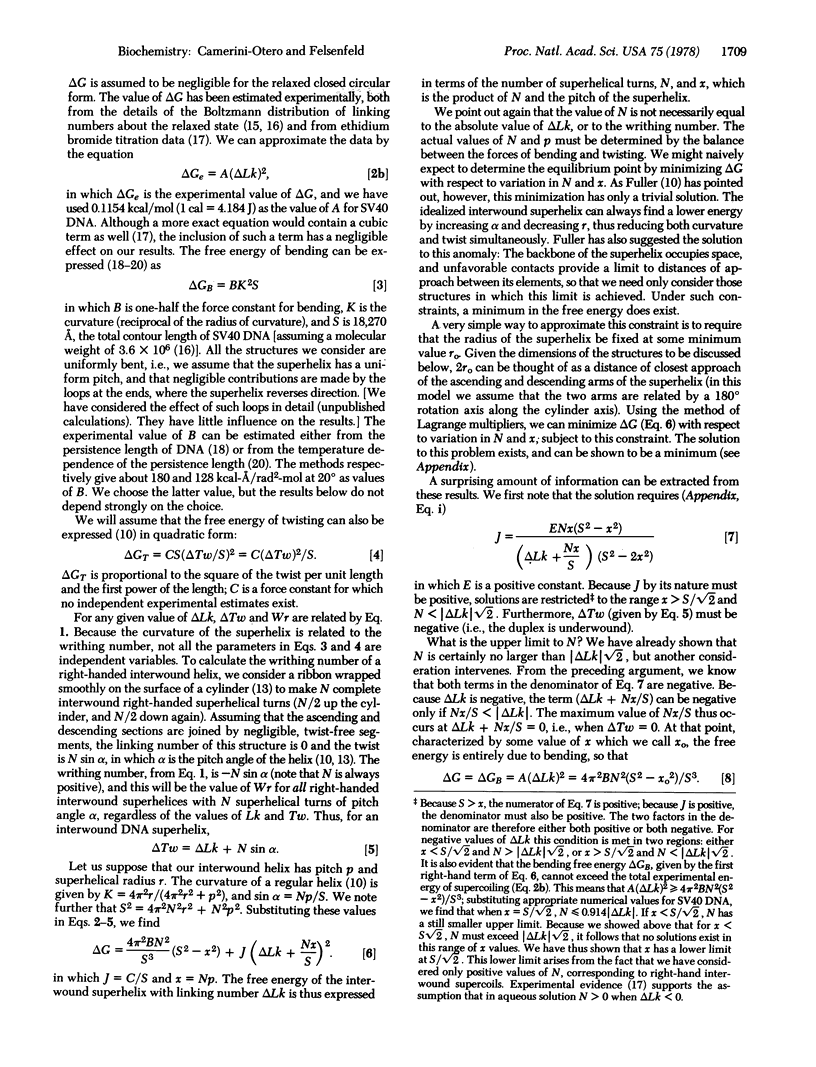
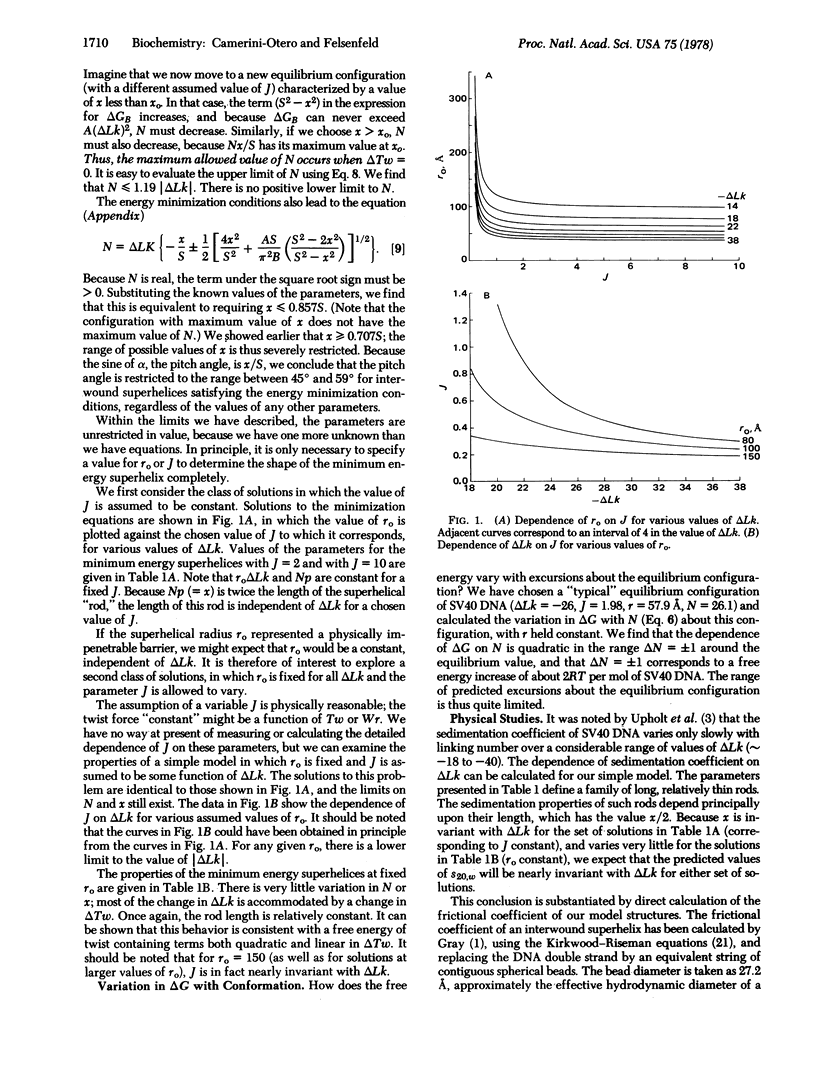
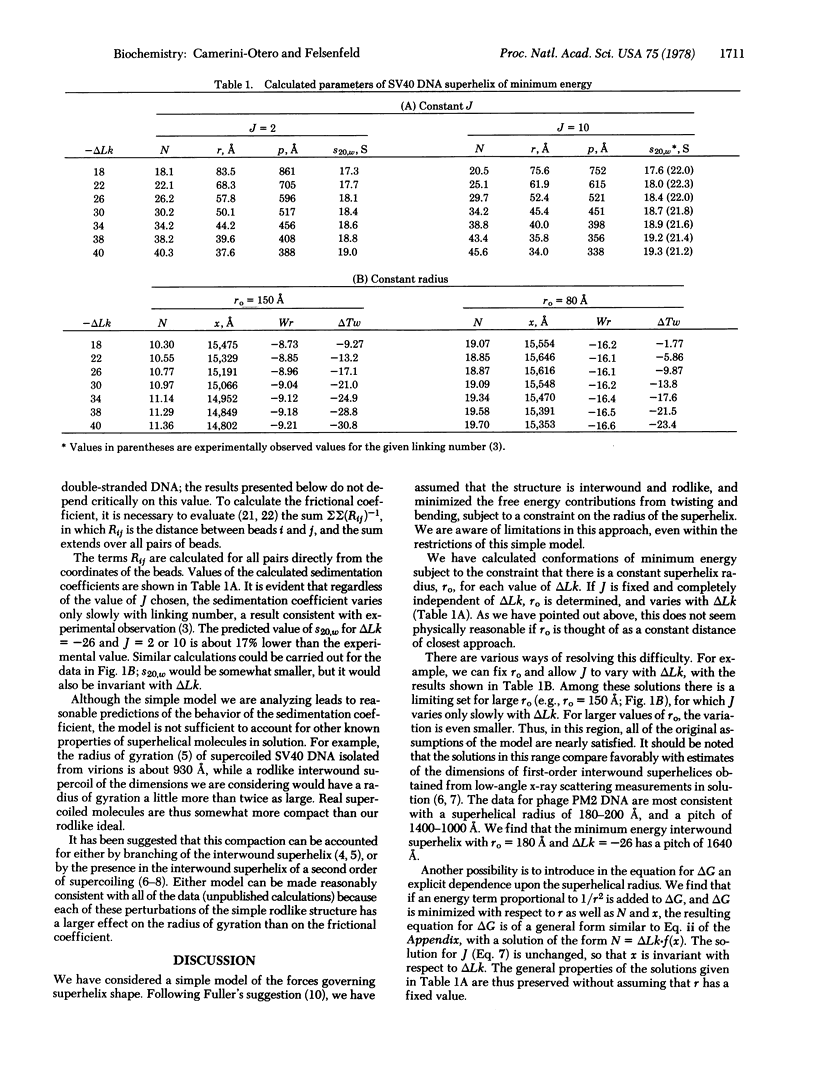
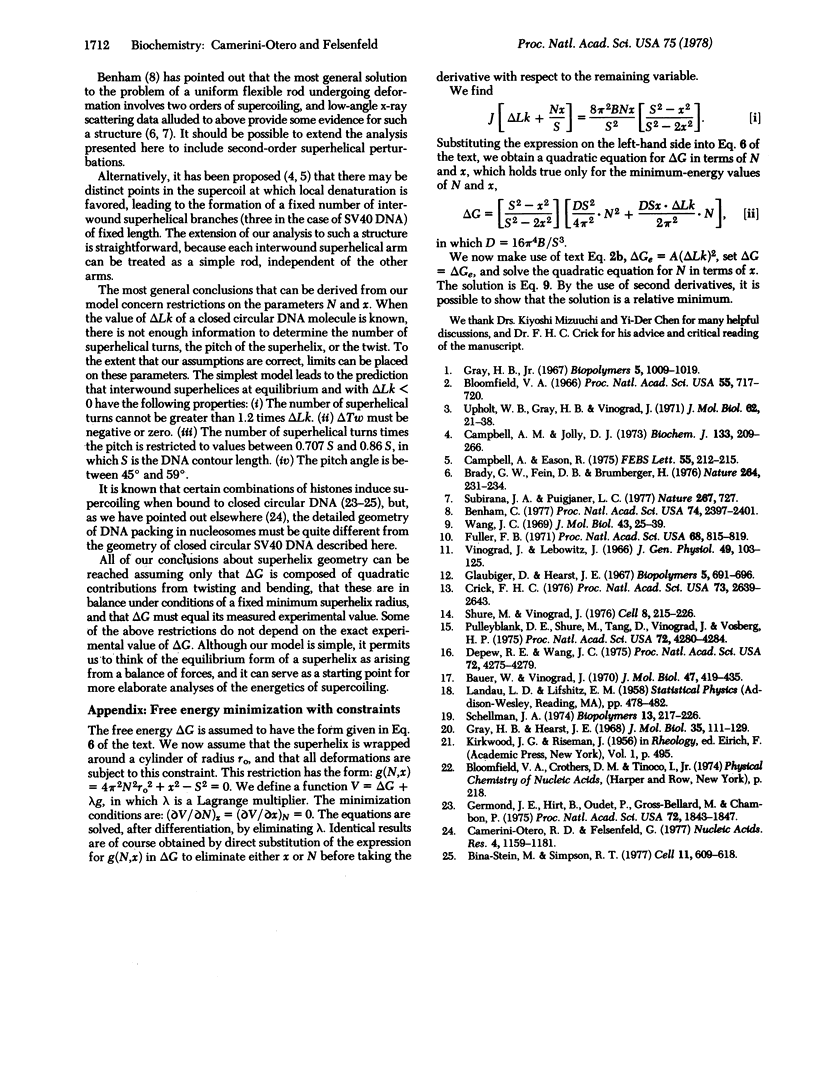
Selected References
These references are in PubMed. This may not be the complete list of references from this article.
- Bauer W., Vinograd J. Interaction of closed circular DNA with intercalative dyes. II. The free energy of superhelix formation in SV40 DNA. J Mol Biol. 1970 Feb 14;47(3):419–435. doi: 10.1016/0022-2836(70)90312-8. [DOI] [PubMed] [Google Scholar]
- Benham C. J. Elastic model of supercoiling. Proc Natl Acad Sci U S A. 1977 Jun;74(6):2397–2401. doi: 10.1073/pnas.74.6.2397. [DOI] [PMC free article] [PubMed] [Google Scholar]
- Bina-Stein M., Simpson R. T. Specific folding and contraction of DNA by histones H3 and H4. Cell. 1977 Jul;11(3):609–618. doi: 10.1016/0092-8674(77)90078-2. [DOI] [PubMed] [Google Scholar]
- Bloomfield V. A. Twisted circular DNA: sedimentation coefficients and the number of twists. Proc Natl Acad Sci U S A. 1966 Apr;55(4):717–720. doi: 10.1073/pnas.55.4.717. [DOI] [PMC free article] [PubMed] [Google Scholar]
- Brady G. W., Fein D. B. X-ray diffraction studies of circular superhelical DNA at 300-10,000-A resolution. Nature. 1976 Nov 18;264(5583):231–234. doi: 10.1038/264231a0. [DOI] [PubMed] [Google Scholar]
- Camerini-Otero R. D., Felsenfeld G. Supercoiling energy and nucleosome formation: the role of the arginine-rich histone kernel. Nucleic Acids Res. 1977;4(5):1159–1181. doi: 10.1093/nar/4.5.1159-a. [DOI] [PMC free article] [PubMed] [Google Scholar]
- Campbell A. M., Jolly D. J. Light-scattering studies on supercoil unwinding. Biochem J. 1973 Jun;133(2):209–226. doi: 10.1042/bj1330209. [DOI] [PMC free article] [PubMed] [Google Scholar]
- Campbell A., Eason R. Effects of DNA primary structure on tertiary structure. FEBS Lett. 1975 Jul 15;55(1):212–215. doi: 10.1016/0014-5793(75)80994-x. [DOI] [PubMed] [Google Scholar]
- Crick F. H. Linking numbers and nucleosomes. Proc Natl Acad Sci U S A. 1976 Aug;73(8):2639–2643. doi: 10.1073/pnas.73.8.2639. [DOI] [PMC free article] [PubMed] [Google Scholar]
- Depew D. E., Wang J. C. Conformational fluctuations of DNA helix. Proc Natl Acad Sci U S A. 1975 Nov;72(11):4275–4279. doi: 10.1073/pnas.72.11.4275. [DOI] [PMC free article] [PubMed] [Google Scholar]
- Fuller F. B. The writhing number of a space curve. Proc Natl Acad Sci U S A. 1971 Apr;68(4):815–819. doi: 10.1073/pnas.68.4.815. [DOI] [PMC free article] [PubMed] [Google Scholar]
- Germond J. E., Hirt B., Oudet P., Gross-Bellark M., Chambon P. Folding of the DNA double helix in chromatin-like structures from simian virus 40. Proc Natl Acad Sci U S A. 1975 May;72(5):1843–1847. doi: 10.1073/pnas.72.5.1843. [DOI] [PMC free article] [PubMed] [Google Scholar]
- Glaubiger D., Hearst J. E. Effect of superhelical structure on the secondary structure of DNA rings. Biopolymers. 1967;5(8):691–696. doi: 10.1002/bip.1967.360050803. [DOI] [PubMed] [Google Scholar]
- Gray H. B., Jr, Hearst J. E. Flexibility of native DNA from the sedimentation behavior as a function of molecular weight and temperature. J Mol Biol. 1968 Jul 14;35(1):111–129. doi: 10.1016/s0022-2836(68)80041-5. [DOI] [PubMed] [Google Scholar]
- Gray H. B., Jr Sedimentation coefficient of polyoma virus DNA. Biopolymers. 1967;5(10):1009–1019. doi: 10.1002/bip.1967.360051012. [DOI] [PubMed] [Google Scholar]
- Pulleyblank D. E., Shure M., Tang D., Vinograd J., Vosberg H. P. Action of nicking-closing enzyme on supercoiled and nonsupercoiled closed circular DNA: formation of a Boltzmann distribution of topological isomers. Proc Natl Acad Sci U S A. 1975 Nov;72(11):4280–4284. doi: 10.1073/pnas.72.11.4280. [DOI] [PMC free article] [PubMed] [Google Scholar]
- Schellman J. A. Flexibility of DNA. Biopolymers. 1974 Jan;13(1):217–226. doi: 10.1002/bip.1974.360130115. [DOI] [PubMed] [Google Scholar]
- Shure M., Vinograd J. The number of superhelical turns in native virion SV40 DNA and minicol DNA determined by the band counting method. Cell. 1976 Jun;8(2):215–226. doi: 10.1016/0092-8674(76)90005-2. [DOI] [PubMed] [Google Scholar]
- Subirana J. A., Puigjaner L. C. Circular superhelical DNA. Nature. 1977 Jun 23;267(5613):727–728. doi: 10.1038/267727a0. [DOI] [PubMed] [Google Scholar]
- Upholt W. B., Gray H. B., Jr, Vinograd J. Sedimentation velocity behavior of closed circular SV40 DNA as a function of superhelix density, ionic strength, counterion and temperature. J Mol Biol. 1971 Nov 28;62(1):21–38. doi: 10.1016/0022-2836(71)90128-8. [DOI] [PubMed] [Google Scholar]
- Vinograd J., Lebowitz J. Physical and topological properties of circular DNA. J Gen Physiol. 1966 Jul;49(6):103–125. doi: 10.1085/jgp.49.6.103. [DOI] [PMC free article] [PubMed] [Google Scholar]
- Wang J. C. Variation of the average rotation angle of the DNA helix and the superhelical turns of covalently closed cyclic lambda DNA. J Mol Biol. 1969 Jul 14;43(1):25–39. doi: 10.1016/0022-2836(69)90076-x. [DOI] [PubMed] [Google Scholar]