Abstract
The density of sodium channels in mammalian myelinated fibers has been estimated from measurements of the binding of [3H]saxitoxin to rabbit sciatic nerve. Binding both to intact and to homogenized nerve consists of a linear, nonspecific, component and a saturable component that represents binding to the sodium channel. The maximum saturable binding capacity in intact nerve is 19.9 +/- 1.9 fmol-mg wet-1; the equilibrium dissociation constant, Kt, is 3.4 +/- 2.0 nM. Homogenization makes little difference, the maximum binding capacity being 19.9 +/- 1.5 fmol-mg wet-1 with Kt = 1.3 +/- 0.7 nM. These values correspond to a density of about 700,000 sodium channels per node--i.e., about 12,000 per mum2 of nodal membrane. From the difference between the values of maximum saturable binding capacity in intact and homogenized preparation, given the statistical uncertainty of their estimate, it seems that the internodal membrane can have no more than about 25 channels per mum2. The significance of these findings for saltatory conduction and in demyelinating disease is discussed.
Full text
PDF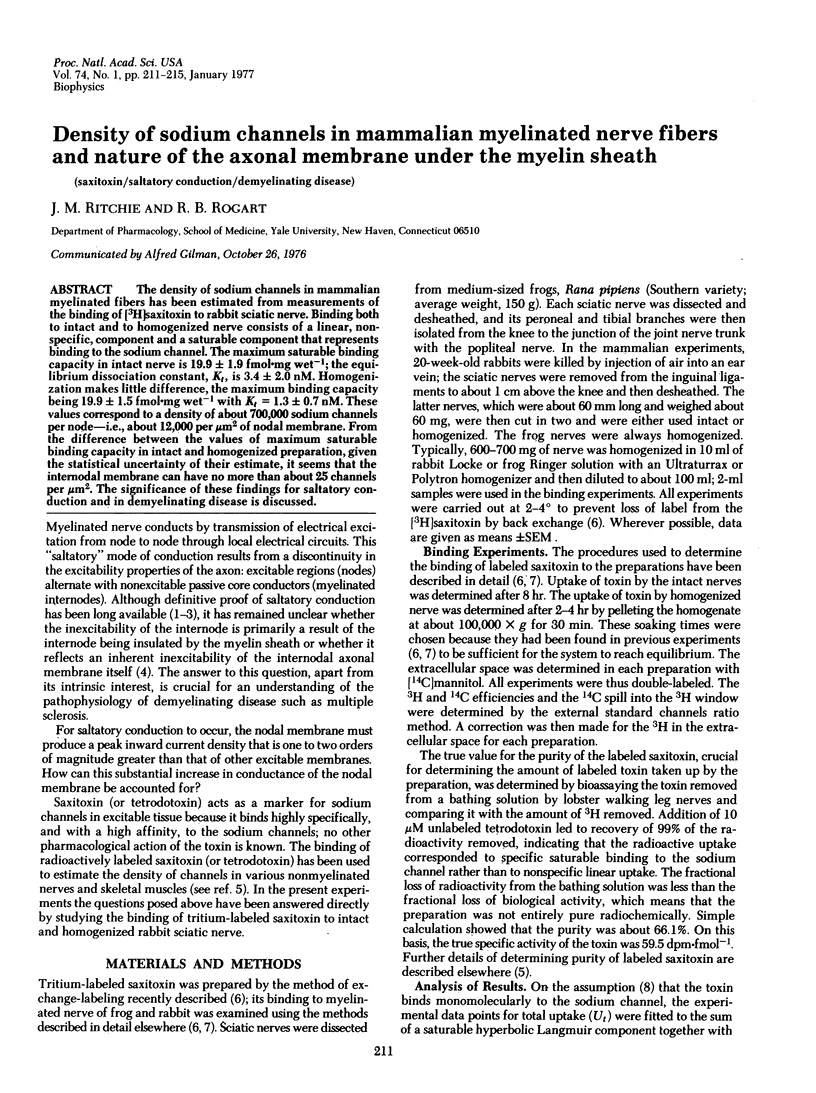
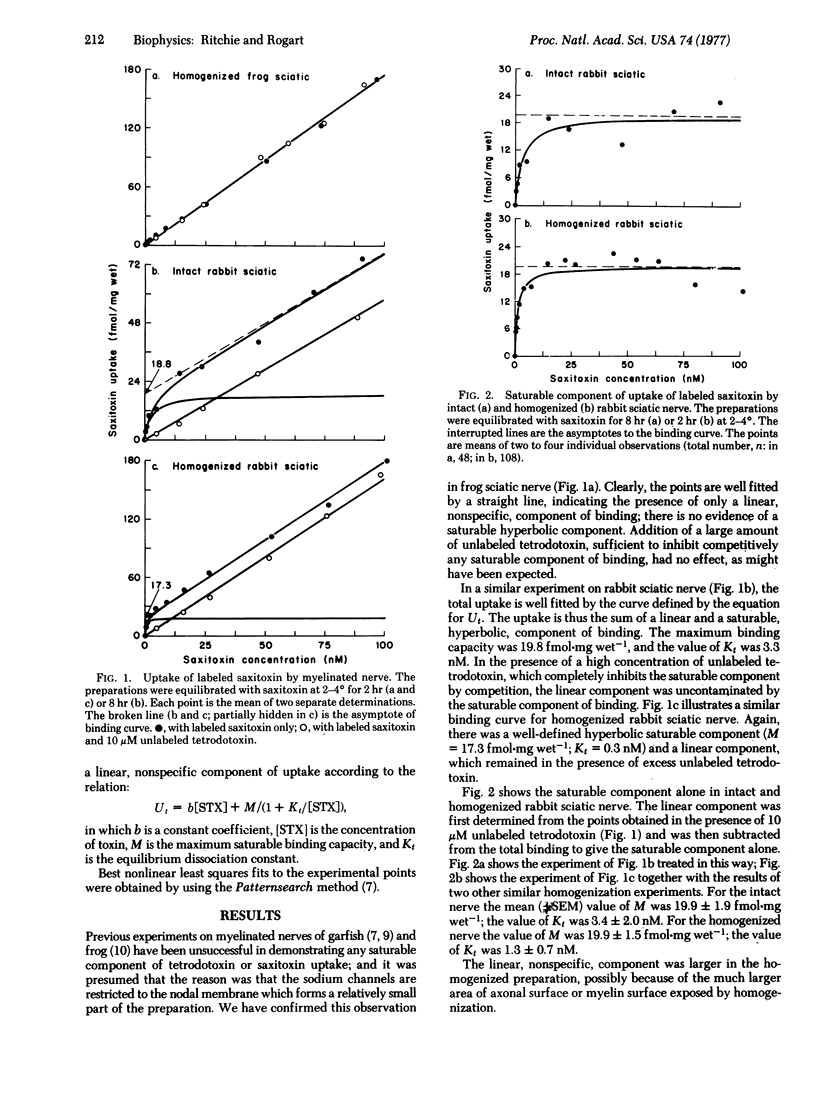
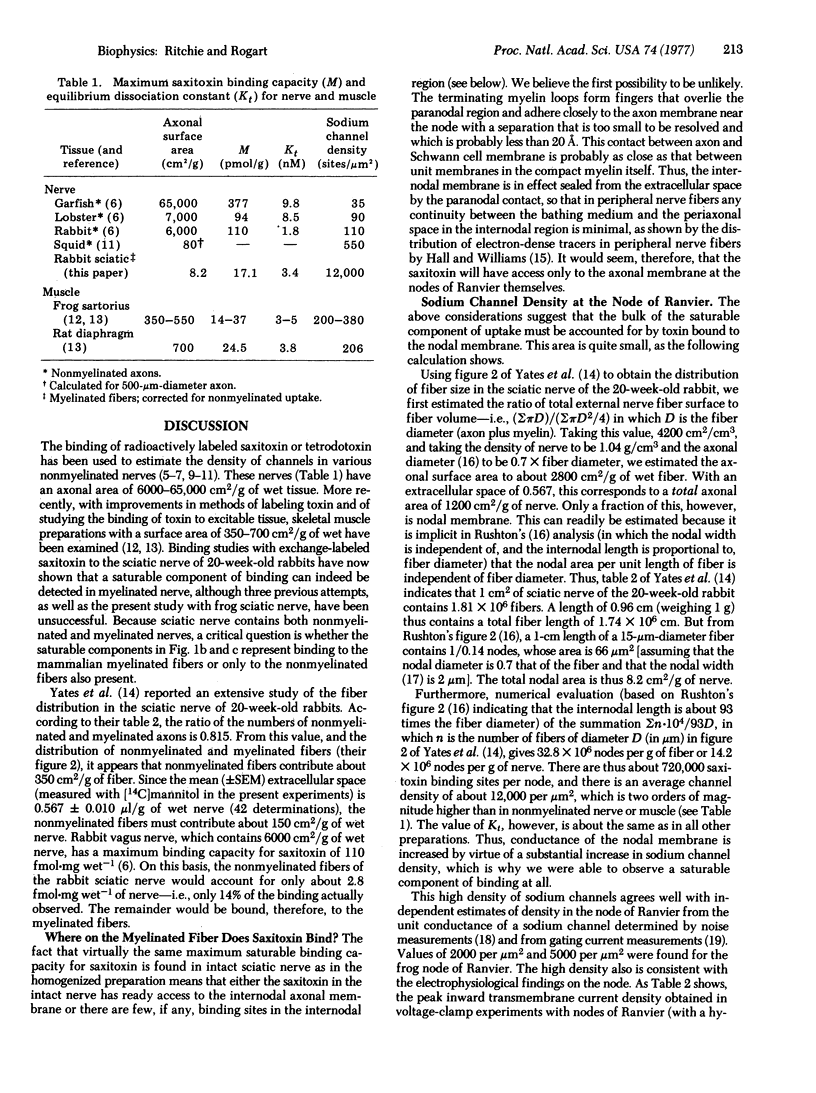
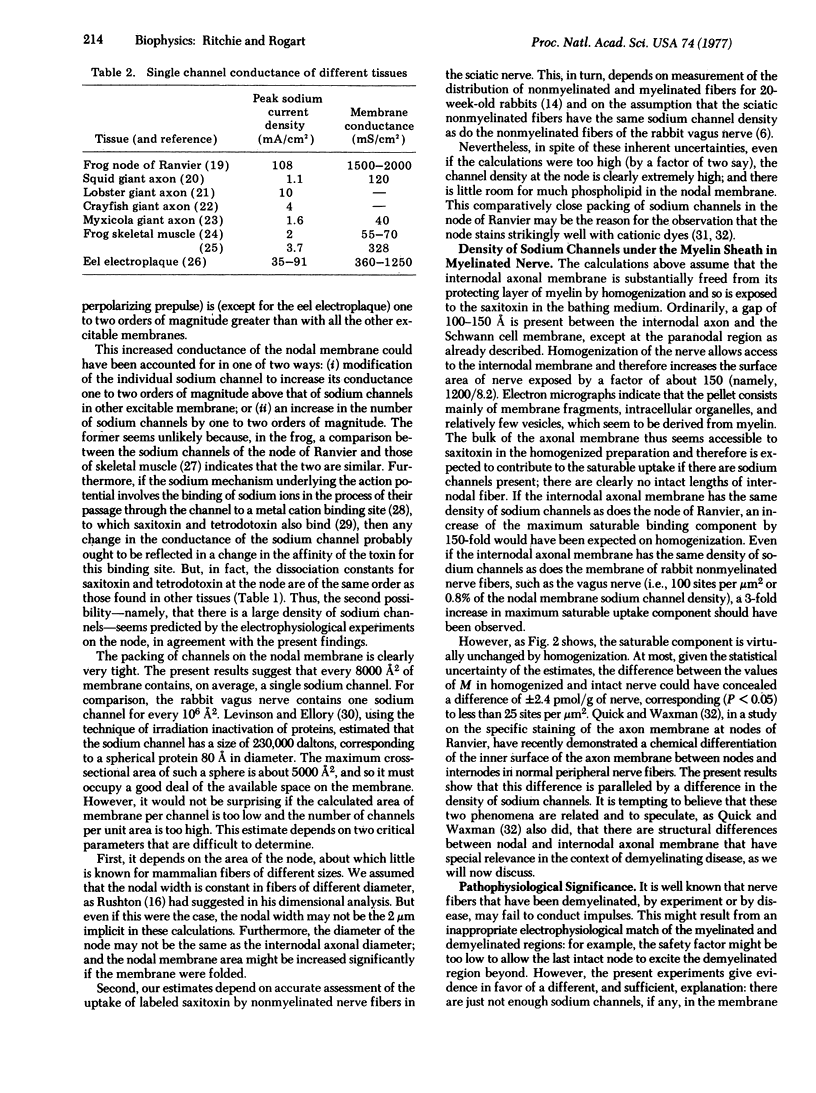
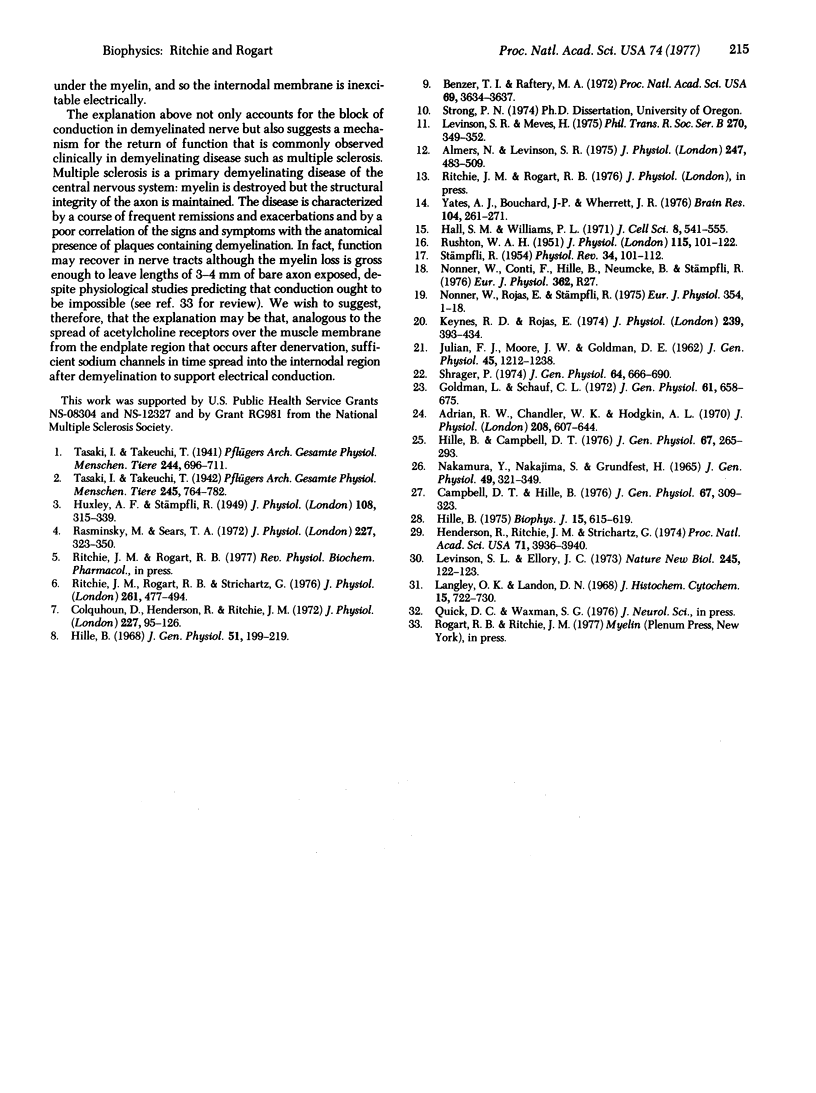
Selected References
These references are in PubMed. This may not be the complete list of references from this article.
- Adrian R. H., Chandler W. K., Hodgkin A. L. Voltage clamp experiments in striated muscle fibres. J Physiol. 1970 Jul;208(3):607–644. doi: 10.1113/jphysiol.1970.sp009139. [DOI] [PMC free article] [PubMed] [Google Scholar]
- Almers W., Levinson S. R. Tetrodotoxin binding to normal depolarized frog muscle and the conductance of a single sodium channel. J Physiol. 1975 May;247(2):483–509. doi: 10.1113/jphysiol.1975.sp010943. [DOI] [PMC free article] [PubMed] [Google Scholar]
- Benzer T. I., Raftery M. A. Partial characterization of a tetrodotoxin-binding component from nerve membrane. Proc Natl Acad Sci U S A. 1972 Dec;69(12):3634–3637. doi: 10.1073/pnas.69.12.3634. [DOI] [PMC free article] [PubMed] [Google Scholar]
- Campbell D. T., Hille B. Kinetic and pharmacological properties of the sodium channel of frog skeletal muscle. J Gen Physiol. 1976 Mar;67(3):309–323. doi: 10.1085/jgp.67.3.309. [DOI] [PMC free article] [PubMed] [Google Scholar]
- Colquhoun D., Henderson R., Ritchie J. M. The binding of labelled tetrodotoxin to non-myelinated nerve fibres. J Physiol. 1972 Dec;227(1):95–126. doi: 10.1113/jphysiol.1972.sp010022. [DOI] [PMC free article] [PubMed] [Google Scholar]
- Goldman L., Schauf C. L. Inactivation of the sodium current in Myxicola giant axons. Evidence for coupling to the activation process. J Gen Physiol. 1972 Jun;59(6):659–675. doi: 10.1085/jgp.59.6.659. [DOI] [PMC free article] [PubMed] [Google Scholar]
- HUXLEY A. F., STAMPFLI R. Evidence for saltatory conduction in peripheral myelinated nerve fibres. J Physiol. 1949 May;108(3):315–339. [PubMed] [Google Scholar]
- Hall S. M., Williams P. L. The distribution of electron-dense tracers in peripheral nerve fibres. J Cell Sci. 1971 Mar;8(2):541–555. doi: 10.1242/jcs.8.2.541. [DOI] [PubMed] [Google Scholar]
- Henderson R., Ritchie J. M., Strichartz G. R. Evidence that tetrodotoxin and saxitoxin act at a metal cation binding site in the sodium channels of nerve membrane. Proc Natl Acad Sci U S A. 1974 Oct;71(10):3936–3940. doi: 10.1073/pnas.71.10.3936. [DOI] [PMC free article] [PubMed] [Google Scholar]
- Hille B., Campbell D. T. An improved vaseline gap voltage clamp for skeletal muscle fibers. J Gen Physiol. 1976 Mar;67(3):265–293. doi: 10.1085/jgp.67.3.265. [DOI] [PMC free article] [PubMed] [Google Scholar]
- Hille B. Pharmacological modifications of the sodium channels of frog nerve. J Gen Physiol. 1968 Feb;51(2):199–219. doi: 10.1085/jgp.51.2.199. [DOI] [PMC free article] [PubMed] [Google Scholar]
- Hille B. The receptor for tetrodotoxin and saxitoxin. A structural hypothesis. Biophys J. 1975 Jun;15(6):615–619. doi: 10.1016/S0006-3495(75)85842-5. [DOI] [PMC free article] [PubMed] [Google Scholar]
- JULIAN F. J., MOORE J. W., GOLDMAN D. E. Current-voltage relations in the lobster giant axon membrane under voltage clamp conditions. J Gen Physiol. 1962 Jul;45:1217–1238. doi: 10.1085/jgp.45.6.1217. [DOI] [PMC free article] [PubMed] [Google Scholar]
- Keynes R. D., Rojas E. Kinetics and steady-state properties of the charged system controlling sodium conductance in the squid giant axon. J Physiol. 1974 Jun;239(2):393–434. doi: 10.1113/jphysiol.1974.sp010575. [DOI] [PMC free article] [PubMed] [Google Scholar]
- Langley O. K., Landon D. N. A light and electron histochemical approach to the node of Ranvier and myelin of peripheral nerve fibers. J Histochem Cytochem. 1967 Dec;15(12):722–731. doi: 10.1177/15.12.722. [DOI] [PubMed] [Google Scholar]
- Levinson S. R., Ellory J. C. Molecular size of the tetrodotoxin binding site estimated by irradiation inactivation. Nat New Biol. 1973 Sep 26;245(143):122–123. doi: 10.1038/newbio245122a0. [DOI] [PubMed] [Google Scholar]
- Levinson S. R., Meves H. The binding of tritiated tetrodotoxin to squid giant axons. Philos Trans R Soc Lond B Biol Sci. 1975 Jun 10;270(908):349–352. doi: 10.1098/rstb.1975.0014. [DOI] [PubMed] [Google Scholar]
- Nakamura Y., Nakajima S., Grundfest H. Analysis of Spike Electrogenesis and Depolarizing K Inactivation in Electroplaques of Electrophorus electricus, L. J Gen Physiol. 1965 Nov 1;49(2):321–349. doi: 10.1085/jgp.49.2.321. [DOI] [PMC free article] [PubMed] [Google Scholar]
- Nonner W., Rojas E., Stämpfli H. Displacement currents in the node of Ranvier. Voltage and time dependence. Pflugers Arch. 1975;354(1):1–18. doi: 10.1007/BF00584499. [DOI] [PubMed] [Google Scholar]
- RUSHTON W. A. H. A theory of the effects of fibre size in medullated nerve. J Physiol. 1951 Sep;115(1):101–122. doi: 10.1113/jphysiol.1951.sp004655. [DOI] [PMC free article] [PubMed] [Google Scholar]
- Rasminsky M., Sears T. A. Internodal conduction in undissected demyelinated nerve fibres. J Physiol. 1972 Dec;227(2):323–350. doi: 10.1113/jphysiol.1972.sp010035. [DOI] [PMC free article] [PubMed] [Google Scholar]
- Ritchie J. M., Rogart R. B., Strichartz G. R. A new method for labelling saxitoxin and its binding to non-myelinated fibres of the rabbit vagus, lobster walking leg, and garfish olfactory nerves. J Physiol. 1976 Oct;261(2):477–494. doi: 10.1113/jphysiol.1976.sp011569. [DOI] [PMC free article] [PubMed] [Google Scholar]
- STAMPFLI R. Saltatory conduction in nerve. Physiol Rev. 1954 Jan;34(1):101–112. doi: 10.1152/physrev.1954.34.1.101. [DOI] [PubMed] [Google Scholar]
- Shrager P. Ionic conductance changes in voltage clamped crayfish axons at low pH. J Gen Physiol. 1974 Dec;64(6):666–690. doi: 10.1085/jgp.64.6.666. [DOI] [PMC free article] [PubMed] [Google Scholar]
- Yates A. J., Bouchard J. P., Wherrett J. R. Relation of axon membrane to myelin membrane in sciatic nerve during development: comparison of morphological and chemical parameters. Brain Res. 1976 Mar 12;104(2):261–271. doi: 10.1016/0006-8993(76)90618-1. [DOI] [PubMed] [Google Scholar]