Abstract
Bacterial flagella are semi-rigid helices that undergo true rotation. In peritrichously flagellated bacteria (e.g., Escherichia and Salmonella) there are many flagella on each cell; during translational cell movement these operate as a coordinated bundle that actively disperses upon reversal of the rotation sense. The dynamic behavior of a set of helices originating on separate rotational axes is explored by a working model, geometrical analysis, and hydrodynamic calculations. A critical relationship exists between the interaxial separation and phase difference of parallel helices with overlapping domains; in the subcritical case the filaments are not intertwisted, whereas in the supercritical case they are intertwisted in the same sense (left-handed) as the helices, with one twist per helical turn. During counter-clockwise rotation (the sense operative in forward swimming) any preexisting twists of this kind are automatically cancelled and the helices brought progressively into phase. Hydrodynamic calculations suggest that some wrapping then occurs in a right-handed sense, opposite to that of the helices; this necessitates a distortion from true helical geometry which is minimized by maintaining a coaxial in-phase relationship. A highly coordinated helical bundle results that is capable of operating smoothly for an indefinite period, in agreement with the observed behavior of swimming bacteria. During reverse rotation, the supercritical case develops to cause jamming of the bundle, as has been observed with bacteria in high-viscosity medium. The explosive dispersal of the bundle during reversal in low-viscosity medium is a consequence of a complicating phenomenon, namely, a drastic change in flagellar quaternary structure. The overall conclusion is that bundle formation and function are perfectly compatible with a rotational mechanism for the individual flagella.
Full text
PDF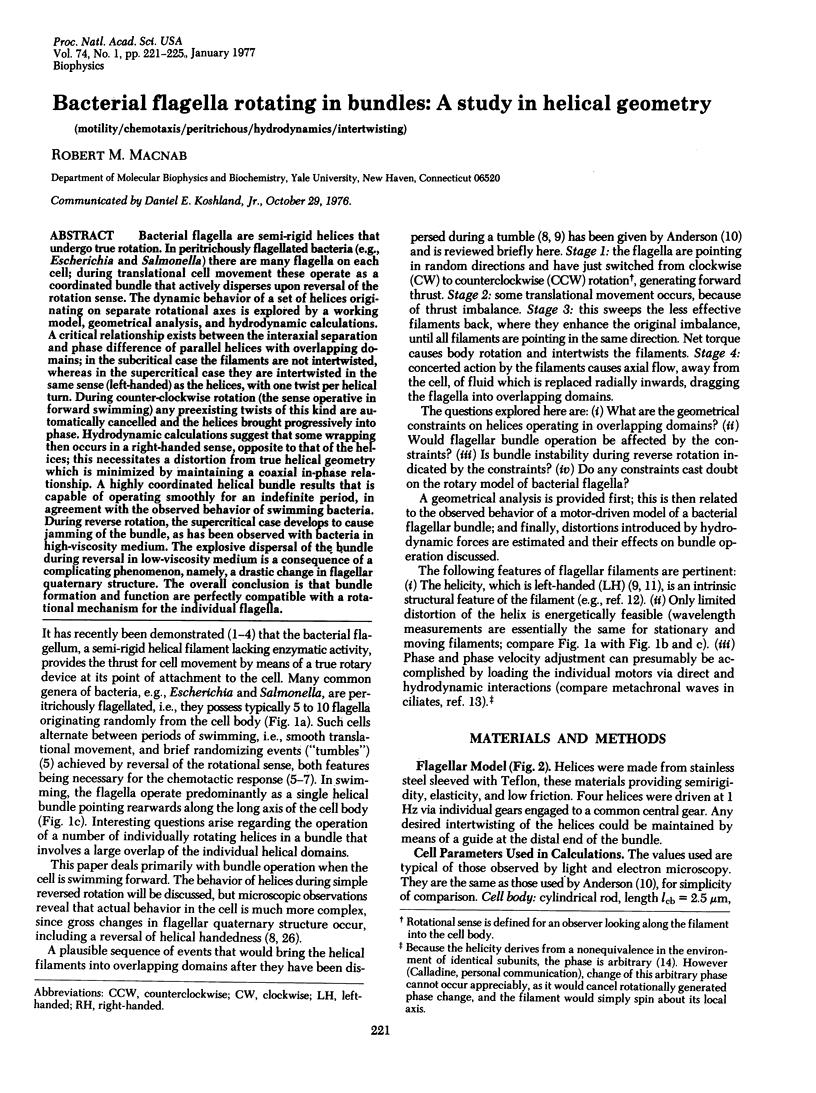
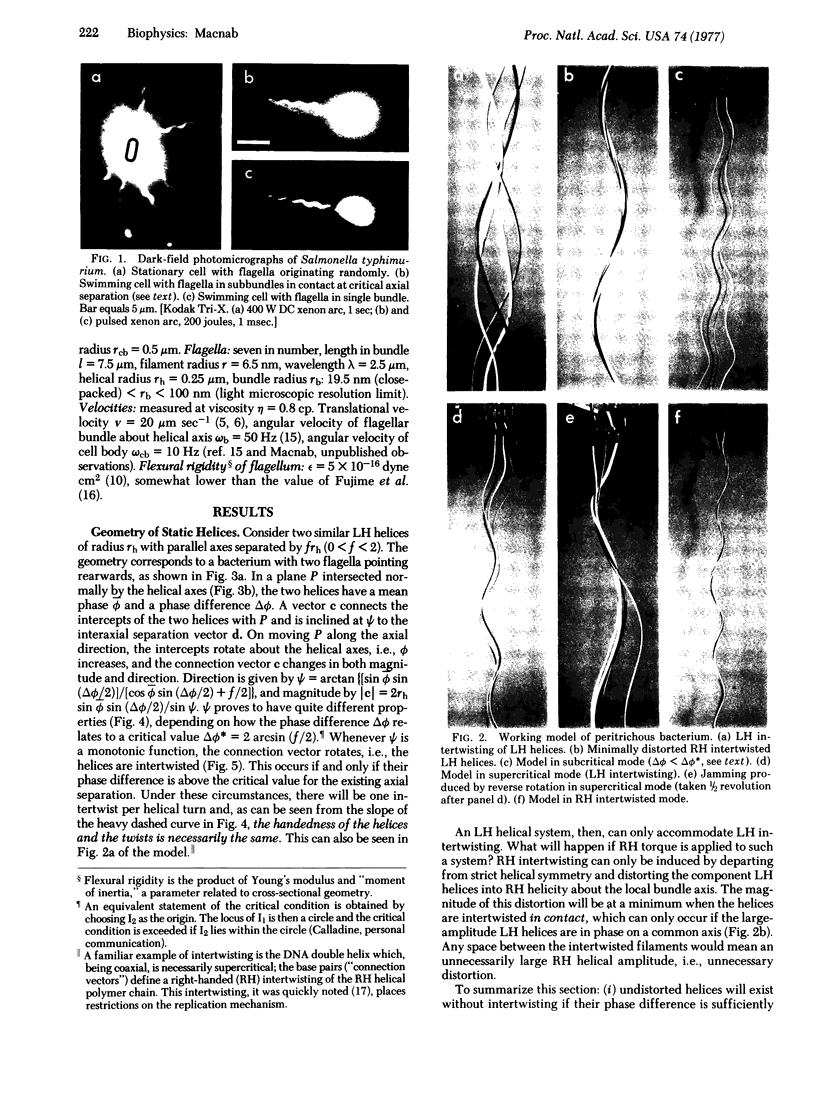
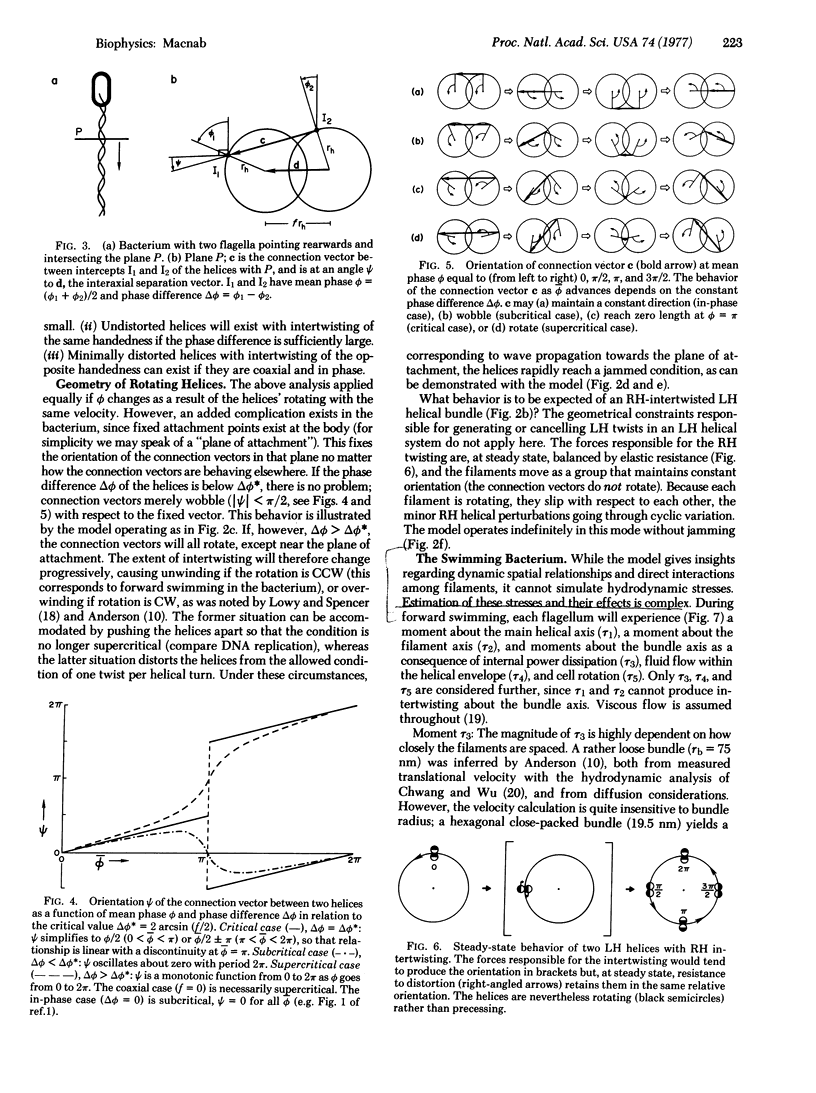
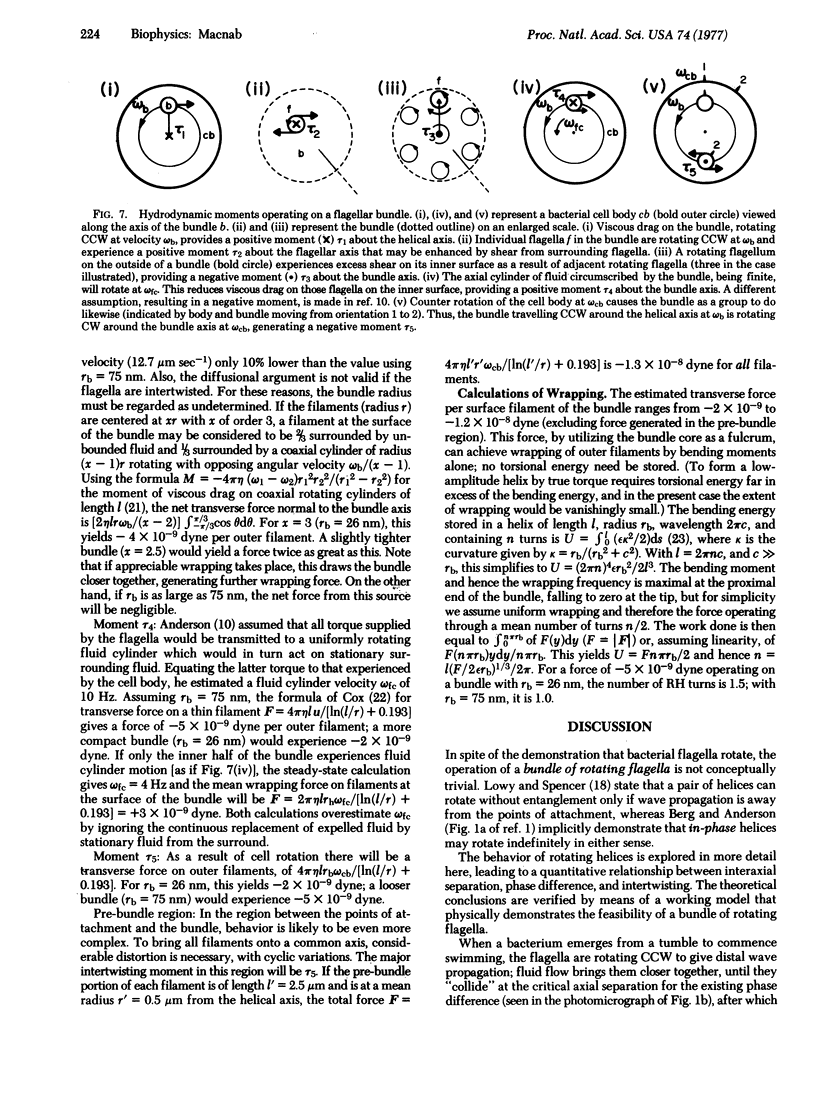
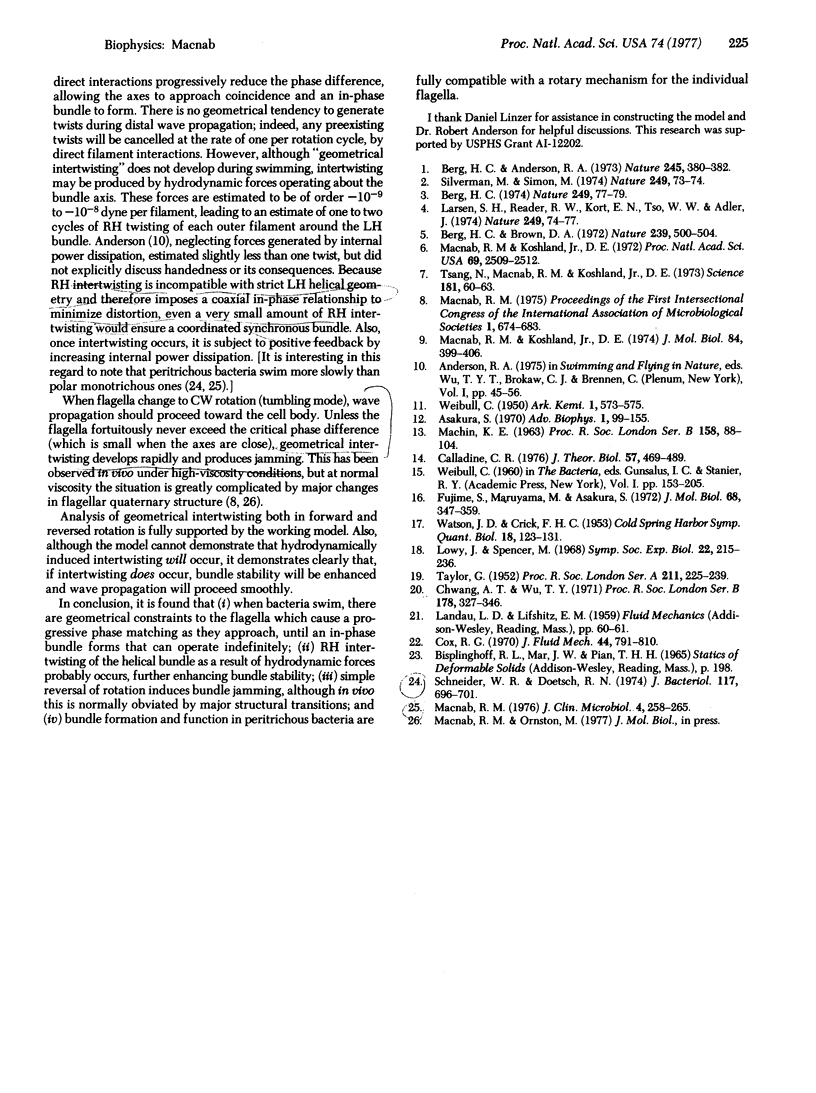
Images in this article
Selected References
These references are in PubMed. This may not be the complete list of references from this article.
- Asakura S. Polymerization of flagellin and polymorphism of flagella. Adv Biophys. 1970;1:99–155. [PubMed] [Google Scholar]
- Berg H. C., Anderson R. A. Bacteria swim by rotating their flagellar filaments. Nature. 1973 Oct 19;245(5425):380–382. doi: 10.1038/245380a0. [DOI] [PubMed] [Google Scholar]
- Berg H. C., Brown D. A. Chemotaxis in Escherichia coli analysed by three-dimensional tracking. Nature. 1972 Oct 27;239(5374):500–504. doi: 10.1038/239500a0. [DOI] [PubMed] [Google Scholar]
- Berg H. C. Dynamic properties of bacterial flagellar motors. Nature. 1974 May 3;249(452):77–79. doi: 10.1038/249077a0. [DOI] [PubMed] [Google Scholar]
- Calladine C. R. Design requirements for the construction of bacterial flagella. J Theor Biol. 1976 Apr;57(2):469–489. doi: 10.1016/0022-5193(76)90016-3. [DOI] [PubMed] [Google Scholar]
- Chwang A. T., Wu T. Y. A note on the helical movement of micro-organisms. Proc R Soc Lond B Biol Sci. 1971 Aug 3;178(1052):327–346. doi: 10.1098/rspb.1971.0068. [DOI] [PubMed] [Google Scholar]
- Fujime S., Maruyama M., Asakura S. Flexural rigidity of bacterial flagella studied by quasielastic scattering of laser light. J Mol Biol. 1972 Jul 21;68(2):347–359. doi: 10.1016/0022-2836(72)90217-3. [DOI] [PubMed] [Google Scholar]
- Larsen S. H., Reader R. W., Kort E. N., Tso W. W., Adler J. Change in direction of flagellar rotation is the basis of the chemotactic response in Escherichia coli. Nature. 1974 May 3;249(452):74–77. doi: 10.1038/249074a0. [DOI] [PubMed] [Google Scholar]
- Lowy J., Spencer M. Structure and function of bacterial flagella. Symp Soc Exp Biol. 1968;22:215–236. [PubMed] [Google Scholar]
- Macnab R. M. Examination of bacterial flagellation by dark-field microscopy. J Clin Microbiol. 1976 Sep;4(3):258–265. doi: 10.1128/jcm.4.3.258-265.1976. [DOI] [PMC free article] [PubMed] [Google Scholar]
- Macnab R. M., Koshland D. E., Jr The gradient-sensing mechanism in bacterial chemotaxis. Proc Natl Acad Sci U S A. 1972 Sep;69(9):2509–2512. doi: 10.1073/pnas.69.9.2509. [DOI] [PMC free article] [PubMed] [Google Scholar]
- Macnab R., Koshland D. E., Jr Bacterial motility and chemotaxis: light-induced tumbling response and visualization of individual flagella. J Mol Biol. 1974 Apr 15;84(3):399–406. doi: 10.1016/0022-2836(74)90448-3. [DOI] [PubMed] [Google Scholar]
- Schneider W. R., Doetsch R. N. Effect of viscosity on bacterial motility. J Bacteriol. 1974 Feb;117(2):696–701. doi: 10.1128/jb.117.2.696-701.1974. [DOI] [PMC free article] [PubMed] [Google Scholar]
- Silverman M., Simon M. Flagellar rotation and the mechanism of bacterial motility. Nature. 1974 May 3;249(452):73–74. doi: 10.1038/249073a0. [DOI] [PubMed] [Google Scholar]
- Tsang N., Macnab R., Koshland D. E., Jr Common mechanism for repellents and attractants in bacterial chemotaxis. Science. 1973 Jul 6;181(4094):60–63. doi: 10.1126/science.181.4094.60. [DOI] [PubMed] [Google Scholar]
- WATSON J. D., CRICK F. H. The structure of DNA. Cold Spring Harb Symp Quant Biol. 1953;18:123–131. doi: 10.1101/sqb.1953.018.01.020. [DOI] [PubMed] [Google Scholar]