Abstract
Several mixed-function oxidation systems catalyze the inactivation of Escherichia coli glutamine synthetase. Inactivation involves modification of a single histidine residue in each enzyme subunit and makes the enzyme susceptible to proteolytic degradation. We show here that 10 key enzymes in metabolism are inactivated by a bacterial NADH oxidase and by an oxidase system comprised of NADPH, cytochrome P-450 reductase, and cytochrome P-450 isozyme 2 from rabbit liver microsomes. Most of the inactivatable enzymes require a divalent cation for activity and all but one (enolase) possess a nucleotide binding site. Glutamine synthetase, pyruvate kinase, and phosphoglycerate kinase are protected from inactivation by their substrates; substrate protection of other enzymes was not tested. We propose that inactivation involves mixed-function oxidization system-catalyzed synthesis of H2O2 and reduction of Fe(III) to Fe(II) followed by oxidation of enzyme-bound Fe(II) by H2O2 to generate oxygen radicals that attack a histidine (or other oxidizable amino acid) at the metal binding site of the enzyme. This is supported by the following: (i) most of the inactivation reactions are inhibited by EDTA and by catalase, (ii) both mixed-function oxidation systems reduce Fe(III), and (iii) H2O2 together with Fe(II) catalyzes nonenzymic inactivation of glutamine synthetase. In view of the fact that inactivation of glutamine synthetase makes it susceptible to proteolytic degradation, it is possible that mixed-function oxidation system-catalyzed inactivation of enzymes is a regulatory step in enzyme turn-over. In addition, the implication of oxidative inactivation reactions in ageing is suggested by the fact that many of the enzymes inactivated by mixed-function oxidation systems are known to accumulate as inactive forms during ageing.
Keywords: cytochrome P-450, NADH oxidase, enzymic inactivation
Full text
PDF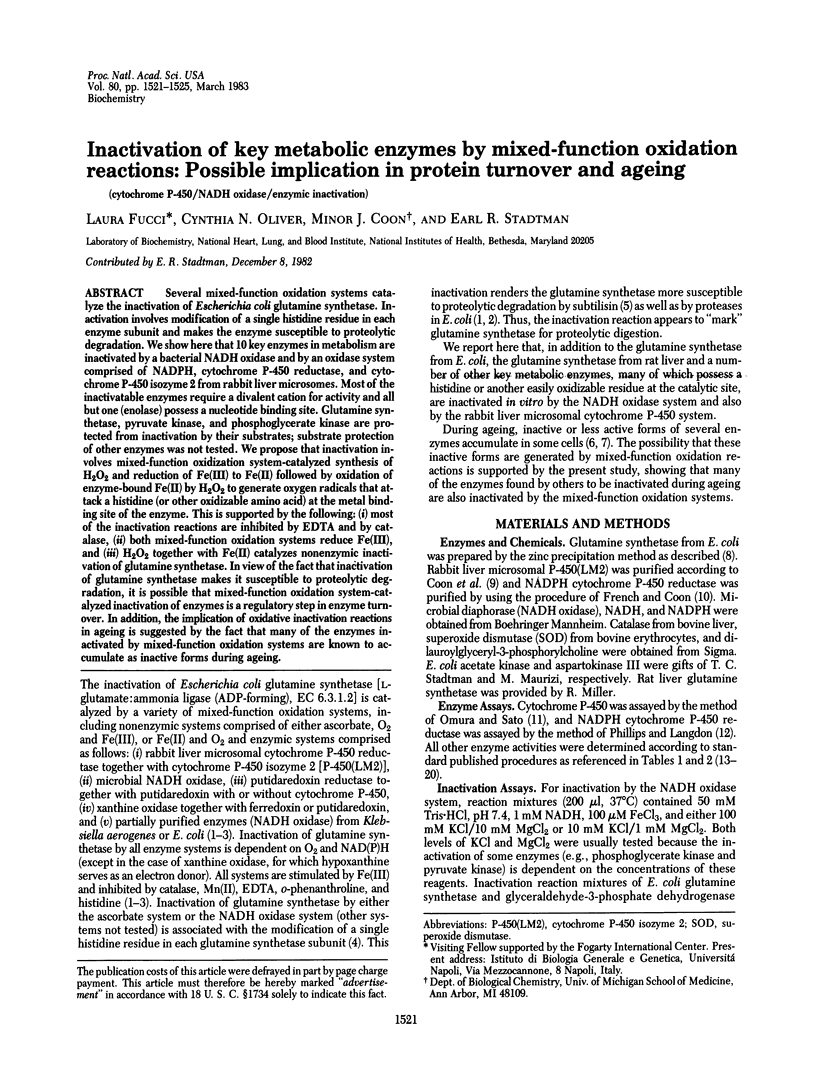
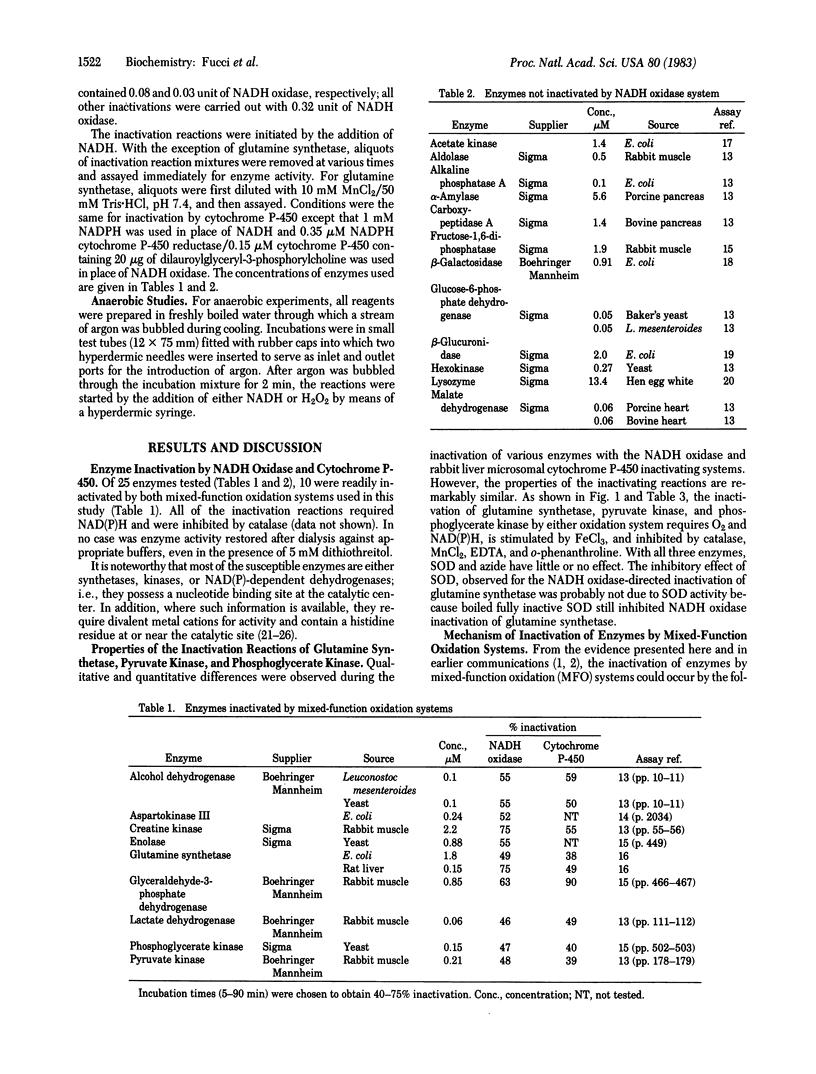
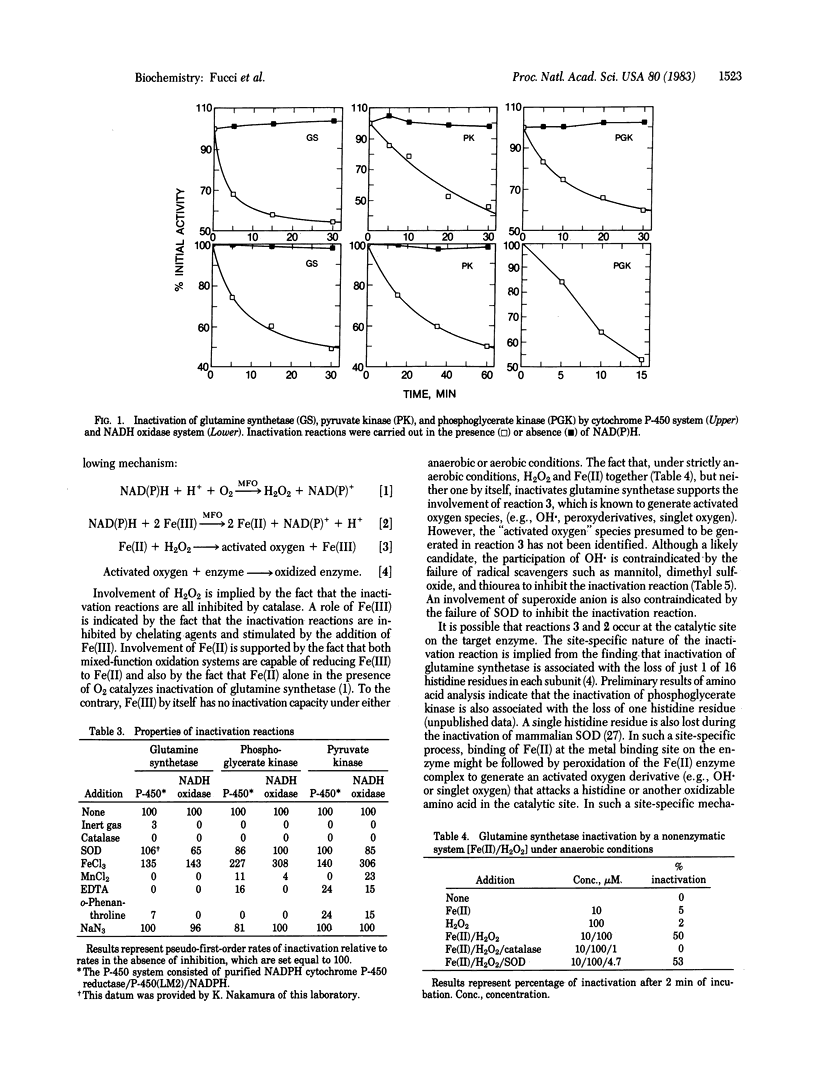
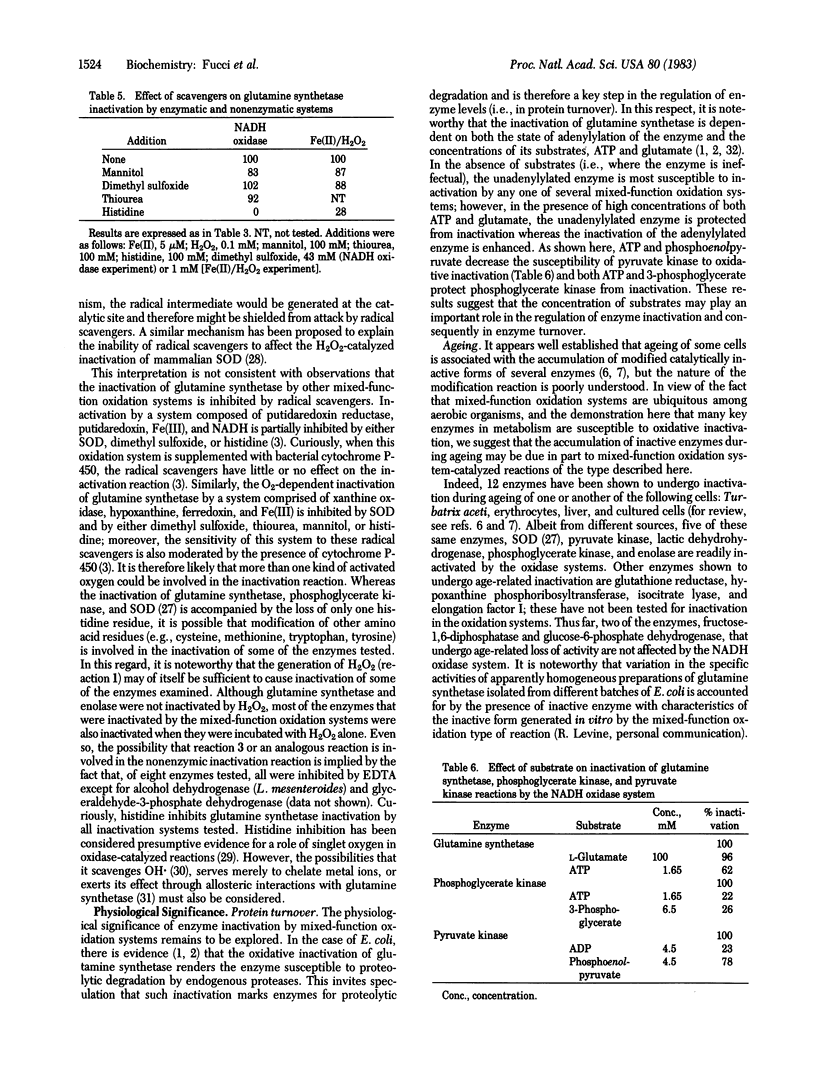
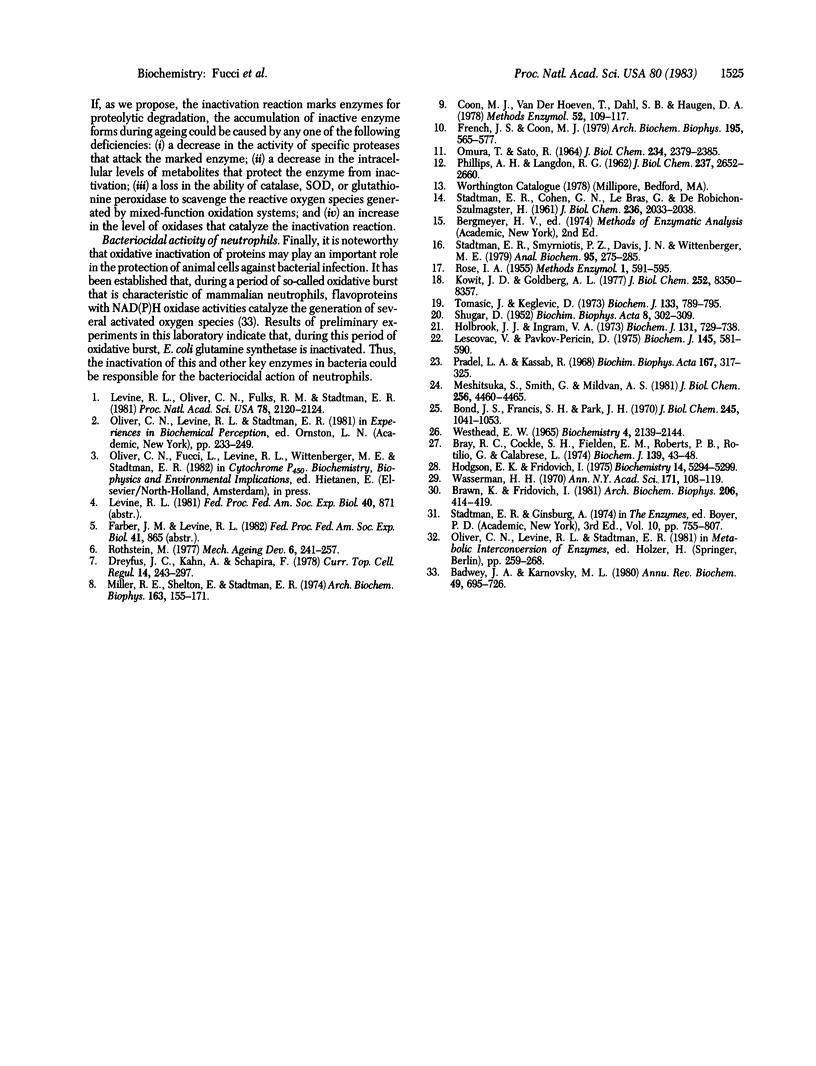
Selected References
These references are in PubMed. This may not be the complete list of references from this article.
- Badwey J. A., Karnovsky M. L. Active oxygen species and the functions of phagocytic leukocytes. Annu Rev Biochem. 1980;49:695–726. doi: 10.1146/annurev.bi.49.070180.003403. [DOI] [PubMed] [Google Scholar]
- Bond J. S., Francis S. H., Park J. H. An essential histidine in the catalytic activities of 3-phosphoglyceraldehyde dehydrogenase. J Biol Chem. 1970 Mar 10;245(5):1041–1053. [PubMed] [Google Scholar]
- Brawn K., Fridovich I. DNA strand scission by enzymically generated oxygen radicals. Arch Biochem Biophys. 1981 Feb;206(2):414–419. doi: 10.1016/0003-9861(81)90108-9. [DOI] [PubMed] [Google Scholar]
- Bray R. C., Cockle S. A., Fielden E. M., Roberts P. B., Rotilio G., Calabrese L. Reduction and inactivation of superoxide dismutase by hydrogen peroxide. Biochem J. 1974 Apr;139(1):43–48. doi: 10.1042/bj1390043. [DOI] [PMC free article] [PubMed] [Google Scholar]
- Coon M. J., van der Hoeven T. A., Dahl S. B., Haugen D. A. Two forms of liver microsomal cytochrome P-450, P-450lm2 and P-450LM4 (rabbit liver). Methods Enzymol. 1978;52:109–117. doi: 10.1016/s0076-6879(78)52012-0. [DOI] [PubMed] [Google Scholar]
- Dreyfus J. C., Kahn A., Schapira F. Posttranslational modifications of enzymes. Curr Top Cell Regul. 1978;14:243–297. doi: 10.1016/b978-0-12-152814-0.50010-1. [DOI] [PubMed] [Google Scholar]
- French J. S., Coon M. J. Properties of NADPH-cytochrome P-450 reductase purified from rabbit liver microsomes. Arch Biochem Biophys. 1979 Jul;195(2):565–577. doi: 10.1016/0003-9861(79)90383-7. [DOI] [PubMed] [Google Scholar]
- Hodgson E. K., Fridovich I. The interaction of bovine erythrocyte superoxide dismutase with hydrogen peroxide: inactivation of the enzyme. Biochemistry. 1975 Dec 2;14(24):5294–5299. doi: 10.1021/bi00695a010. [DOI] [PubMed] [Google Scholar]
- Holbrook J. J., Ingram V. A. Ionic properties of an essential histidine residue in pig heart lactate dehydrogenase. Biochem J. 1973 Apr;131(4):729–738. doi: 10.1042/bj1310729. [DOI] [PMC free article] [PubMed] [Google Scholar]
- Kowit J. D., Goldberg A. L. Intermediate steps in the degradation of a specific abnormal protein in Escherichia coli. J Biol Chem. 1977 Dec 10;252(23):8350–8357. [PubMed] [Google Scholar]
- Leskovac V., Pavkov-Pericin D. Evidence for a histidine and a cysteine residue in the substrate-binding site of yeast alcohol dehydrogenase. Biochem J. 1975 Mar;145(3):581–590. doi: 10.1042/bj1450581. [DOI] [PMC free article] [PubMed] [Google Scholar]
- Levine R. L., Oliver C. N., Fulks R. M., Stadtman E. R. Turnover of bacterial glutamine synthetase: oxidative inactivation precedes proteolysis. Proc Natl Acad Sci U S A. 1981 Apr;78(4):2120–2124. doi: 10.1073/pnas.78.4.2120. [DOI] [PMC free article] [PubMed] [Google Scholar]
- Meshitsuka S., Smith G. M., Mildvan A. S. Proton NMR studies of the histidine residues of rabbit muscle pyruvate kinase and of its phosphoenolpyruvate complex. J Biol Chem. 1981 May 10;256(9):4460–4465. [PubMed] [Google Scholar]
- Miller R. E., Shelton E., Stadtman E. R. Zinc-induced paracrystalline aggregation of glutamine synthetase. Arch Biochem Biophys. 1974 Jul;163(1):155–171. doi: 10.1016/0003-9861(74)90465-2. [DOI] [PubMed] [Google Scholar]
- OMURA T., SATO R. THE CARBON MONOXIDE-BINDING PIGMENT OF LIVER MICROSOMES. II. SOLUBILIZATION, PURIFICATION, AND PROPERTIES. J Biol Chem. 1964 Jul;239:2379–2385. [PubMed] [Google Scholar]
- PHILLIPS A. H., LANGDON R. G. Hepatic triphosphopyridine nucleotide-cytochrome c reductase: isolation, characterization, and kinetic studies. J Biol Chem. 1962 Aug;237:2652–2660. [PubMed] [Google Scholar]
- Pradel L. A., Kassab R. Site actif des ATP: guanidine phosphotransférases. II. Mise en évidence de résidus histidine essentiels au moyen du pyrocarbonate d'éthyle. Biochim Biophys Acta. 1968 Oct 8;167(2):317–325. doi: 10.1016/0005-2744(68)90211-8. [DOI] [PubMed] [Google Scholar]
- Rothstein M. Recent developments in the age-related alteration of enzymes: a review. Mech Ageing Dev. 1977 Jul-Aug;6(4):241–257. doi: 10.1016/0047-6374(77)90025-2. [DOI] [PubMed] [Google Scholar]
- SHUGAR D. The measurement of lysozyme activity and the ultra-violet inactivation of lysozyme. Biochim Biophys Acta. 1952 Mar;8(3):302–309. doi: 10.1016/0006-3002(52)90045-0. [DOI] [PubMed] [Google Scholar]
- Stadtman E. R., Smyrniotis P. Z., Davis J. N., Wittenberger M. E. Enzymic procedures for determining the average state of adenylylation of Escherichia coli glutamine synthetase. Anal Biochem. 1979 May;95(1):275–285. doi: 10.1016/0003-2697(79)90217-3. [DOI] [PubMed] [Google Scholar]
- Tomasić J., Keglević D. The kinetics of hydrolysis of synthetic glucuronic esters and glucuronic ethers by bovine liver and Escherichia coli beta-glucuronidase. Biochem J. 1973 Aug;133(4):789–795. doi: 10.1042/bj1330789. [DOI] [PMC free article] [PubMed] [Google Scholar]