Abstract
Evidence has been gathering from several laboratories that protons in proton-pumping membranes move along or within the bilayer rather than exchange with the bulk phase. These experiments are typically conducted on the natural membrane in vivo or in vitro or on fragments of natural membrane. Anionic lipids are present in all proton-pumping membranes. Model studies on the protonation state of the fatty acids of liposomes containing entrapped water show that the bilayers always contain mixtures of protonated and deprotonated carboxylates. Protonated fatty acids form stable acid-anion pairs with deprotonated fatty acids through unusually strong hydrogen bonds. Such acid-anion dimers have a single negative charge, which is shared by the four negative oxygens of both headgroups. The two pK values of the resulting dimer will be significantly different from the pK of the monomeric species, so that the dimer will be stable over a wide pH range. It is proposed that anionic lipid headgroups in biological membranes share protons as acid-anion dimers and that anionic lipids thus trap and conduct protons along the headgroup domain of bilayers that contain such anionic lipids. Protons pumped from the other side of the membrane may enter and move within the headgroup sheet because the protonation rate of negatively charged proton acceptors is 5 orders of magnitude faster than that of water. Protons trapped in the acidic headgroup sheet need not leave this region in order to be utilized by a responsive proton-translocating pore (a transport protein using the proton gradient). Experiments suggest the proton concentration in the headgroup domain may vary widely and the anionic lipid headgroup sheet may therefore function as a proton buffer. Due to the Gouy-Chapman-Stern layer at polyanionic surfaces, anionic lipids will also sequester protons from the bulk solution at low and moderate ionic strengths. At high ionic strength metal cations may replace protons sequestered near the headgroups, but these cations cannot substitute for protons in the "proton-conducting pathway," which is based on hydrogen bonding.
Full text
PDF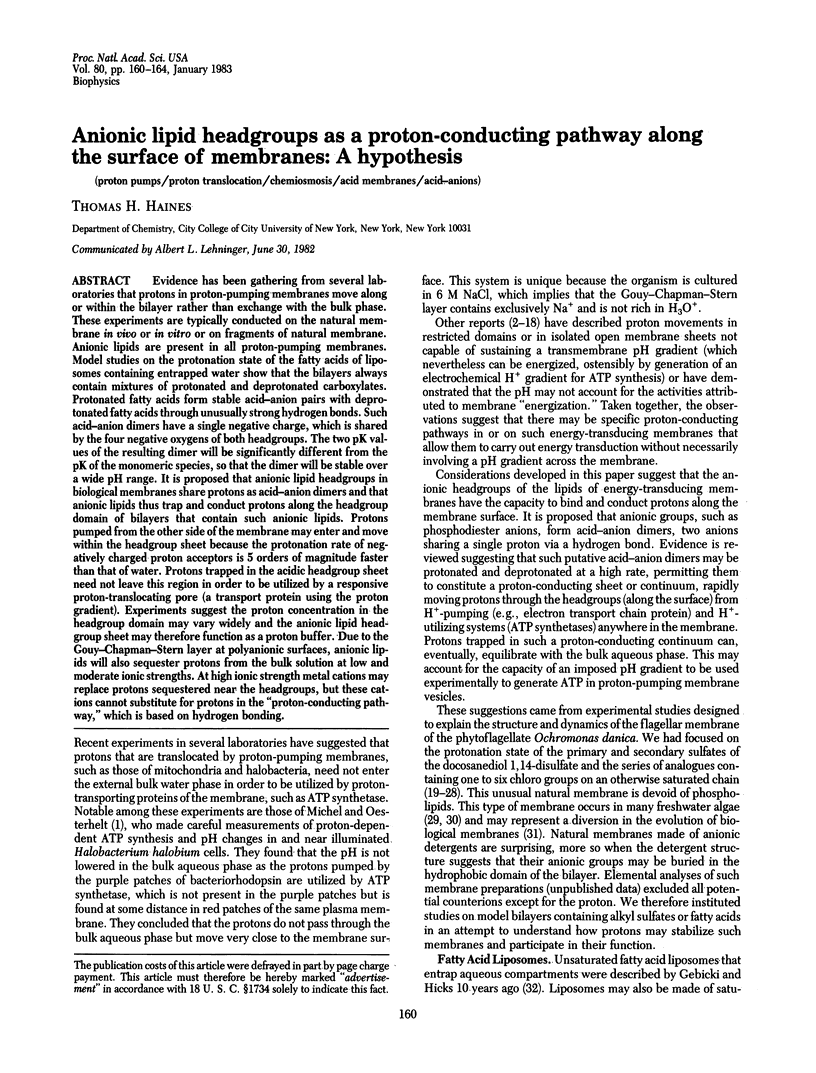
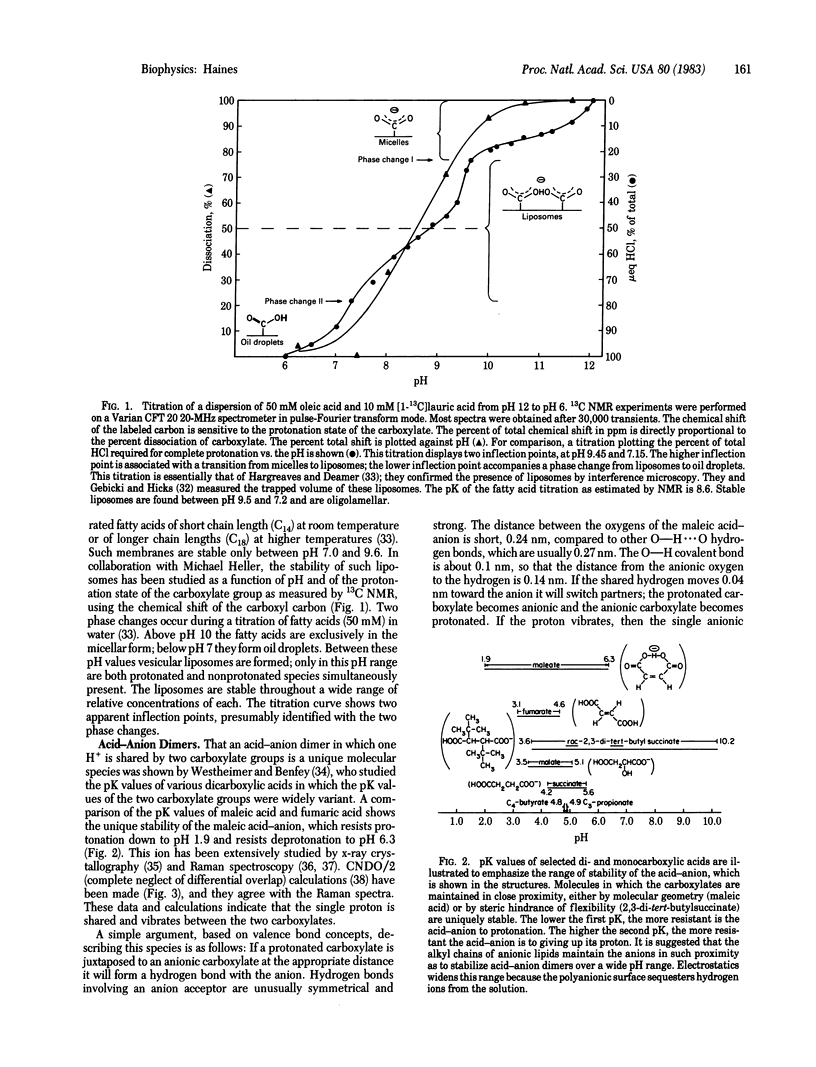
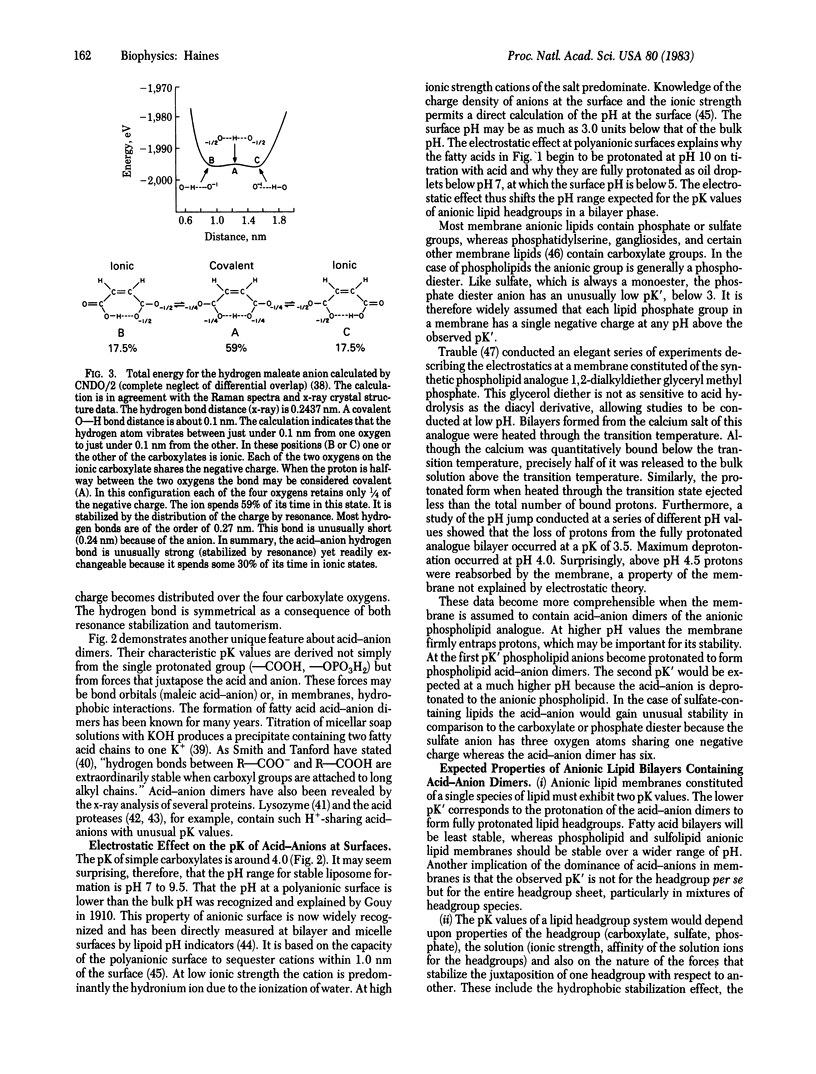
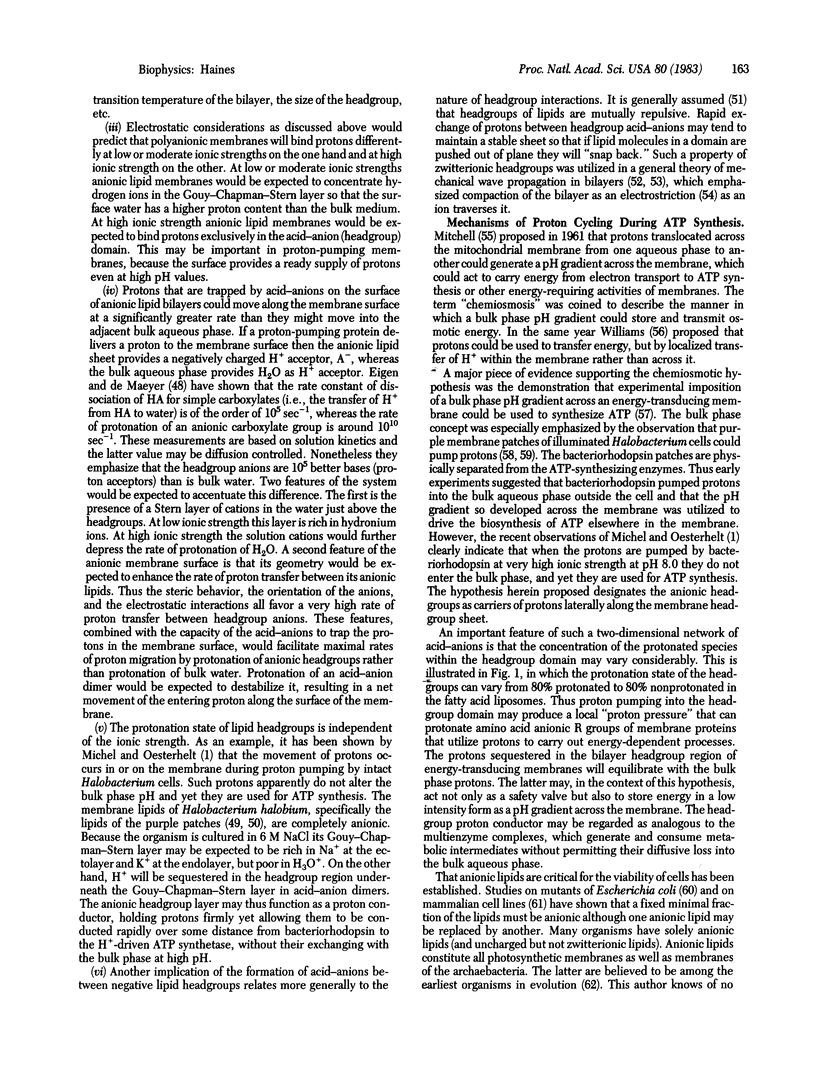
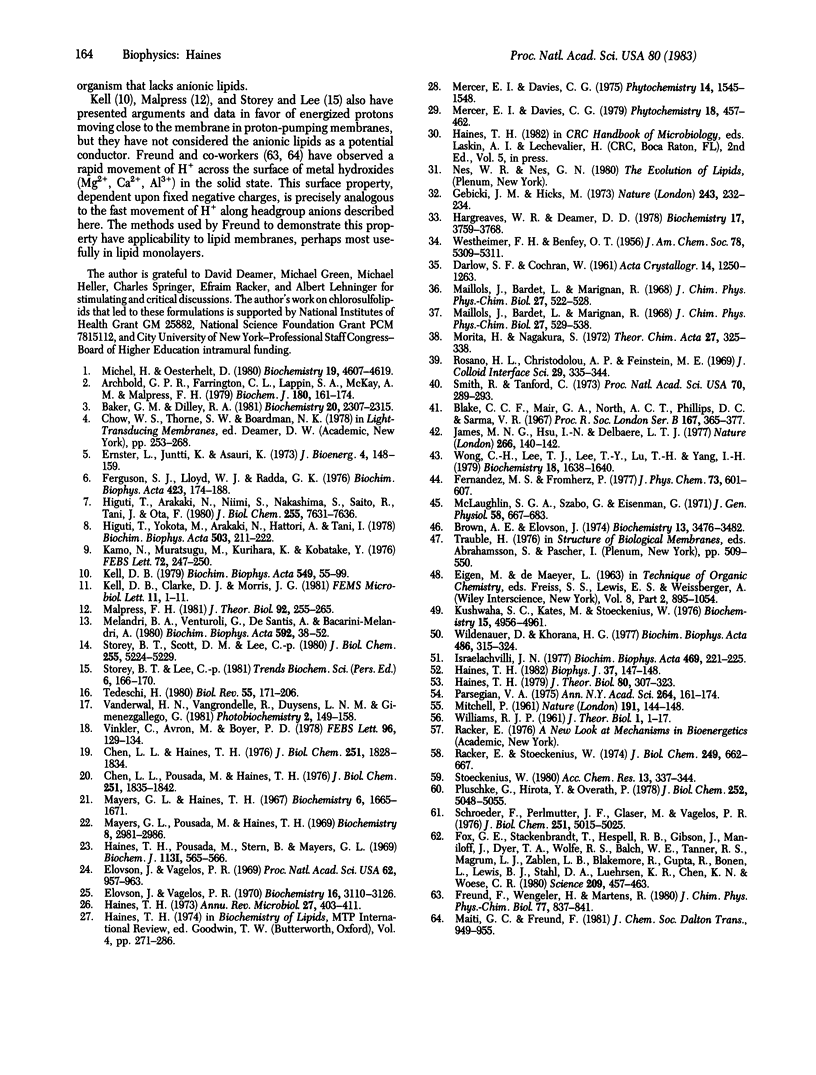
Selected References
These references are in PubMed. This may not be the complete list of references from this article.
- Archbold G. P., Farrington C. L., Lappin S. A., McKay A. M., Malpress F. H. Oxygen-pulse curves in rat liver mitochondrial suspensions. Some observations and deductions. Biochem J. 1979 Apr 15;180(1):161–174. doi: 10.1042/bj1800161. [DOI] [PMC free article] [PubMed] [Google Scholar]
- Baker G. M., Bhatnagar D., Dilley R. A. Proton release in photosynthetic water oxidation: evidence for proton movement in a restricted domain. Biochemistry. 1981 Apr 14;20(8):2307–2315. doi: 10.1021/bi00511a037. [DOI] [PubMed] [Google Scholar]
- Blake C. C., Mair G. A., North A. C., Phillips D. C., Sarma V. R. On the conformation of the hen egg-white lysozyme molecule. Proc R Soc Lond B Biol Sci. 1967 Apr 18;167(1009):365–377. doi: 10.1098/rspb.1967.0034. [DOI] [PubMed] [Google Scholar]
- Brown A. E., Elovson J. Isolation and characterization of a novel lipid, 1(3),2-diacylglyceryl-(3)-O-4'-(N,N,N-trimethyl)homoserine, from Ochromonas danica. Biochemistry. 1974 Aug 13;13(17):3476–3482. doi: 10.1021/bi00714a009. [DOI] [PubMed] [Google Scholar]
- Chen L. L., Haines T. H. The flagellar membrane of Ochromonas danica. Isolation and electrophoretic analysis of the flagellar membrane, axonemes, and mastigonemes. J Biol Chem. 1976 Mar 25;251(6):1828–1834. [PubMed] [Google Scholar]
- Chen L. L., Pousada M., Haines T. H. The flagellar membrane of Ochromonas danica. Lipid composition. J Biol Chem. 1976 Mar 25;251(6):1835–1842. [PubMed] [Google Scholar]
- Elovson J., Vagelos P. R. A new class of lipids: chlorosulfolipids. Proc Natl Acad Sci U S A. 1969 Mar;62(3):957–963. doi: 10.1073/pnas.62.3.957. [DOI] [PMC free article] [PubMed] [Google Scholar]
- Elovson J., Vagelos P. R. Structure of the major species of chlorosulfolipid from Ochromonas danica. 2,2,11,13,15,16-hexachloro-N-docosane 1,14-disulfate. Biochemistry. 1970 Aug 4;9(16):3110–3126. doi: 10.1021/bi00818a002. [DOI] [PubMed] [Google Scholar]
- Ernster L., Juntti K., Asami K. Mechanisms of energy conservation in the mitochondrial membrane. J Bioenerg. 1973 Jan;4(1):149–159. doi: 10.1007/BF01516053. [DOI] [PubMed] [Google Scholar]
- Ferguson S. J., Lloyd W. J., Radda G. K. On the nature of the energised state of submitochondrial particles; investigations with N-aryl naphthalene sulphonate probes. Biochim Biophys Acta. 1976 Feb 16;423(2):174–188. doi: 10.1016/0005-2728(76)90176-6. [DOI] [PubMed] [Google Scholar]
- Fox G. E., Stackebrandt E., Hespell R. B., Gibson J., Maniloff J., Dyer T. A., Wolfe R. S., Balch W. E., Tanner R. S., Magrum L. J. The phylogeny of prokaryotes. Science. 1980 Jul 25;209(4455):457–463. doi: 10.1126/science.6771870. [DOI] [PubMed] [Google Scholar]
- Gebicki J. M., Hicks M. Ufasomes are stable particles surrounded by unsaturated fatty acid membranes. Nature. 1973 May 25;243(5404):232–234. doi: 10.1038/243232a0. [DOI] [PubMed] [Google Scholar]
- Haines T. H. A model for transition state dynamics in bilayers: implications for the role of lipids in biomembrane transport. Biophys J. 1982 Jan;37(1):147–148. doi: 10.1016/S0006-3495(82)84644-4. [DOI] [PMC free article] [PubMed] [Google Scholar]
- Haines T. H. A proposal on the function of unsaturated fatty acids and ionic lipids: the role of potential compaction in biological membranes. J Theor Biol. 1979 Oct 7;80(3):307–323. doi: 10.1016/0022-5193(79)90096-1. [DOI] [PubMed] [Google Scholar]
- Haines T. H. Halogen- and sulfur-containing lipids of Ochromonas. Annu Rev Microbiol. 1973;27:403–411. doi: 10.1146/annurev.mi.27.100173.002155. [DOI] [PubMed] [Google Scholar]
- Haines T. H., Pousada M., Stern B., Mayers G. L. Microbial sulpholipids: (R)-13-choro-1-(R)-14-docosanediol disulphate and polychlorosulpholipids in Ochromonas danica. Biochem J. 1969 Jul;113(3):565–566. doi: 10.1042/bj1130565. [DOI] [PMC free article] [PubMed] [Google Scholar]
- Hargreaves W. R., Deamer D. W. Liposomes from ionic, single-chain amphiphiles. Biochemistry. 1978 Sep 5;17(18):3759–3768. doi: 10.1021/bi00611a014. [DOI] [PubMed] [Google Scholar]
- Higuti T., Arakaki N., Niimi S., Nakasima S., Saito R., Tani I., Ota F. Anisotropic inhibition of energy transduction in oxidative phosphorylation in rat liver mitochondria by tetraphenylarsonium. J Biol Chem. 1980 Aug 25;255(16):7631–7636. [PubMed] [Google Scholar]
- Higuti T., Yokota M., Arakaki N., Hattori A., Tani I. Sidedness of inhibition of energy transduction in oxidative phosphorylation in rat liver mitochondria by ethidium bromide. Biochim Biophys Acta. 1978 Aug 8;503(2):211–222. doi: 10.1016/0005-2728(78)90183-4. [DOI] [PubMed] [Google Scholar]
- Hsu I. N., Delbaere L. T., James M. N., Hofmann T. Penicillopepsin from Penicillium janthinellum crystal structure at 2.8 A and sequence homology with porcine pepsin. Nature. 1977 Mar 10;266(5598):140–145. doi: 10.1038/266140a0. [DOI] [PubMed] [Google Scholar]
- Israelachvili J. N. Refinement of the fluid-mosaic model of membrane structure. Biochim Biophys Acta. 1977 Sep 5;469(2):221–225. doi: 10.1016/0005-2736(77)90185-7. [DOI] [PubMed] [Google Scholar]
- Kamo N., Muratsugu M., Kurihara K., Kobatake Y. Change in surface charge density and membrane potential of intact mitochondria during energization. FEBS Lett. 1976 Dec 31;72(2):247–250. doi: 10.1016/0014-5793(76)80979-9. [DOI] [PubMed] [Google Scholar]
- Kell D. B. On the functional proton current pathway of electron transport phosphorylation. An electrodic view. Biochim Biophys Acta. 1979 Jul 3;549(1):55–99. doi: 10.1016/0304-4173(79)90018-1. [DOI] [PubMed] [Google Scholar]
- Kuriki Y., Halsey J., Biltonen R., Racker E. Calorimetric studies of the interaction of magnesium and phosphate with Na+, K+) ATPase: evidence for a ligand-induced conformational change in the enzyme. Biochemistry. 1976 Nov 16;15(23):4956–4961. doi: 10.1021/bi00668a002. [DOI] [PubMed] [Google Scholar]
- MITCHELL P. Coupling of phosphorylation to electron and hydrogen transfer by a chemi-osmotic type of mechanism. Nature. 1961 Jul 8;191:144–148. doi: 10.1038/191144a0. [DOI] [PubMed] [Google Scholar]
- Malpress F. H. On the chemiosmotic hypothesis and the nature of the mitochondrial protonmotive force. J Theor Biol. 1981 Oct 7;92(3):255–265. doi: 10.1016/0022-5193(81)90291-5. [DOI] [PubMed] [Google Scholar]
- Mayers G. L., Haines T. H. A microbial sulfolipid. II. Structural studies. Biochemistry. 1967 Jun;6(6):1665–1671. doi: 10.1021/bi00858a013. [DOI] [PubMed] [Google Scholar]
- Mayers G. L., Pousada M., Haines T. H. Microbial sulfolipids. 3. The disulfate of (+)-1,14-docosanediol in Ochromonas danica. Biochemistry. 1969 Jul;8(7):2981–2986. doi: 10.1021/bi00835a045. [DOI] [PubMed] [Google Scholar]
- McLaughlin S. G., Szabo G., Eisenman G. Divalent ions and the surface potential of charged phospholipid membranes. J Gen Physiol. 1971 Dec;58(6):667–687. doi: 10.1085/jgp.58.6.667. [DOI] [PMC free article] [PubMed] [Google Scholar]
- Melandri B. A., Venturoli G., de Santis A., Baccarini-Melandri A. The induction kinetics of bacterial photophosphorylation. Threshold effects by the phosphate potential and correlation with the amplitude of the carotenoid absorption band shift. Biochim Biophys Acta. 1980 Aug 5;592(1):38–52. doi: 10.1016/0005-2728(80)90112-7. [DOI] [PubMed] [Google Scholar]
- Michel H., Oesterhelt D. Electrochemical proton gradient across the cell membrane of Halobacterium halobium: effect of N,N'-dicyclohexylcarbodiimide, relation to intracellular adenosine triphosphate, adenosine diphosphate, and phosphate concentration, and influence of the potassium gradient. Biochemistry. 1980 Sep 30;19(20):4607–4614. doi: 10.1021/bi00561a011. [DOI] [PubMed] [Google Scholar]
- Parsegian V. A. Ion-membrane interactions as structural forces. Ann N Y Acad Sci. 1975 Dec 30;264:161–171. doi: 10.1111/j.1749-6632.1975.tb31481.x. [DOI] [PubMed] [Google Scholar]
- Pluschke G., Hirota Y., Overath P. Function of phospholipids in Escherichia coli. Characterization of a mutant deficient in cardiolipin synthesis. J Biol Chem. 1978 Jul 25;253(14):5048–5055. [PubMed] [Google Scholar]
- Racker E., Stoeckenius W. Reconstitution of purple membrane vesicles catalyzing light-driven proton uptake and adenosine triphosphate formation. J Biol Chem. 1974 Jan 25;249(2):662–663. [PubMed] [Google Scholar]
- Rosano H. L., Christodoulou A. P., Feinstein M. E. Competition of cations at charged micelle and monolayer interfaces. J Colloid Interface Sci. 1969 Feb;29(2):335–344. [PubMed] [Google Scholar]
- Schroeder F., Perlmutter J. F., Glaser M., Vagelos P. R. Isolation and characterization of subcellular membranes with altered phospholipid composition from cultured fibroblasts. J Biol Chem. 1976 Aug 25;251(16):5015–5026. [PubMed] [Google Scholar]
- Smith R., Tanford C. Hydrophobicity of Long Chain n-Alkyl Carboxylic Acids, as Measured by Their Distribution Between Heptane and Aqueous Solutions. Proc Natl Acad Sci U S A. 1973 Feb;70(2):289–293. doi: 10.1073/pnas.70.2.289. [DOI] [PMC free article] [PubMed] [Google Scholar]
- Storey B. T., Scott D. M., Lee C. Energy-linked quinacrine fluorescence changes in submitochondrial particles from skeletal muscle mitochondria. Evidence for intramembrane H+ transfer as a primary reaction of energy coupling. J Biol Chem. 1980 Jun 10;255(11):5224–5229. [PubMed] [Google Scholar]
- Tedeschi H. The mitochondrial membrane potential. Biol Rev Camb Philos Soc. 1980 May;55(2):171–206. doi: 10.1111/j.1469-185x.1980.tb00692.x. [DOI] [PubMed] [Google Scholar]
- WILLIAMS R. J. Possible functions of chains of catalysts. J Theor Biol. 1961 Jan;1:1–17. doi: 10.1016/0022-5193(61)90023-6. [DOI] [PubMed] [Google Scholar]
- Wildenauer D., Khorana H. G. The preparation of lipid-depleted bacteriorhodopsin. Biochim Biophys Acta. 1977 Apr 18;466(2):315–324. doi: 10.1016/0005-2736(77)90227-9. [DOI] [PubMed] [Google Scholar]
- Wong C. H., Lee T. J., Lee T. Y., Lu T. H., Yang I. H. Structure of acid protease from Endothia parasitica in cross-linked form at 2.45-A resolution. Biochemistry. 1979 Apr 17;18(8):1638–1640. doi: 10.1021/bi00575a041. [DOI] [PubMed] [Google Scholar]