Abstract
By postulating temperature-dependent equilibria between two or more electron carriers acting as traps for electrons or holes, it is possible to modify the Randall-Wilkins theory of thermoluminescence so as to explain the abnormally large apparent activation energies and apparent frequency factors observed in photosynthetic glow curves when fitted by unmodified Randall-Wilkins theory. The equilibria serve to inhibit the formation of the light-emitting excited state by withholding the needed precursor state. When the inhibition is released at higher temperature by shift of equilibrium with temperature, the rise of the glow peak can be much faster than would result from Arrhenius behavior based on the true activation energy and so appears to correspond to a higher activation energy accompanied by a larger frequency factor. From another viewpoint, the enthalpy changes, ΔH, of the equilibria tend to add to the activation energy. Similarly the entropy changes, ΔS, of the equilibria tend to add to the entropy of activation, giving the large apparent frequency factors. The positive values of ΔS needed would correspond to entropy decreases in the forward early electron transport. A comparison of the glow peaks obtained by different workers is also presented.
Keywords: thermoluminescence, delayed light, reversed electron flow, electron and hole traps
Full text
PDF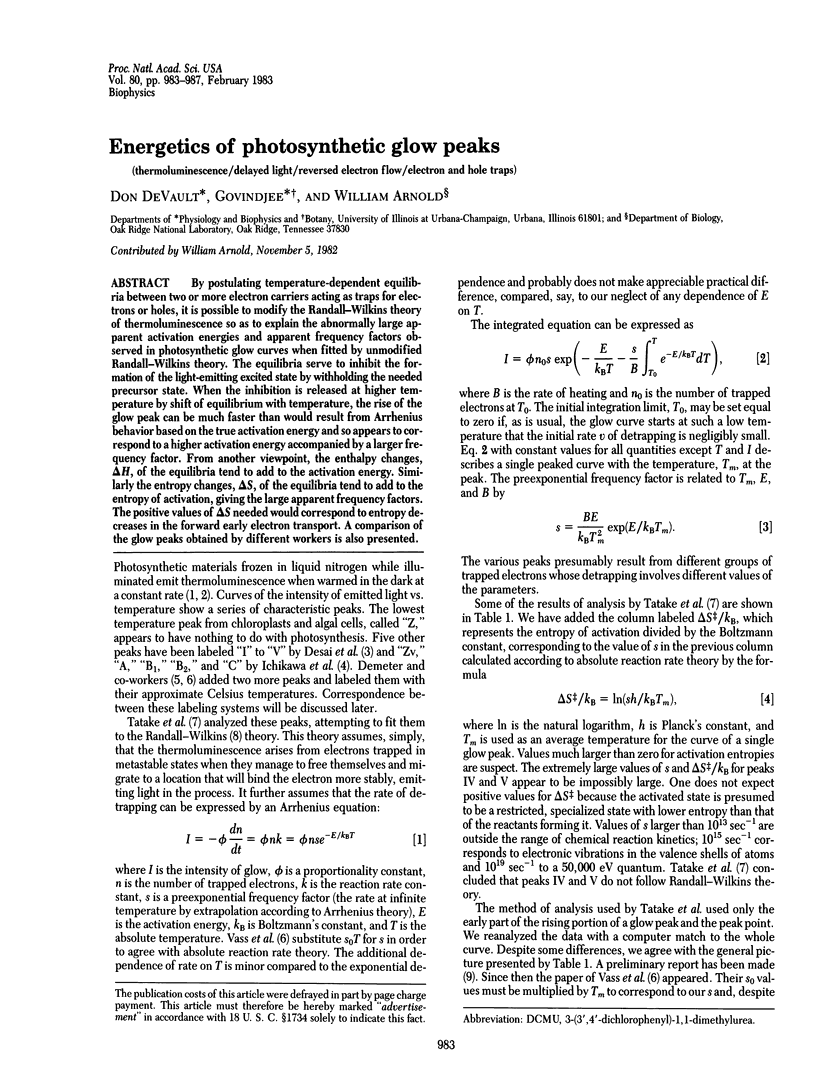
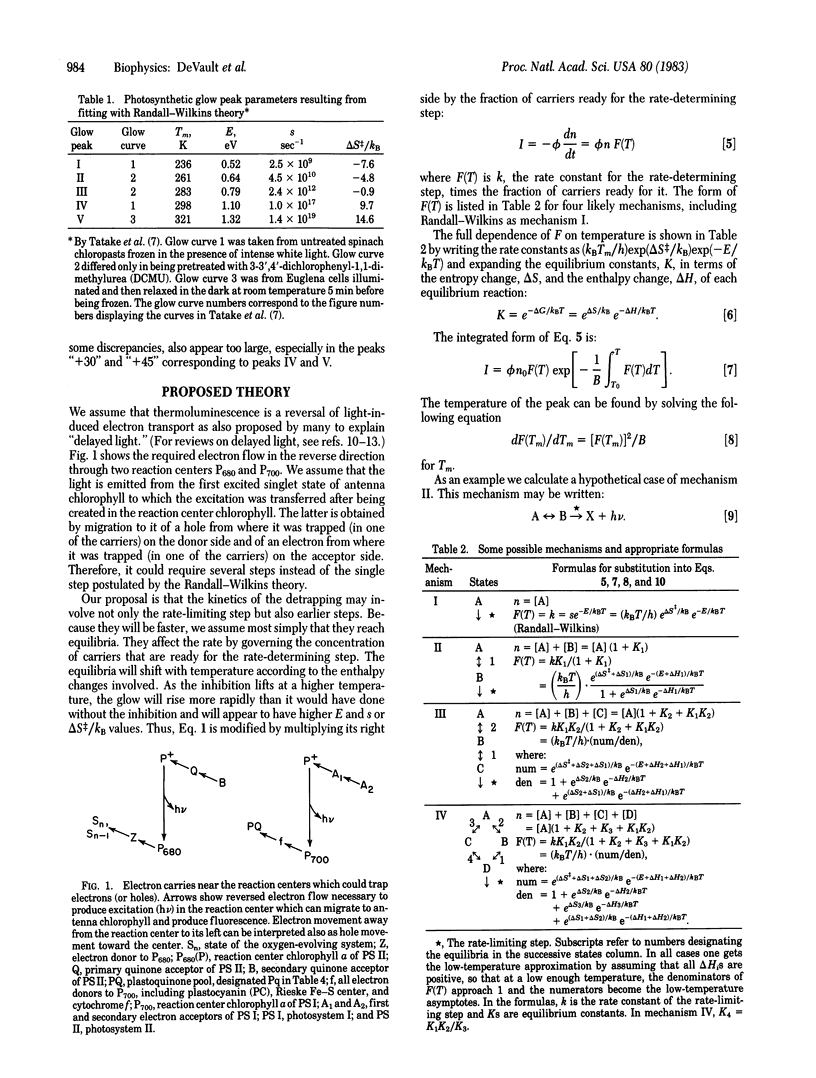
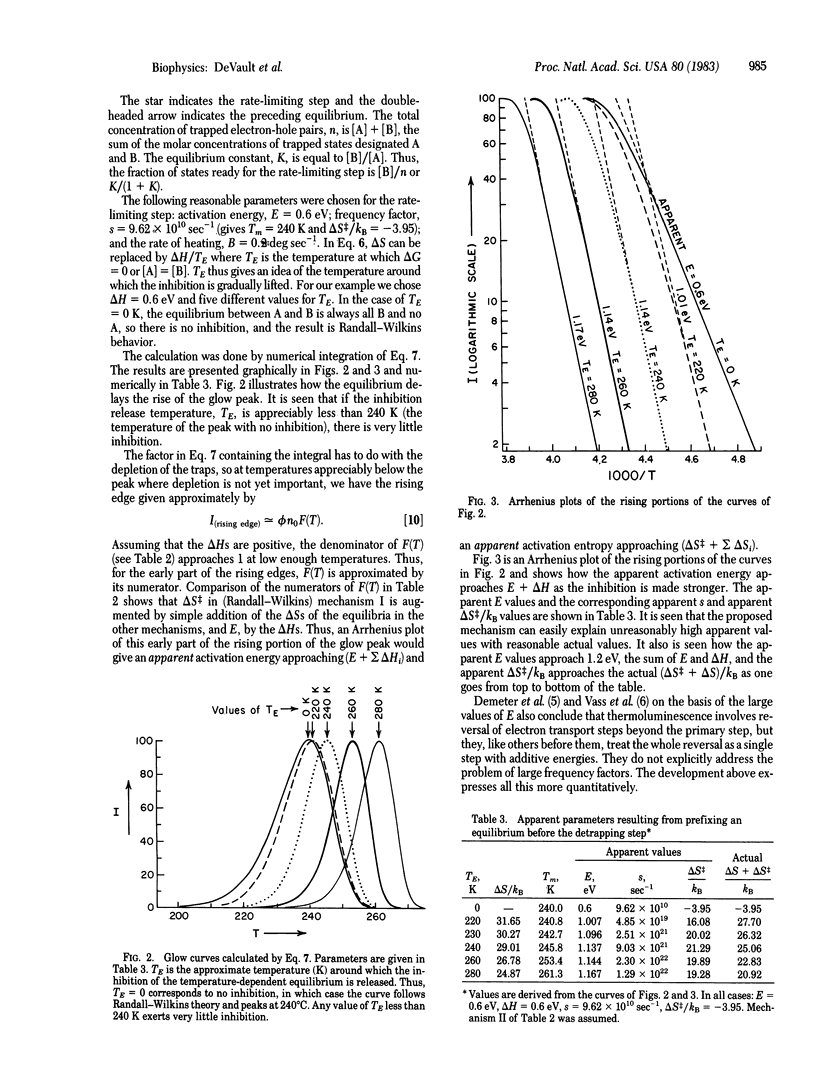
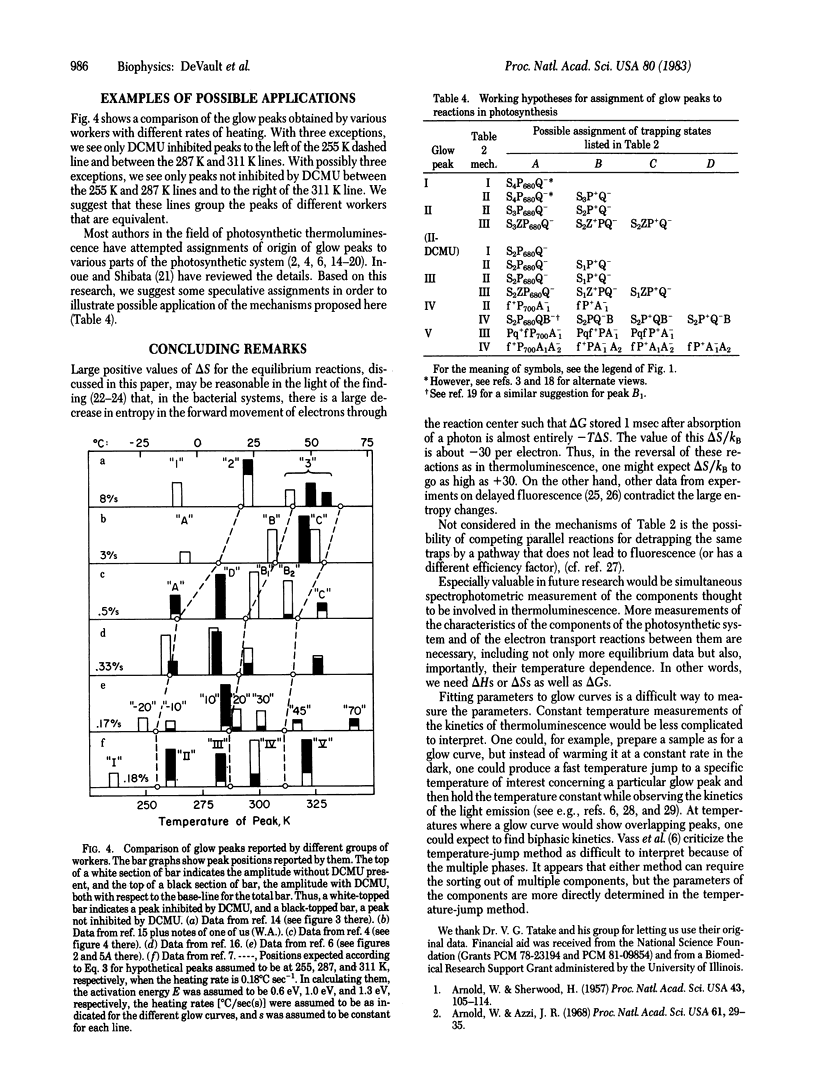
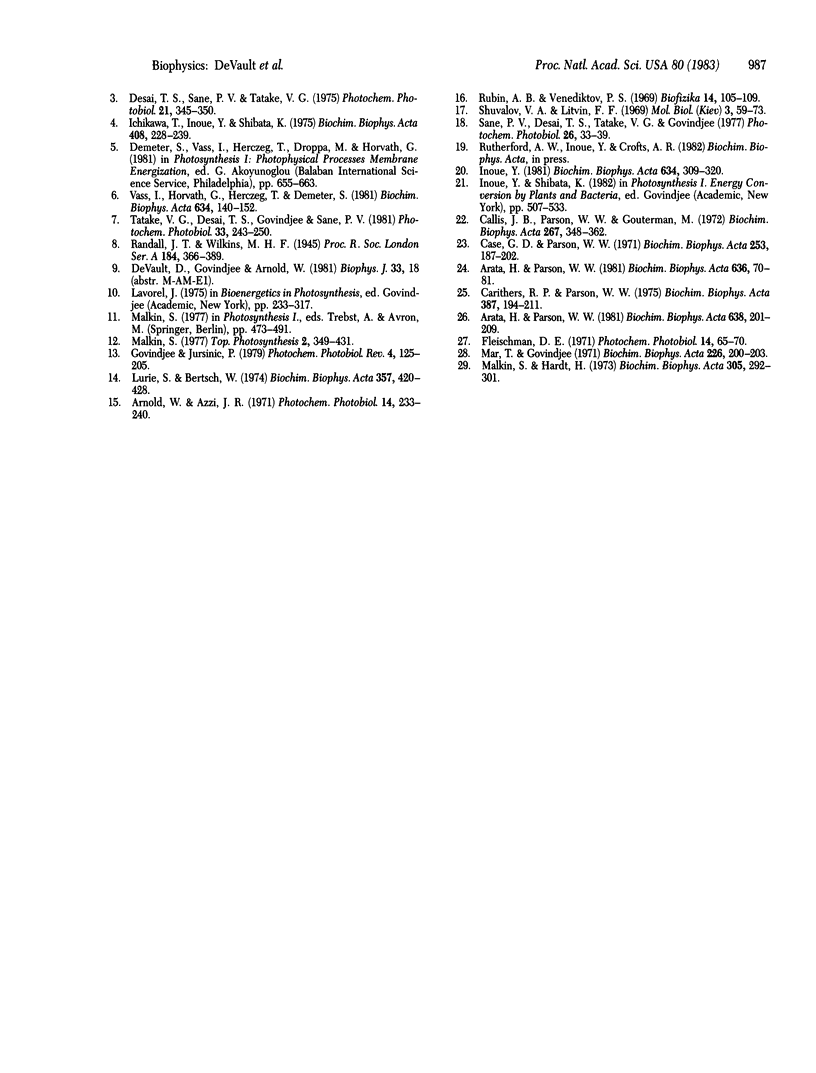
Selected References
These references are in PubMed. This may not be the complete list of references from this article.
- Arata H., Parson W. W. Enthalpy and volume changes accompanying electron transfer from P-870 to quinones in Rhodopseudomonas sphaeroides reaction centers. Biochim Biophys Acta. 1981 Jun 12;636(1):70–81. doi: 10.1016/0005-2728(81)90077-3. [DOI] [PubMed] [Google Scholar]
- Arnold W., Azzi J. R. Chlorophyll energy levels and electron flow in photosynthesis. Proc Natl Acad Sci U S A. 1968 Sep;61(1):29–35. doi: 10.1073/pnas.61.1.29. [DOI] [PMC free article] [PubMed] [Google Scholar]
- Arnold W., Sherwood H. K. ARE CHLOROPLASTS SEMICONDUCTORS? Proc Natl Acad Sci U S A. 1957 Jan 15;43(1):105–114. doi: 10.1073/pnas.43.1.105. [DOI] [PMC free article] [PubMed] [Google Scholar]
- Callis J. B., Parson W. W., Gouterman M. Fast changes of enthalpy and volume on flash excitation of Chromatium chromatophores. Biochim Biophys Acta. 1972 May 25;267(2):348–362. doi: 10.1016/0005-2728(72)90122-3. [DOI] [PubMed] [Google Scholar]
- Carithers R. P., Parson W. W. Delayed fluorescence from Rhodopseudomonas viridis following single flashes. Biochim Biophys Acta. 1975 May 15;387(2):194–211. doi: 10.1016/0005-2728(75)90103-6. [DOI] [PubMed] [Google Scholar]
- Case G. D., Parson W. W. Thermodynamics of the primary and secondary photochemical reactions in Chromatium. Biochim Biophys Acta. 1971 Nov 2;253(1):187–202. doi: 10.1016/0005-2728(71)90244-1. [DOI] [PubMed] [Google Scholar]
- Desai T. S., Sane P. V., Tatake V. G. Thermoluminescence studies on spinach leaves and Euglena. Photochem Photobiol. 1975 May;21(5):345–350. doi: 10.1111/j.1751-1097.1975.tb06682.x. [DOI] [PubMed] [Google Scholar]
- Ichikawa T., Inoue Y., Shibata K. Characteristics of thermoluminescence bands of intact leaves and isolated chloroplasts in relation to the water-splitting activity in photosynthesis. Biochim Biophys Acta. 1975 Dec 11;408(3):228–239. doi: 10.1016/0005-2728(75)90126-7. [DOI] [PubMed] [Google Scholar]
- Inoue Y. Charging of the A band of thermoluminescence, dependent on the S3 state in isolated chloroplasts. Biochim Biophys Acta. 1981 Feb 12;634(2):309–320. doi: 10.1016/0005-2728(81)90149-3. [DOI] [PubMed] [Google Scholar]
- Lurie S., Bertsch W. Thermoluminescence studies on photosynthetic energy conversion. I. Evidence for three types of energy storage by photoreaction II of higher plants. Biochim Biophys Acta. 1974 Sep 20;357(3):420–428. doi: 10.1016/0005-2728(74)90032-2. [DOI] [PubMed] [Google Scholar]
- Malkin S., Hardt H. Kinetic characterization of T-jump thermoluminescence in isolated chloroplasts. Biochim Biophys Acta. 1973 May 30;305(2):292–301. doi: 10.1016/0005-2728(73)90177-1. [DOI] [PubMed] [Google Scholar]
- Mar T., Govindjee Thermoluminescence in spinach chloroplasts and in Chlorella. Biochim Biophys Acta. 1971 Jan 12;226(1):200–203. doi: 10.1016/0005-2728(71)90193-9. [DOI] [PubMed] [Google Scholar]
- Rubin A. B., Venediktov P. S. O zapasanii svetovoi énergii fotosinteziruiushchimi organizmami pri nizkikh temperaturakh. Biofizika. 1969 Jan-Feb;14(1):105–109. [PubMed] [Google Scholar]
- Vass I., Horváth G., Herczeg T., Demeter S. Photosynthetic energy conservation investigated by thermoluminescence. Activation energies and half-lives of thermoluminescence bands of chloroplasts determined by mathematical resolution of glow curves. Biochim Biophys Acta. 1981 Jan 14;634(1):140–152. doi: 10.1016/0005-2728(81)90134-1. [DOI] [PubMed] [Google Scholar]