Abstract
The biochemistry of visual excitation is kinetically explored by measuring the activity of the cGMP phosphodiesterase (PDE) at light levels that activate only a few tens of rhodopsin molecules per rod. At 23 degrees C and in the presence of ATP, the pulse of PDE activity lasts 4 s (full width at half maximum). Complementing the rod outer segments (ROS) with rhodopsin kinase (RK) and arrestin or its splice variant p44 does not significantly shorten the pulse. But when the ROS are washed, the duration of the signal doubles. Adding either arrestin or p44 back to washed ROS approximately restores the pulse width to its initial value, with p44 being 10 times more efficient than arrestin. This supports the idea that, in vivo, capping of phosphorylated R* is mostly done by p44. When myristoylated (14:0) recoverin is added to unwashed ROS, the pulse duration and amplitude increase by about 50% if the free calcium is 500 nM. This effect increases further if the calcium is raised to 1 microM. Whenever R* deactivation is changed--when RK is exogenously enriched or when ATP is omitted from the buffer--there is no impact on the rising slope of the PDE pulse but only on its amplitude and duration. We explain this effect as due to the unequal competition between transducin and RK for R*. The kinetic model issued from this idea fits the data well, and its prediction that enrichment with transducin should lengthen the PDE pulse is successfully validated.
Full text
PDF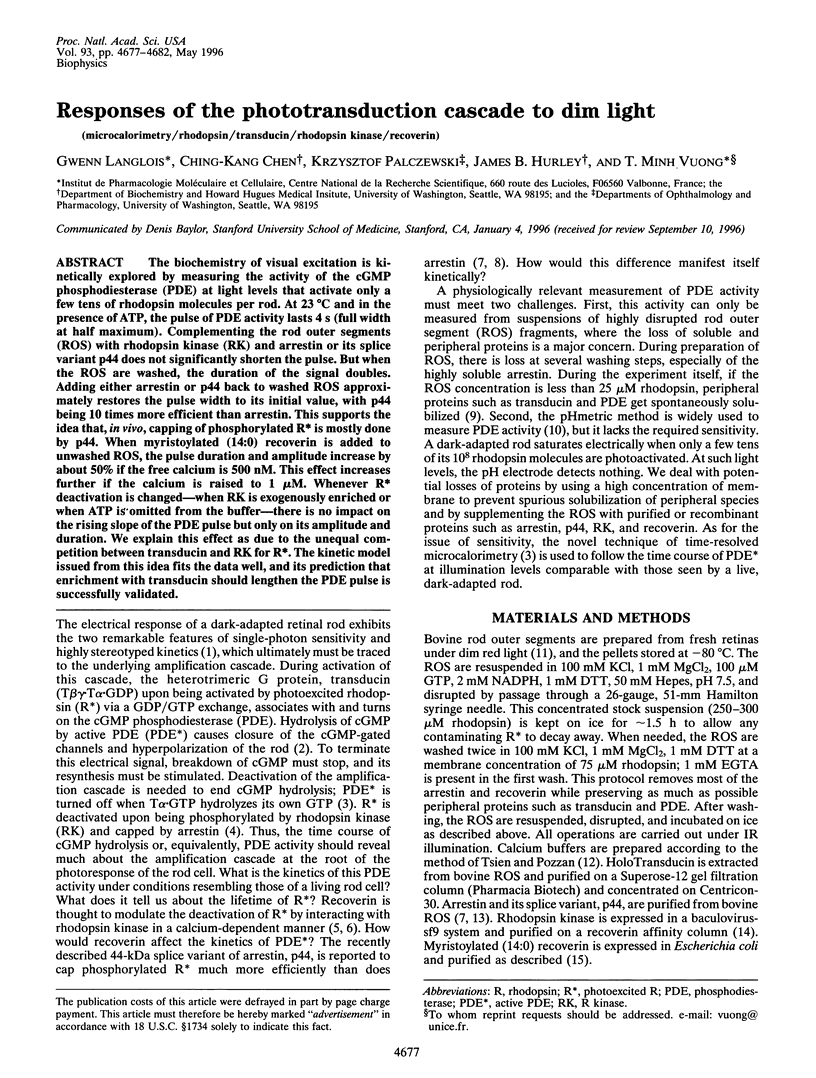
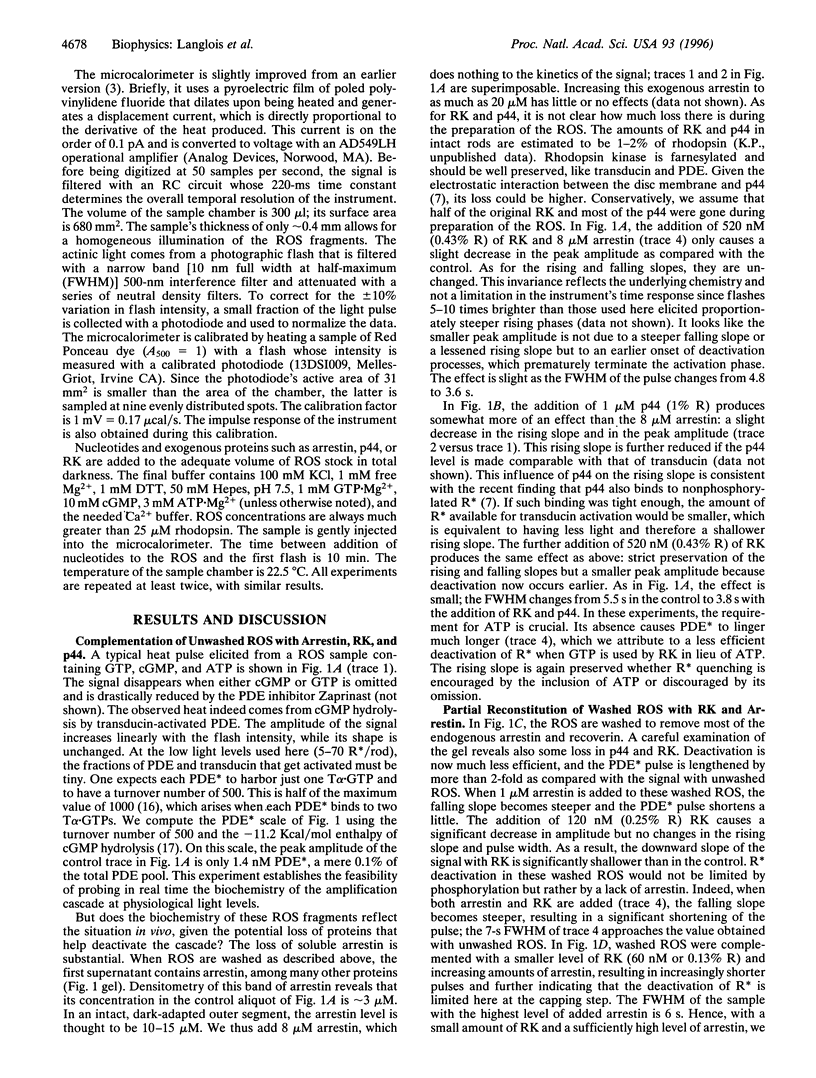
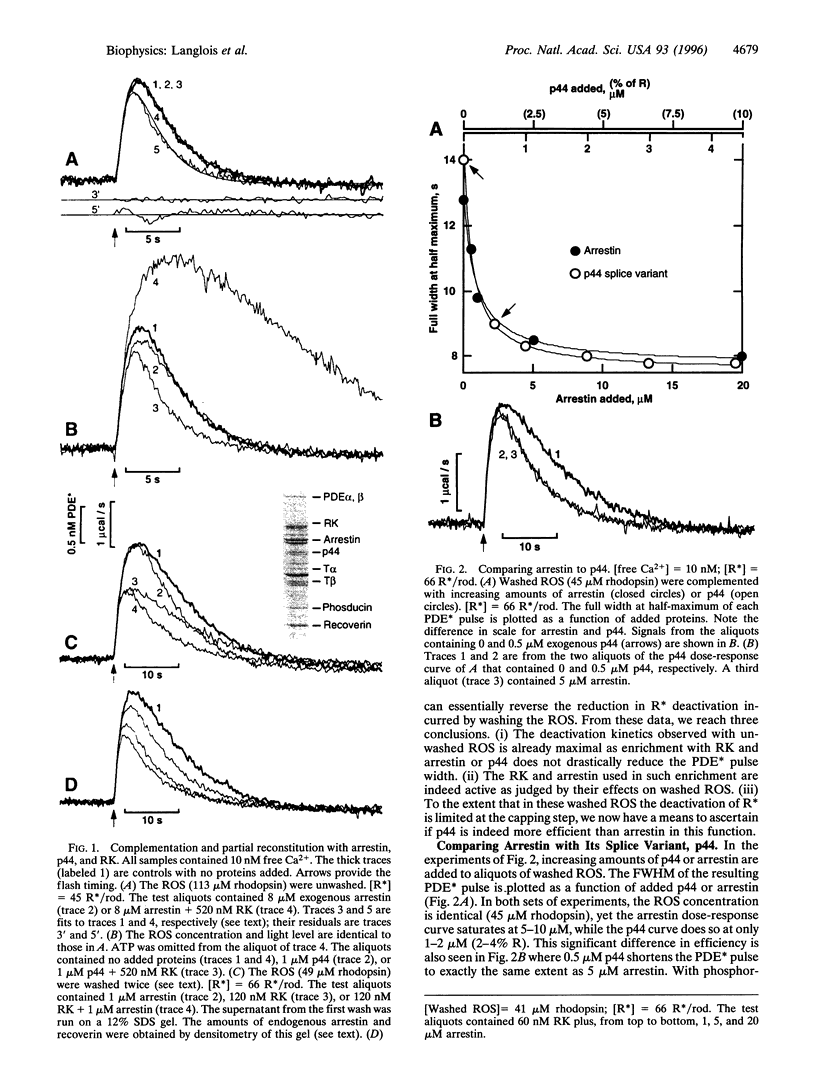
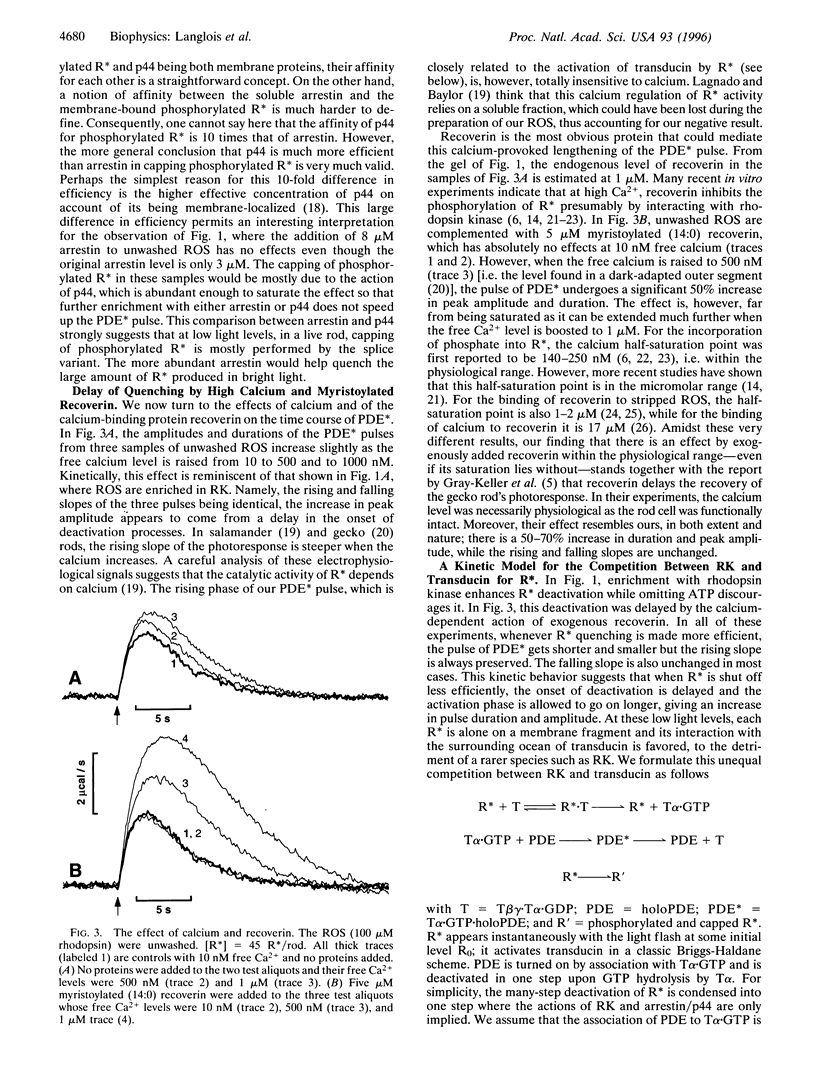
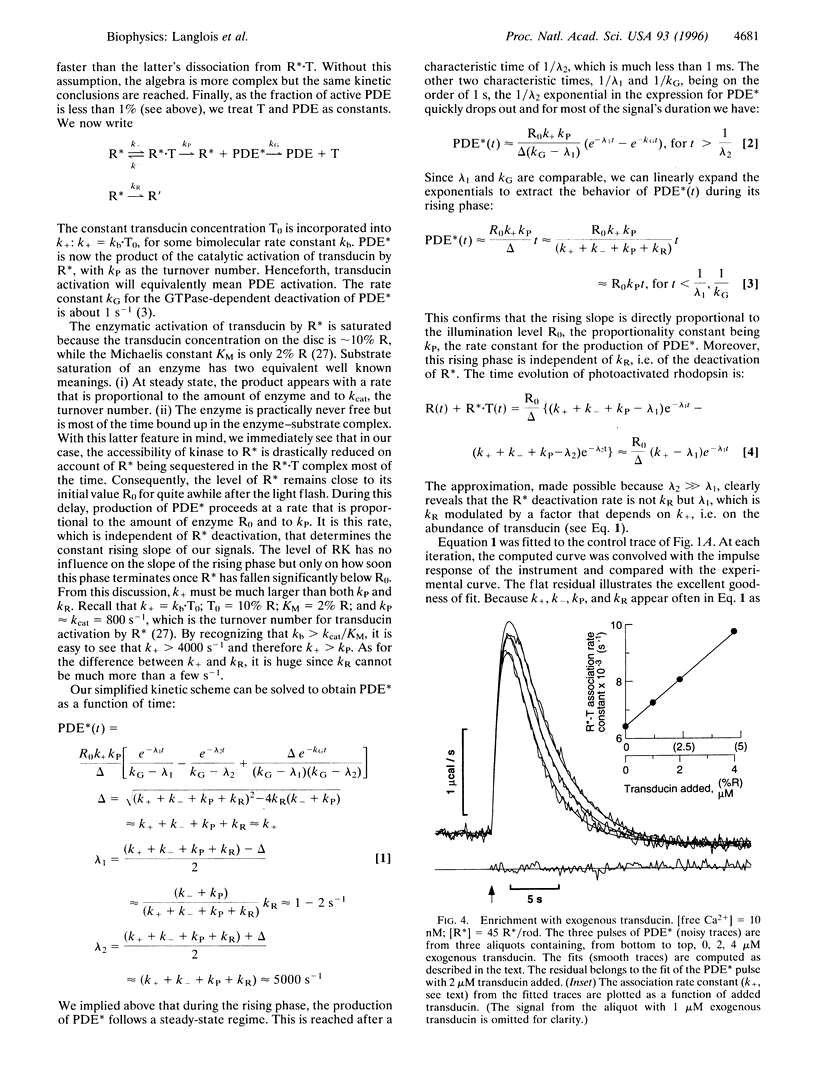
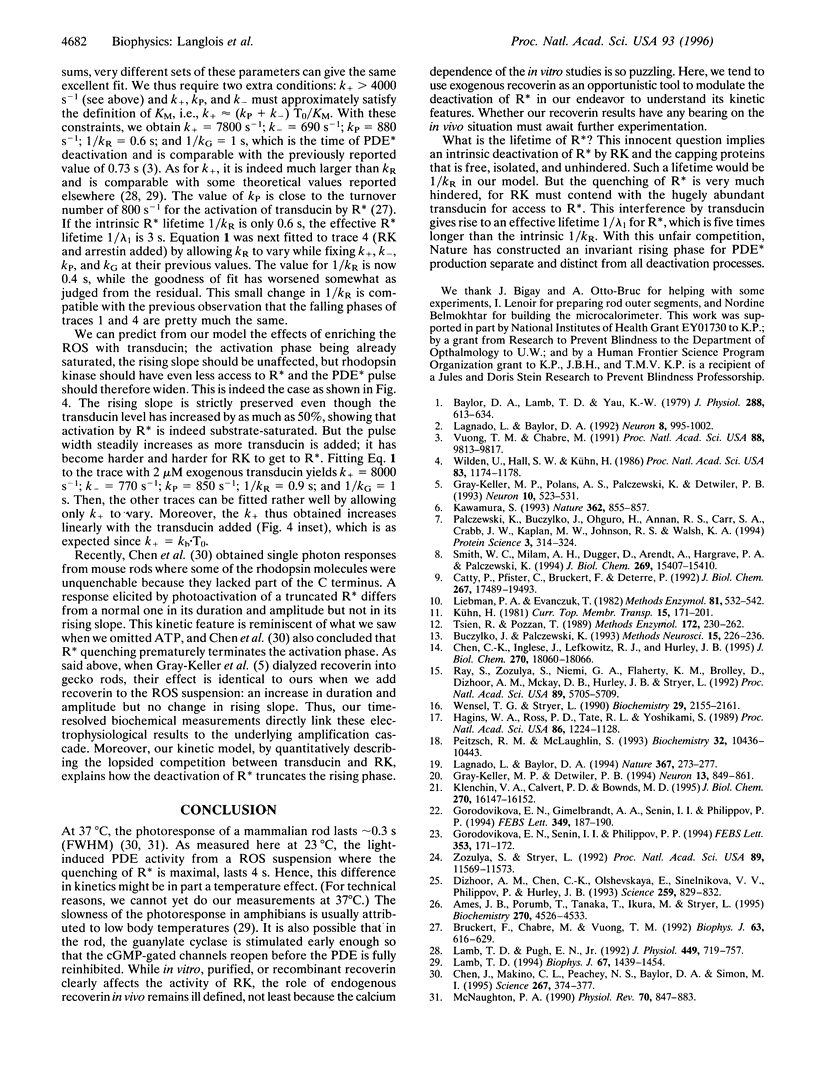
Images in this article
Selected References
These references are in PubMed. This may not be the complete list of references from this article.
- Ames J. B., Porumb T., Tanaka T., Ikura M., Stryer L. Amino-terminal myristoylation induces cooperative calcium binding to recoverin. J Biol Chem. 1995 Mar 3;270(9):4526–4533. doi: 10.1074/jbc.270.9.4526. [DOI] [PubMed] [Google Scholar]
- Baylor D. A., Lamb T. D., Yau K. W. Responses of retinal rods to single photons. J Physiol. 1979 Mar;288:613–634. [PMC free article] [PubMed] [Google Scholar]
- Bruckert F., Chabre M., Vuong T. M. Kinetic analysis of the activation of transducin by photoexcited rhodopsin. Influence of the lateral diffusion of transducin and competition of guanosine diphosphate and guanosine triphosphate for the nucleotide site. Biophys J. 1992 Sep;63(3):616–629. doi: 10.1016/S0006-3495(92)81650-8. [DOI] [PMC free article] [PubMed] [Google Scholar]
- Catty P., Pfister C., Bruckert F., Deterre P. The cGMP phosphodiesterase-transducin complex of retinal rods. Membrane binding and subunits interactions. J Biol Chem. 1992 Sep 25;267(27):19489–19493. [PubMed] [Google Scholar]
- Chen C. K., Inglese J., Lefkowitz R. J., Hurley J. B. Ca(2+)-dependent interaction of recoverin with rhodopsin kinase. J Biol Chem. 1995 Jul 28;270(30):18060–18066. doi: 10.1074/jbc.270.30.18060. [DOI] [PubMed] [Google Scholar]
- Chen J., Makino C. L., Peachey N. S., Baylor D. A., Simon M. I. Mechanisms of rhodopsin inactivation in vivo as revealed by a COOH-terminal truncation mutant. Science. 1995 Jan 20;267(5196):374–377. doi: 10.1126/science.7824934. [DOI] [PubMed] [Google Scholar]
- Dizhoor A. M., Chen C. K., Olshevskaya E., Sinelnikova V. V., Phillipov P., Hurley J. B. Role of the acylated amino terminus of recoverin in Ca(2+)-dependent membrane interaction. Science. 1993 Feb 5;259(5096):829–832. doi: 10.1126/science.8430337. [DOI] [PubMed] [Google Scholar]
- Gorodovikova E. N., Gimelbrant A. A., Senin I. I., Philippov P. P. Recoverin mediates the calcium effect upon rhodopsin phosphorylation and cGMP hydrolysis in bovine retina rod cells. FEBS Lett. 1994 Aug 1;349(2):187–190. doi: 10.1016/0014-5793(94)00661-x. [DOI] [PubMed] [Google Scholar]
- Gorodovikova E. N., Senin I. I., Philippov P. P. Calcium-sensitive control of rhodopsin phosphorylation in the reconstituted system consisting of photoreceptor membranes, rhodopsin kinase and recoverin. FEBS Lett. 1994 Oct 17;353(2):171–172. doi: 10.1016/0014-5793(94)01030-7. [DOI] [PubMed] [Google Scholar]
- Gray-Keller M. P., Detwiler P. B. The calcium feedback signal in the phototransduction cascade of vertebrate rods. Neuron. 1994 Oct;13(4):849–861. doi: 10.1016/0896-6273(94)90251-8. [DOI] [PubMed] [Google Scholar]
- Gray-Keller M. P., Polans A. S., Palczewski K., Detwiler P. B. The effect of recoverin-like calcium-binding proteins on the photoresponse of retinal rods. Neuron. 1993 Mar;10(3):523–531. doi: 10.1016/0896-6273(93)90339-s. [DOI] [PubMed] [Google Scholar]
- Hagins W. A., Ross P. D., Tate R. L., Yoshikami S. Transduction heats in retinal rods: tests of the role of cGMP by pyroelectric calorimetry. Proc Natl Acad Sci U S A. 1989 Feb;86(4):1224–1228. doi: 10.1073/pnas.86.4.1224. [DOI] [PMC free article] [PubMed] [Google Scholar]
- Kawamura S. Rhodopsin phosphorylation as a mechanism of cyclic GMP phosphodiesterase regulation by S-modulin. Nature. 1993 Apr 29;362(6423):855–857. doi: 10.1038/362855a0. [DOI] [PubMed] [Google Scholar]
- Klenchin V. A., Calvert P. D., Bownds M. D. Inhibition of rhodopsin kinase by recoverin. Further evidence for a negative feedback system in phototransduction. J Biol Chem. 1995 Jul 7;270(27):16147–16152. doi: 10.1074/jbc.270.27.16147. [DOI] [PubMed] [Google Scholar]
- Lagnado L., Baylor D. A. Calcium controls light-triggered formation of catalytically active rhodopsin. Nature. 1994 Jan 20;367(6460):273–277. doi: 10.1038/367273a0. [DOI] [PubMed] [Google Scholar]
- Lagnado L., Baylor D. Signal flow in visual transduction. Neuron. 1992 Jun;8(6):995–1002. doi: 10.1016/0896-6273(92)90122-t. [DOI] [PubMed] [Google Scholar]
- Lamb T. D., Pugh E. N., Jr A quantitative account of the activation steps involved in phototransduction in amphibian photoreceptors. J Physiol. 1992 Apr;449:719–758. doi: 10.1113/jphysiol.1992.sp019111. [DOI] [PMC free article] [PubMed] [Google Scholar]
- Lamb T. D. Stochastic simulation of activation in the G-protein cascade of phototransduction. Biophys J. 1994 Oct;67(4):1439–1454. doi: 10.1016/S0006-3495(94)80617-4. [DOI] [PMC free article] [PubMed] [Google Scholar]
- Liebman P. A., Evanczuk A. T. Real time assay of rod disk membrane cGMP phosphodiesterase and its controller enzymes. Methods Enzymol. 1982;81:532–542. doi: 10.1016/s0076-6879(82)81074-4. [DOI] [PubMed] [Google Scholar]
- McNaughton P. A. Light response of vertebrate photoreceptors. Physiol Rev. 1990 Jul;70(3):847–883. doi: 10.1152/physrev.1990.70.3.847. [DOI] [PubMed] [Google Scholar]
- Palczewski K., Buczylko J., Ohguro H., Annan R. S., Carr S. A., Crabb J. W., Kaplan M. W., Johnson R. S., Walsh K. A. Characterization of a truncated form of arrestin isolated from bovine rod outer segments. Protein Sci. 1994 Feb;3(2):314–324. doi: 10.1002/pro.5560030215. [DOI] [PMC free article] [PubMed] [Google Scholar]
- Peitzsch R. M., McLaughlin S. Binding of acylated peptides and fatty acids to phospholipid vesicles: pertinence to myristoylated proteins. Biochemistry. 1993 Oct 5;32(39):10436–10443. doi: 10.1021/bi00090a020. [DOI] [PubMed] [Google Scholar]
- Ray S., Zozulya S., Niemi G. A., Flaherty K. M., Brolley D., Dizhoor A. M., McKay D. B., Hurley J., Stryer L. Cloning, expression, and crystallization of recoverin, a calcium sensor in vision. Proc Natl Acad Sci U S A. 1992 Jul 1;89(13):5705–5709. doi: 10.1073/pnas.89.13.5705. [DOI] [PMC free article] [PubMed] [Google Scholar]
- Smith W. C., Milam A. H., Dugger D., Arendt A., Hargrave P. A., Palczewski K. A splice variant of arrestin. Molecular cloning and localization in bovine retina. J Biol Chem. 1994 Jun 3;269(22):15407–15410. [PubMed] [Google Scholar]
- Tsien R., Pozzan T. Measurement of cytosolic free Ca2+ with quin2. Methods Enzymol. 1989;172:230–262. doi: 10.1016/s0076-6879(89)72017-6. [DOI] [PubMed] [Google Scholar]
- Vuong T. M., Chabre M. Deactivation kinetics of the transduction cascade of vision. Proc Natl Acad Sci U S A. 1991 Nov 1;88(21):9813–9817. doi: 10.1073/pnas.88.21.9813. [DOI] [PMC free article] [PubMed] [Google Scholar]
- Wensel T. G., Stryer L. Activation mechanism of retinal rod cyclic GMP phosphodiesterase probed by fluorescein-labeled inhibitory subunit. Biochemistry. 1990 Feb 27;29(8):2155–2161. doi: 10.1021/bi00460a028. [DOI] [PubMed] [Google Scholar]
- Wilden U., Hall S. W., Kühn H. Phosphodiesterase activation by photoexcited rhodopsin is quenched when rhodopsin is phosphorylated and binds the intrinsic 48-kDa protein of rod outer segments. Proc Natl Acad Sci U S A. 1986 Mar;83(5):1174–1178. doi: 10.1073/pnas.83.5.1174. [DOI] [PMC free article] [PubMed] [Google Scholar]
- Zozulya S., Stryer L. Calcium-myristoyl protein switch. Proc Natl Acad Sci U S A. 1992 Dec 1;89(23):11569–11573. doi: 10.1073/pnas.89.23.11569. [DOI] [PMC free article] [PubMed] [Google Scholar]